Bacterial Warfare: Toxins, mutations and adaptations
Bacterial communities are often comprised of numerous different species which either co-exist in harmony or compete with each other for resources. To gain an upper hand on the competition, some bacteria have developed a form of needle-like machinery called a type VI secretion system (or T6SS for short) that injects toxic proteins directly into their rivals (Basler et al., 2012; Hood et al., 2010; Klein et al., 2020; Russell et al., 2011). It is generally thought that the toxins secreted by T6SS decapacitate the target organism by impairing important processes such as cell wall synthesis, ATP production and DNA replication (Ahmad et al., 2019; Jurėnas and Journet, 2020; Whitney et al., 2015). While these toxins are clearly involved in anti-bacterial warfare, it is unclear whether T6SS can also facilitate symbiotic relationships within bacterial communities.
Recently, a collaboration between groups led by Joseph Mougous (University of Washington) and David Liu (Harvard University and the Broad Institute) discovered that the T6SS of Burkholderia cenocepacia secretes a toxin called DddA that catalyzes the removal of an amino group from cytosine, converting it to uracil (Mok et al., 2020). In most cells, these enzymes – known as cytosine deaminases – are important for maintaining the levels of nucleotide precursors in cells (Neuhard, 1968). While most cytosine deaminases catalyze this reaction in single-stranded DNA, DddA is the first enzyme found to convert cytosine to uracil in double-stranded DNA. However, uracil is normally only found in RNA, where it pairs with another nucleotide base called adenosine: in DNA, adenosine pairs with thymine, whereas cytosine pairs with guanine in both DNA and RNA (Figure 1).
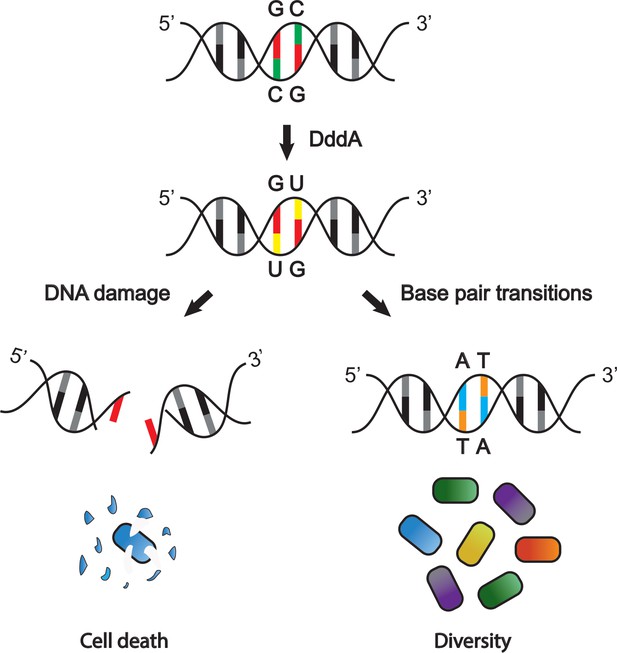
The DddA toxin from B. cenocepacia affects other bacterial species in different ways.
DddA is a toxin that removes an amino group from cytosine (C; green), converting it into uracil (U; yellow) in chromosomal DNA (top). In some bacterial species uracil is then removed by the DNA repair machinery (left), which can lead to double-stranded DNA breaks and ultimately cell death. In bacteria resistant to the toxic effects of DddA, DNA breaks do not occur (right): instead, uracil is converted into thymine (T; orange), which causes guanine (G; red) to convert to adenosine (A; blue). This results in genetic variation within the targeted population, further diversifying the community of bacteria.
Now, in eLife, Mougous and colleagues – including Marcos de Moraes as first author – report that as well as destroying bacteria, DddA also provides a selective advantage for some bacteria in the community (de Moraes et al., 2021). The team (who are based at the University of Washington) found that when the bacterium B. cenocepacia injects DddA, uracil accumulates in the DNA of the targeted bacteria. Since uracil is normally only found in RNA, its presence triggers a repair mechanism that attempts to remove it from the DNA. However, this repair mechanism can lead to a break in one strand of the DNA, and if there are too many breaks in close proximity, they can lead to double-stranded breaks which stop DNA replication and result in bacterial cell death (Figure 1; D'souza and Harrison, 2003; Wallace, 2014). Indeed, de Moraes et al. found that the DddA delivered by B. cenocepacia suppresses the viability of several other bacterial species, including Pseudomona aeruginosa and Burkholderia thailandensis.
Although DddA causes double-stranded DNA breaks and cell death in most bacterial species, some disease-causing bacteria – including Eschericia coli and Salmonella enterica – are able to resist its detrimental effects. To better understand this observation, de Moraes et al. examined the long-term effects of DddA on these bacteria. They found that rather than excising the uracil that had replaced cytosine, the DNA replication machinery in the infected bacteria converts the uracil into a different nucleotide, thymine. As thymine pairs with adenosine in DNA, C-G pairs throughout the genome get replaced with T-A pairs (Figure 1).
An unanticipated consequence of this exchange was that the bacteria not killed by DddA acquired resistance to the antibiotic rifampicin. Further experiments revealed that other deaminase toxins similar to DddA were also able to introduce mutations in single-stranded DNA, suggesting this may be a widespread mechanism within bacterial populations (de Moraes et al., 2021).
