Membrane Fusion: Molecular machinery turns full circle
Many biological processes require two membranes to fuse together, such as the release of neurotransmitters from synaptic vesicles in neurons and the fusion of vacuoles in yeast. Scientists have been trying to fully understand what happens during membrane fusion for over three decades and this has led to many twists and turns, like in a mystery movie.
A protein called NSF (short for N-ethylmaleimide sensitive factor) was one of the first to be associated with membrane fusion, and it was postulated that NSF (which is the mammalian homologue of the yeast protein Sec18) was part of a 'fusion machine' made of multiple subunits (Malhotra et al., 1988). It was later found that NSF binds to sites in the membrane with the help of proteins named SNAPs (short for soluble NSF attachment proteins), which are the mammalian homologues of the yeast protein Sec17 (Whiteheart et al., 1992).
Further research led to the discovery that three proteins that had previously been implicated in neurotransmitter release were in fact SNAP receptors (SNAREs) and formed a large membrane-anchored assembly with NSF and SNAP called the 20S complex (Söllner et al., 1993b). It turned out that the three SNAREs could actually form a highly stable complex by themselves, and that this complex is disassembled after binding to SNAPs and NSF through the ATPase activity of NSF (Söllner et al., 1993a).
Two of the neuronal SNAREs that form the complex are anchored to the plasma membrane and the other to the synaptic vesicle. The SNAREs contain one or two strands of amino acids that are called SNARE motifs, which adopt helical structures as they join together to form a complex (Sutton et al., 1998; Figure 1A). The realization that the SNARE motifs bind to one another in a parallel fashion led to the idea that ‘zippering’ of the SNARE motifs, from the N-terminus to the C-terminus, draws the two membranes close together, forcing them to fuse (Hanson et al., 1997; Figure 1A).
This model was bolstered by experiments suggesting that the neuronal SNAREs can fuse model membranes called liposomes (Weber et al., 1998). Moreover, fusion in most intracellular membrane compartments was found to require similar SNARE complexes. These observations led to the textbook notion that SNARE complexes constitute the universal machine that causes membrane fusion, while NSF/Sec18 and SNAPs/Sec17 are responsible for disassembling the complex so the SNAREs can be re-used. Now, in eLife, Bill Wickner and co-workers from Dartmouth College and Stanford University – including Hongki Song and Thomas Torng as joint first authors – report new findings which suggest that this model needs to be revised (Song et al., 2021).
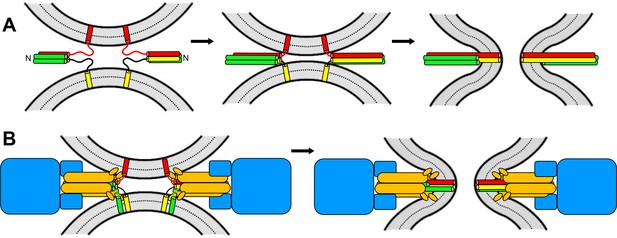
Models of membrane fusion.
(A) Textbook model illustrated with the three neuronal SNARES: two of these (red and yellow) have one SNARE motif each and are attached to opposing membranes (shown in grey) via a transmembrane region; the third SNARE contains two SNARE motifs (green). These motifs bind together like a zipper which runs from the N-terminus to the C-terminus; this zippering process results in the formation of a four-helix bundle that pulls the two membranes together, forcing them to fuse (right). (B) In the model proposed by Song et al., membrane fusion relies on Sec17 (orange), Sec18 (blue) and four SNARES (one in yellow; one in red; two in green) that also form a four-helix bundle. Fusion is induced by the action of the SNAREs, as in A, and by the Sec17 N-terminal hydrophobic loops (small orange ellipses) perturbing the lipid bilayer of the membrane. Some of the SNAREs have N-terminal domains that are not shown for simplicity. The arrangement of the proteins is based on the structure of the neuronal 20S complex (Zhao et al., 2015).
Image credit: Josep Rizo.
Previous studies from the Wickner lab using liposomes that mimic the membranes of yeast vacuoles showed that a protein complex called HOPS helps tether liposomes together and assemble the SNARE complex (Baker et al., 2015; Mima et al., 2008). These studies also showed that the yeast proteins Sec17 and Sec18 synergize with HOPS and the SNAREs to induce fusion of liposomes. Moreover, in a remarkable twist in the plot, another group showed that Sec17 could rescue liposome fusion that was arrested because 22 amino acid residues were removed from the C-terminus of a SNARE motif (Schwartz and Merz, 2009). The removal of the residues destabilizes the SNARE complex, and hence hinders its ability to pull the two membranes together. The fact that Sec17 rescued liposome fusion under these circumstances suggests that it may have an important role in membrane fusion, beyond its involvement in the disassembly of SNARE complexes. However, it is possible that Sec17 merely helped the compromised SNARE complex to zipper fully.
