Cell Communication: Studying gap junctions with PARIS
For our bodies to work properly, cells need to communicate with each other. One way to do so is via gap junctions, which are connections between cells that allow small signaling molecules or electrical signals to pass between them. Cells coupled together by gap junctions often synchronize their activity (Bennett and Zukin, 2004).
Neuromodulators and the activity of neurons can affect gap junction coupling between neurons, which, in turn, can influence the pattern of activity in neurons (Lane et al., 2018; Haas et al., 2011). Gap junctions have also been implicated in a variety of diseases (Nielsen et al., 2012). So far, it has been difficult to identify their precise roles in health and disease, as present methods to map or monitor gap junction coupling are often invasive and damaging, or unable to measure a specific cell type in enough detail.
Now, in eLife, Yulong Li and colleagues at Peking University – including Ling Wu as first author – report that they have developed a new method called PARIS to map and measure gap junctions (Wu et al., 2019). PARIS is a genetically encoded system, consisting of an optically controlled ‘actuator’ in one cell (the actuator cell) and a fluorescent sensor in another (the receiver cell). When stimulated with light, the actuator generates an electrochemical gradient with the help of a light-gated proton pump. The activated pump then transports hydrogen ions out of the cell. If the cells are coupled to each other, the departing ions change the pH in the receiver cell, causing a measurable increase in fluorescence (Figure 1A,B).
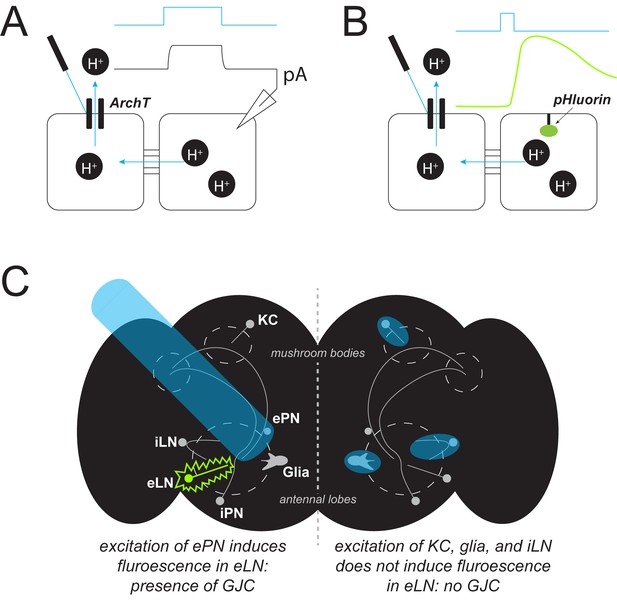
Detecting gap junction coupling.
(A) A new method called PARIS can be used to study gap junctions between cells (black squares). It is a genetically encoded system in which one cell (the ‘actuator’) contains a light-gated proton pump (ArchT). If the actuator cell is coupled to another (‘receiver’) cell, shining light onto the pump (blue trace) causes protons (H+, black circles) to flow, generating a measurable electrical current (black trace, pA). (B) In an alternate detection method, a fluorescent sensor (pHluorin, green oval) can be placed in the receiver cell. Once activated, the pump transports protons out of the actuator cell; if the cells are coupled, this induces a change in the pH of the receiver cell that increases the fluorescence of the sensor (green trace). (C) PARIS can be used to determine whether gap junction coupling (GJC) exists between different cell types by using light (blue beam) to stimulate a particular type of neuron and checking for fluorescence (green) from another type of neuron. For example, Wu et al. showed that excitatory projection neurons (ePN) in the Drosophila brain (black shape, left hemisphere) form gap junctions with excitatory local neurons (eLN) but not with other nearby cell types (KC, glia, iLN).
The pH gradient generated by PARIS can be detected in multiple receiver cells, even when they are not coupled directly to the actuator. Changes to the pH are known to affect the coupling and the strength of gap junctions (Nielsen et al., 2012). However, Wu et al. show that a slight pH increase of 0.1 is sufficient to detect gap junction coupling and therefore does not appear to disrupt the activity of a cell, even in repeated trials.
Furthermore, the change of fluorescence is noticeable within seconds and provides a consistent signal over repeated measurements that is strong enough to be tracked over hours. Moreover, since the technique is fully genetically encoded, there is no need to use dyes or to deliver compounds directly into the cell. Instead, Wu et al. have shown that PARIS can be delivered into the cell by various molecular gene transfer techniques, including virus-based transfer methods, allowing it to be applied to a wide range of organisms.
Given these attributes, PARIS can be used in a variety of fields where it promises to accelerate and expand research. For example, it could be used to study abnormal gap junction coupling associated with certain conditions, or to monitor drugs expected to alter coupling (Haas et al., 2011; Lane et al., 2018; Lane et al., 2016). It may even be used to study signaling within a cell, as it has been shown that certain intracellular enzymes can alter gap junction coupling too.
