Plant Disease: Autophagy under attack
The Irish potato famine was responsible for more than one million deaths and the emigration of one million people from Europe in the 1840s (Andrivon, 1996). Today, the microbe that caused the famine, an oomycete called Phytophthora infestans, continues to cause serious outbreaks of disease in potato crops. Traditional control measures, such as fungicides and breeding for resistance, often have only marginal success in combating the disease, especially when the climate favors the growth and development of P. infestans (Fry and Goodwin, 1997). Now, in eLife, Sophien Kamoun, Tolga Bozkurt and colleagues – including Yasin Dagdas and Khaoula Belhaj as joint first authors – reveal how one of the proteins produced by P. infestans manipulates host plant cells to weaken their defenses (Dagdas et al., 2016).
It is well established that plant pathogens secrete proteins and small molecules – collectively known as effectors – that can interfere with plant defenses and make it easier for pathogens to infect and spread (Djamei et al., 2011; de Wit et al., 2009; Rovenich et al., 2014; Gawehns et al., 2014). However, as part of an ongoing arms race between plants and pathogens, some effectors are recognized by proteins in the host plant, which triggers immune responses that act to contain the infection. Relatively little is known about how effectors interfere with plant defenses. In particular, the identities of the plant molecules that are targeted by the effectors, and details of how the effectors are transported into plant cells, remain unclear.
The success of P. infestans as a pathogen is largely due to its ability to secrete hundreds of different effectors. Now, Dagdas, Belhaj et al. – who are based at the Sainsbury Laboratory, the John Innes Centre and Imperial College – report how they carried out a screen for plant molecules that interact with effectors from P. infestans (Dagdas et al., 2016). The experiments were carried out in the leaves of tobacco, which is a commonly used plant model, and show that an effector called PexRD54 targets a process called autophagy in plant cells.
Autophagy is a complex “self-eating” process that occurs when plant and other eukaryotic cells experience certain stresses – for example, due to a shortage of nutrients or a change in environmental conditions. During autophagy, cell material is broken down to supply the building blocks needed to maintain essential processes (Li and Vierstra, 2009). More recently, autophagy has been implicated in a variety of other situations, including restricting the growth and spread of invading microbes. A growing body of evidence suggests that autophagy plays a dual role both in promoting the survival of cells and in triggering cell death.
During autophagy, cell materials are sequestered by structures called autophagosomes and then delivered to acidic cell compartments where the material is degraded and recycled. In addition to supporting the bulk degradation of cell materials, it was recently shown that autophagy allows the selective removal of cellular components that are damaged or no longer needed. In selective autophagy, the sequestered material is loaded into autophagosomes by specific interactions between receptor proteins and specific autophagy proteins, such as the ATG8 proteins (Stolz et al., 2014, Lamb et al., 2013).
Dagdas, Belhaj et al. found that PexRD54 interferes with the activity of a potato cargo receptor called Joka2. PexRD54 out-competes Joka2 to bind to an ATG8 protein and stimulate the formation of an autophagosome in the plant cell (Figure 1). In doing so, the oomycete cleverly reduces the loading of specific types of cargo into autophagosomes and thus limits the plant defense response.
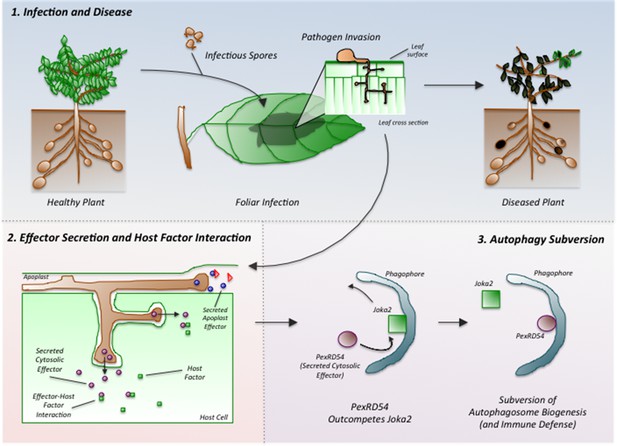
Phytophthora infestans interferes with the immune responses of potato plants.