It is generally thought that DNA mutations that arise during natural selection are caused by errors during chromosome replication or by exogenous factors such as chemicals and ionizing stress (Schroeder et al., 2018). Some bacteria rapidly adapt by importing fragments of foreign DNA from the environment and integrating them into their genome. This mechanism has been proposed to increase the genetic variation of populations, which provides a selective advantage to bacteria living in challenging environments or competing with other species (Dubnau and Blokesch, 2019; Mell and Redfield, 2014). The findings of de Moreas et al. suggest a similar mechanism in which the mutations produced by deaminase toxins help to diversify the population, creating new variants that can rapidly adapt to environmental changes.
There are several exciting avenues of research resulting from these findings. First, it is unclear why some bacteria are susceptible to DddA intoxication, whereas others are resistant. Defining these resistance mechanisms could help reveal the biological scenarios in which T6SS helps communities of bacteria adapt to their environment. Second, it will be interesting to determine how DddA toxins alter the composition of bacterial communities: DddA intoxication could eliminate competing species, while the genetic variations induced in resistant species may help enhance the long-term fitness of the community. Lastly, new insights into these topics may increase our understanding of human diseases where pathogenic bacteria come into contact with communities of microbes in the body, such as inflammatory bowel disease and cystic fibrosis, which could ultimately lead to better treatments (Buffie and Pamer, 2013; Spiewak et al., 2019).
References
-
Microbiota-mediated colonization resistance against intestinal pathogensNature Reviews Immunology 13:790–801.https://doi.org/10.1038/nri3535
-
Repair of clustered uracil DNA damages in Escherichia coliNucleic Acids Research 31:4573–4581.https://doi.org/10.1093/nar/gkg493
-
Mechanisms of DNA uptake by naturally competent bacteriaAnnual Review of Genetics 53:217–237.https://doi.org/10.1146/annurev-genet-112618-043641
-
Activity, delivery, and diversity of type VI secretion effectorsMolecular Microbiology 575:14648.https://doi.org/10.1111/mmi.14648
-
Contact-dependent interbacterial antagonism mediated by protein secretion machinesTrends in Microbiology 28:387–400.https://doi.org/10.1016/j.tim.2020.01.003
-
Natural competence and the evolution of DNA uptake specificityJournal of Bacteriology 196:1471–1483.https://doi.org/10.1128/JB.01293-13
-
Sources of spontaneous mutagenesis in bacteriaCritical Reviews in Biochemistry and Molecular Biology 53:29–48.https://doi.org/10.1080/10409238.2017.1394262
Article and author information
Author details
Publication history
Copyright
© 2021, De Jong and Alto
This article is distributed under the terms of the Creative Commons Attribution License, which permits unrestricted use and redistribution provided that the original author and source are credited.
Metrics
-
- 1,786
- views
-
- 164
- downloads
-
- 2
- citations
Views, downloads and citations are aggregated across all versions of this paper published by eLife.
Download links
Downloads (link to download the article as PDF)
Open citations (links to open the citations from this article in various online reference manager services)
Cite this article (links to download the citations from this article in formats compatible with various reference manager tools)
Further reading
-
- Evolutionary Biology
- Genetics and Genomics
It is well established that several Homo sapiens populations experienced admixture with extinct human species during their evolutionary history. Sometimes, such a gene flow could have played a role in modulating their capability to cope with a variety of selective pressures, thus resulting in archaic adaptive introgression events. A paradigmatic example of this evolutionary mechanism is offered by the EPAS1 gene, whose most frequent haplotype in Himalayan highlanders was proved to reduce their susceptibility to chronic mountain sickness and to be introduced in the gene pool of their ancestors by admixture with Denisovans. In this study, we aimed at further expanding the investigation of the impact of archaic introgression on more complex adaptive responses to hypobaric hypoxia evolved by populations of Tibetan/Sherpa ancestry, which have been plausibly mediated by soft selective sweeps and/or polygenic adaptations rather than by hard selective sweeps. For this purpose, we used a combination of composite-likelihood and gene network-based methods to detect adaptive loci in introgressed chromosomal segments from Tibetan WGS data and to shortlist those presenting Denisovan-like derived alleles that participate to the same functional pathways and are absent in populations of African ancestry, which are supposed to do not have experienced Denisovan admixture. According to this approach, we identified multiple genes putatively involved in archaic introgression events and that, especially as regards TBC1D1, RASGRF2, PRKAG2, and KRAS, have plausibly contributed to shape the adaptive modulation of angiogenesis and of certain cardiovascular traits in high-altitude Himalayan peoples. These findings provided unprecedented evidence about the complexity of the adaptive phenotype evolved by these human groups to cope with challenges imposed by hypobaric hypoxia, offering new insights into the tangled interplay of genetic determinants that mediates the physiological adjustments crucial for human adaptation to the high-altitude environment.
-
- Cancer Biology
- Genetics and Genomics
A new approach helps examine the proportion of cancerous and healthy stem cells in patients with chronic myeloid leukemia and how this influences treatment outcomes.