Investigating this further, Song et al. revealed that Sec17 rescues liposome fusion when the C-termini of two SNARE motifs are truncated so that the zippering process is doubly hindered. Further work showed that this recovery depends on a hydrophobic N-terminal loop that Sec17 inserts into membranes, and that fusion is strongly enhanced by Sec18. Amazingly, Sec17 and Sec18 can restore fusion even when the C-termini of three SNARE motifs are impaired, showing that the SNARE complex does not need to be fully zippered in order for membranes to fuse. These findings indicate that the hydrophobic loop of Sec17 can induce fusion as long as the two membranes are brought into close enough proximity by the SNAREs.
Song et al. also found that Sec18 relies on ATP to enhance liposome fusion but without hydrolyzing ATP. This suggests that when Sec18 is bound to ATP, it joins together with Sec17 and the SNAREs to form an assembly that resembles the 20S complex, and that this most likely constitutes the true ‘fusion machine’ (Figure 1B). It is tempting to speculate that nature created this machine so that ATPase activity is slow before the membranes start to fuse; however, once fusion is complete, ATP hydrolysis speeds up to disassemble the 20S complex and recycle the SNAREs.
It now seems likely that 20S complexes mediate most types of intracellular fusion, but there may be exceptions. Indeed, a recent study showed that a mammalian SNAP stopped neuronal SNARE complexes from completing membrane fusion, suggesting that this machinery may control the fusion of synaptic vesicles in other ways (Stepien et al., 2019). It appears that the future may hold more twists and turns for this story.
References
-
Capture and release of partially zipped trans-SNARE complexes on intact organellesJournal of Cell Biology 185:535–549.https://doi.org/10.1083/jcb.200811082
-
Soluble N-ethylmaleimide-sensitive fusion attachment proteins (SNAPs) bind to a multi-SNAP receptor complex in Golgi membranesJournal of Biological Chemistry 267:12239–12243.https://doi.org/10.1016/S0021-9258(19)49830-X
Article and author information
Author details
Publication history
Copyright
© 2021, Rizo et al.
This article is distributed under the terms of the Creative Commons Attribution License, which permits unrestricted use and redistribution provided that the original author and source are credited.
Metrics
-
- 1,203
- views
-
- 99
- downloads
-
- 2
- citations
Views, downloads and citations are aggregated across all versions of this paper published by eLife.
Download links
Downloads (link to download the article as PDF)
Open citations (links to open the citations from this article in various online reference manager services)
Cite this article (links to download the citations from this article in formats compatible with various reference manager tools)
Further reading
-
- Biochemistry and Chemical Biology
- Genetics and Genomics
Yerba mate (YM, Ilex paraguariensis) is an economically important crop marketed for the elaboration of mate, the third-most widely consumed caffeine-containing infusion worldwide. Here, we report the first genome assembly of this species, which has a total length of 1.06 Gb and contains 53,390 protein-coding genes. Comparative analyses revealed that the large YM genome size is partly due to a whole-genome duplication (Ip-α) during the early evolutionary history of Ilex, in addition to the hexaploidization event (γ) shared by core eudicots. Characterization of the genome allowed us to clone the genes encoding methyltransferase enzymes that catalyse multiple reactions required for caffeine production. To our surprise, this species has converged upon a different biochemical pathway compared to that of coffee and tea. In order to gain insight into the structural basis for the convergent enzyme activities, we obtained a crystal structure for the terminal enzyme in the pathway that forms caffeine. The structure reveals that convergent solutions have evolved for substrate positioning because different amino acid residues facilitate a different substrate orientation such that efficient methylation occurs in the independently evolved enzymes in YM and coffee. While our results show phylogenomic constraint limits the genes coopted for convergence of caffeine biosynthesis, the X-ray diffraction data suggest structural constraints are minimal for the convergent evolution of individual reactions.
-
- Biochemistry and Chemical Biology
- Structural Biology and Molecular Biophysics
The SARS-CoV-2 main protease (Mpro or Nsp5) is critical for production of viral proteins during infection and, like many viral proteases, also targets host proteins to subvert their cellular functions. Here, we show that the human tRNA methyltransferase TRMT1 is recognized and cleaved by SARS-CoV-2 Mpro. TRMT1 installs the N2,N2-dimethylguanosine (m2,2G) modification on mammalian tRNAs, which promotes cellular protein synthesis and redox homeostasis. We find that Mpro can cleave endogenous TRMT1 in human cell lysate, resulting in removal of the TRMT1 zinc finger domain. Evolutionary analysis shows the TRMT1 cleavage site is highly conserved in mammals, except in Muroidea, where TRMT1 is likely resistant to cleavage. TRMT1 proteolysis results in reduced tRNA binding and elimination of tRNA methyltransferase activity. We also determined the structure of an Mpro-TRMT1 peptide complex that shows how TRMT1 engages the Mpro active site in an uncommon substrate binding conformation. Finally, enzymology and molecular dynamics simulations indicate that kinetic discrimination occurs during a later step of Mpro-mediated proteolysis following substrate binding. Together, these data provide new insights into substrate recognition by SARS-CoV-2 Mpro that could help guide future antiviral therapeutic development and show how proteolysis of TRMT1 during SARS-CoV-2 infection impairs both TRMT1 tRNA binding and tRNA modification activity to disrupt host translation and potentially impact COVID-19 pathogenesis or phenotypes.