Spores of P. infestans land on the leaves of potato plants and germinate (top middle). The growing fungus enters the leaves and spreads around the plant, leading to disease (top right). Proteins called effectors are released from the pathogen and some are taken into the cells of the host plant (bottom left). These effectors (purple ovals) interact with host factors (green squares) to promote the progression of the disease. Dagdas, Belhaj et al. found that a P. infestans effector called PexRD54 (purple oval; bottom right) out-competes a plant cargo receptor known as Joka2 (green square) on the surface of a membrane structure called a phagophore, which eventually becomes an autophagosome. In this way, PexRD54 prevents the loading of cargo proteins into autophagosomes and inhibits plant defenses.
The reported observations expand upon studies of mammalian pathogens that also harbor effectors that interfere with autophagy (Table 1). Taken together, this work provides a template for future investigations into the ways in which effectors subvert host plant defenses. However, a number of interesting questions remain unanswered. For example, how do cargo receptors work? How are they regulated? What is the nature of the cargo in the autophagosomes and how does it regulate immune responses? In addition, our understanding of the mechanisms that control selective autophagy remain incomplete. How is the selectivity regulated, and what other cell mechanisms might be subverted by effectors? Phytophthora diseases can have devastating effects, but as this study illustrates, they can also illuminate and advance our understanding of fundamental cellular processes.
Mammalian pathogens that express proteins that interfere with host autophagosome biogenesis or function.
Domain | Pathogen | Host | Effector | Activity | Refs |
---|---|---|---|---|---|
Virus | HIV virus | human | Nef1 | Inhibits host autophagy | Campbell et al., 2015 |
CMV virus | human | Trs1 | Inhibits host autophagy | Chaumorcel et al., 2012 | |
Dengue virus | mammal | NS4A | Upregulation of autophagy | McLean et al., 2011 | |
Bacteria | Legionella | mammal | RavZ | Cleaves an Atg8 protein from pre-autophagosomes | Choy et al., 2012; Horenkamp et al., 2015 |
Coxiella | mammal | Cig2 | Disrupts interactions between acidic compartments and host autophagosomes | Newton et al., 2014 | |
Salmonella | mammal | SseL | Inhibits selective autophagy of cytosolic aggregates | Mesquita et al., 2012 | |
Anaplasma phagocytophilum | mammal | Ats-1 | Hijacks a pathway that activates autophagy to promote its growth inside cells | Niu et al., 2012 | |
Vibrio parahemolyticus | mammal | VopQ | Creates pores in acidic compartments in host cells | Sreelatha et al., 2013 | |
Eukaryote | Phytophthora | plant | PexRD54 | Inappropriately activates the formation of autophagosomes | Dagdas et al., 2016 |
References
-
The human cytomegalovirus protein TRS1 inhibits autophagy via its interaction with beclin 1Journal of Virology 86:2571–2584.https://doi.org/10.1128/JVI.05746-11
-
Fungal effector proteins: past, present and futureMolecular Plant Pathology 10:735–747.https://doi.org/10.1111/j.1364-3703.2009.00591.x
-
The Fusarium oxysporum effector Six6 contributes to virulence and suppresses i-2-mediated cell deathMolecular Plant-Microbe Interactions 27:336–348.https://doi.org/10.1094/MPMI-11-13-0330-R
-
The autophagosome: origins unknown, biogenesis complexNature Reviews Molecular Cell Biology 14:759–774.https://doi.org/10.1038/nrm3696
-
Autophagy: a multifaceted intracellular system for bulk and selective recyclingTrends in Plant Science 17:526–537.https://doi.org/10.1016/j.tplants.2012.05.006
-
Flavivirus NS4A-induced autophagy protects cells against death and enhances virus replicationJournal of Biological Chemistry 286:22147–22159.https://doi.org/10.1074/jbc.M110.192500
-
Autophagosomes induced by a bacterial beclin 1 binding protein facilitate obligatory intracellular infectionProceedings of the National Academy of Sciences 109:20800–20807.https://doi.org/10.1073/pnas.1218674109
-
Filamentous pathogen effector functions: of pathogens, hosts and microbiomesCurrent Opinion in Plant Biology 20:96–103.https://doi.org/10.1016/j.pbi.2014.05.001
-
Late blight of potato and tomatoThe Plant Health Instructor.https://doi.org/10.1094/PHI-I-2000-0724-01
-
Cargo recognition and trafficking in selective autophagyNature Cell Biology 16:495–501.https://doi.org/10.1038/ncb2979
Article and author information
Author details
Publication history
Copyright
© 2016, de Figueiredo et al.