The ability of PARIS to assess gap junction coupling between individual cell types, especially in the brain, is particularly attractive. Wu et al. were able to confirm that in the olfactory system of fruit flies, a type of neuron known as an excitatory projection neuron, forms gap junctions with excitatory local neurons, but not with other nearby cell types (Figure 1C; Huang et al., 2010; Yaksi and Wilson, 2010).
PARIS also can be used to gain insight into where cells connect with each other. For example, the researchers revealed that excitatory and inhibitory projection neurons are coupled at their dendrites rather than at their axons. This degree of resolution is invaluable for studying nervous systems, and is difficult to achieve by other means.
Projects to map the brain connectivity in humans and other species based on chemical synaptic connectivity alone will be incomplete because they fail to describe alternate pathways information may use to travel, such as gap junctions (Marder et al., 2017). With future improvements in the sensitivity of the fluorescent receiver to an even smaller pH change, PARIS has the potential to be a valuable tool to study cell communication and its role in many different diseases.
References
-
Complicating connectomes: electrical coupling creates parallel pathways and degenerate circuit mechanismsDevelopmental Neurobiology 77:597–609.https://doi.org/10.1002/dneu.22410
Article and author information
Author details
Publication history
Copyright
© 2019, Kick and Schulz
This article is distributed under the terms of the Creative Commons Attribution License, which permits unrestricted use and redistribution provided that the original author and source are credited.
Metrics
-
- 1,339
- views
-
- 144
- downloads
-
- 1
- citations
Views, downloads and citations are aggregated across all versions of this paper published by eLife.
Download links
Downloads (link to download the article as PDF)
Open citations (links to open the citations from this article in various online reference manager services)
Cite this article (links to download the citations from this article in formats compatible with various reference manager tools)
Further reading
-
- Neuroscience
Concurrent verbal working memory task can eliminate the color-word Stroop effect. Previous research, based on specific and limited resources, suggested that the disappearance of the conflict effect was due to the memory information preempting the resources for distractors. However, it remains unclear which particular stage of Stroop conflict processing is influenced by working memory loads. In this study, electroencephalography (EEG) recordings with event-related potential (ERP) analyses, time-frequency analyses, multivariate pattern analyses (MVPAs), and representational similarity analyses (RSAs) were applied to provide an in-depth investigation of the aforementioned issue. Subjects were required to complete the single task (the classical manual color-word Stroop task) and the dual task (the Sternberg working memory task combined with the Stroop task), respectively. Behaviorally, the results indicated that the Stroop effect was eliminated in the dual-task condition. The EEG results showed that the concurrent working memory task did not modulate the P1, N450, and alpha bands. However, it modulated the sustained potential (SP), late theta (740–820 ms), and beta (920–1040 ms) power, showing no difference between congruent and incongruent trials in the dual-task condition but significant difference in the single-task condition. Importantly, the RSA results revealed that the neural activation pattern of the late theta was similar to the response interaction pattern. Together, these findings implied that the concurrent working memory task eliminated the Stroop effect through disrupting stimulus-response mapping.
-
- Neuroscience
Two-photon (2P) fluorescence imaging through gradient index (GRIN) lens-based endoscopes is fundamental to investigate the functional properties of neural populations in deep brain circuits. However, GRIN lenses have intrinsic optical aberrations, which severely degrade their imaging performance. GRIN aberrations decrease the signal-to-noise ratio (SNR) and spatial resolution of fluorescence signals, especially in lateral portions of the field-of-view (FOV), leading to restricted FOV and smaller number of recorded neurons. This is especially relevant for GRIN lenses of several millimeters in length, which are needed to reach the deeper regions of the rodent brain. We have previously demonstrated a novel method to enlarge the FOV and improve the spatial resolution of 2P microendoscopes based on GRIN lenses of length <4.1 mm (Antonini et al., 2020). However, previously developed microendoscopes were too short to reach the most ventral regions of the mouse brain. In this study, we combined optical simulations with fabrication of aspherical polymer microlenses through three-dimensional (3D) microprinting to correct for optical aberrations in long (length >6 mm) GRIN lens-based microendoscopes (diameter, 500 µm). Long corrected microendoscopes had improved spatial resolution, enabling imaging in significantly enlarged FOVs. Moreover, using synthetic calcium data we showed that aberration correction enabled detection of cells with higher SNR of fluorescent signals and decreased cross-contamination between neurons. Finally, we applied long corrected microendoscopes to perform large-scale and high-precision recordings of calcium signals in populations of neurons in the olfactory cortex, a brain region laying approximately 5 mm from the brain surface, of awake head-fixed mice. Long corrected microendoscopes are powerful new tools enabling population imaging with unprecedented large FOV and high spatial resolution in the most ventral regions of the mouse brain.