This article is distributed under the terms of the Creative Commons Attribution License, which permits unrestricted use and redistribution provided that the original author and source are credited.
Metrics
-
- 2,719
- views
-
- 481
- downloads
-
- 3
- citations
Views, downloads and citations are aggregated across all versions of this paper published by eLife.
Download links
Downloads (link to download the article as PDF)
Open citations (links to open the citations from this article in various online reference manager services)
Cite this article (links to download the citations from this article in formats compatible with various reference manager tools)
Further reading
-
- Ecology
- Microbiology and Infectious Disease
Interspecies interactions involving direct competition via bacteriocin production play a vital role in shaping ecological dynamics within microbial ecosystems. For instance, the ribosomally produced siderophore bacteriocins, known as class IIb microcins, affect the colonization of host-associated pathogenic Enterobacteriaceae species. Notably, to date, only five of these antimicrobials have been identified, all derived from specific Escherichia coli and Klebsiella pneumoniae strains. We hypothesized that class IIb microcin production extends beyond these specific compounds and organisms. With a customized informatics-driven approach, screening bacterial genomes in public databases with BLAST and manual curation, we have discovered 12 previously unknown class IIb microcins in seven additional Enterobacteriaceae species, encompassing phytopathogens and environmental isolates. We introduce three novel clades of microcins (MccW, MccX, and MccZ), while also identifying eight new variants of the five known class IIb microcins. To validate their antimicrobial potential, we heterologously expressed these microcins in E. coli and demonstrated efficacy against a variety of bacterial isolates, including plant pathogens from the genera Brenneria, Gibbsiella, and Rahnella. Two newly discovered microcins exhibit activity against Gram-negative ESKAPE pathogens, i.e., Acinetobacter baumannii or Pseudomonas aeruginosa, providing the first evidence that class IIb microcins can target bacteria outside of the Enterobacteriaceae family. This study underscores that class IIb microcin genes are more prevalent in the microbial world than previously recognized and that synthetic hybrid microcins can be a viable tool to target clinically relevant drug-resistant pathogens. Our findings hold significant promise for the development of innovative engineered live biotherapeutic products tailored to combat these resilient bacteria.
-
- Biochemistry and Chemical Biology
- Microbiology and Infectious Disease
Malaria parasites have evolved unusual metabolic adaptations that specialize them for growth within heme-rich human erythrocytes. During blood-stage infection, Plasmodium falciparum parasites internalize and digest abundant host hemoglobin within the digestive vacuole. This massive catabolic process generates copious free heme, most of which is biomineralized into inert hemozoin. Parasites also express a divergent heme oxygenase (HO)-like protein (PfHO) that lacks key active-site residues and has lost canonical HO activity. The cellular role of this unusual protein that underpins its retention by parasites has been unknown. To unravel PfHO function, we first determined a 2.8 Å-resolution X-ray structure that revealed a highly α-helical fold indicative of distant HO homology. Localization studies unveiled PfHO targeting to the apicoplast organelle, where it is imported and undergoes N-terminal processing but retains most of the electropositive transit peptide. We observed that conditional knockdown of PfHO was lethal to parasites, which died from defective apicoplast biogenesis and impaired isoprenoid-precursor synthesis. Complementation and molecular-interaction studies revealed an essential role for the electropositive N-terminus of PfHO, which selectively associates with the apicoplast genome and enzymes involved in nucleic acid metabolism and gene expression. PfHO knockdown resulted in a specific deficiency in levels of apicoplast-encoded RNA but not DNA. These studies reveal an essential function for PfHO in apicoplast maintenance and suggest that Plasmodium repurposed the conserved HO scaffold from its canonical heme-degrading function in the ancestral chloroplast to fulfill a critical adaptive role in organelle gene expression.