Breast Cancer: How cell crowding causes cancer cells to spread
Cells must constantly respond and adapt to changes in their environment, such as temperature fluctuations and mechanical stress. These responses include cell growth and migration (Rice et al., 2020; Di et al., 2023). Cancer cells must also respond to their environment, in particular to the high density of nearby cells caused by their rapid proliferation. The mechanical pressure caused by this “cell crowding” can make the cancer cells more likely to spread and invade surrounding tissues, but we do not fully understand the mechanisms driving these processes. Understanding how cancer cells respond to cell crowding, and identifying the signals that make them more invasive in these conditions, is important for developing effective cancer treatments.
Ductal carcinoma in situ (or DCIS for short) is a non-invasive form of breast cancer that is confined to the milk ducts. Although it does not spread to surrounding tissue, if left untreated, it can transition into an invasive form of cancer that does spread (Pinder and Ellis, 2003). Now, in eLife, Inhee Chung and colleagues from George Washington University and Thomas Jefferson High School for Science and Technology – including Xiangning Bu as first author – report new insights into how cell crowding drives migration in certain types of DCIS cells (Bu et al., 2024).
First, Bu et al. demonstrated that DCIS cells categorized as more likely to be aggressive based on their appearance (also known as high-grade cells) become more invasive in crowded conditions. These invasive cells had a smaller volume than healthy cells or lower-grade DCIS cells. Cell volume changes are known to be closely linked to cell stiffness, which affects a cell’s ability to push through and invade surrounding tissues (Guo et al., 2017; Xie et al., 2024).
To investigate the mechanism behind this increased invasiveness, Bu et al. used a technique known as mass spectrometry to examine various proteins in the cells. In high-grade DCIS cells, they observed that cell crowding led to an increase in the number of various proteins on the plasma membrane of the cells. In particular, there was a striking 153-fold increase for a calcium ion channel called TRPV4. Transport proteins, such as ion channels and aquaporins, play a crucial role in the regulation of cell volume by creating osmotic gradients that cause water to move in and out of cells (Jentsch, 2016). TRPV4 is known to be activated by osmotic stress, mechanical stress, heat and certain chemical stimuli (Heller and O’Neil, 2007). This movement of TRPV4 and other ion-channel proteins from the cytoplasm to the plasma membrane, as well as reduced cell volume, could also be triggered by increasing osmotic pressure outside the cell, even in the absence of cell crowding.
Based on the finding that TRPV4 relocation was associated with cell crowding, Bu et al. next studied calcium levels in high-grade DCIS cells exposed to different cell crowding densities. This showed that cell crowding decreases intracellular calcium. Bu et al. next blocked TRPV4 function pharmacologically, finding that this mimicked the effects of cell crowding, promoting cell shrinkage and invasion. On the other hand, activating TRPV4 reversed these changes by increasing cell volume.
These results suggest that cell crowding acts as a mechanical cue that inhibits the action of TRPV4, reducing the transport of calcium ions into the cell and thus causing water to move out of the cell, leading to volume loss. This inhibition causes TRPV4 to move from the cytoplasm to the plasma membrane, to ensure that it is primed to become activated by mechanical stress later (Figure 1). Silencing the TRPV4 gene impaired the ability of cancer cells to respond to mechanical cues, leading to less calcium depletion, smaller volume changes, and reduced mobility.
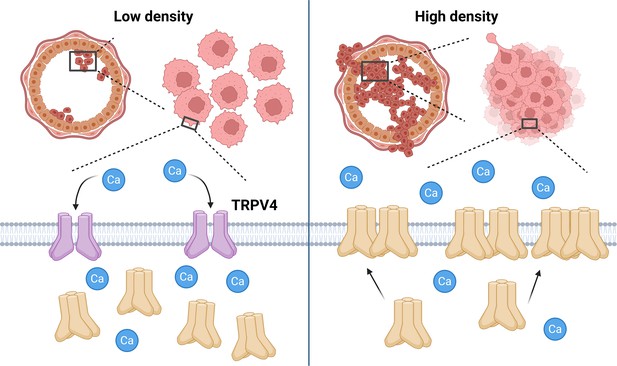
The influence of cell crowding on TRPV4 calcium ion channels.
When the density of breast cancer cells (pink) in the milk duct (large circular structure) is low (left), the majority of TRPV4 calcium ion channels are found in the cytoplasm of the cells in an inactive state (cream shapes), with a minority at the plasma membrane (gray) in an active state (purple shapes). The active channels facilitate the movement of calcium ions (Ca; blue circles) into the cell. However, when the density of cells is high (right), the mechanical stress caused by cell crowding inhibits TRPV4 activity and causes TRPV4 to move from the cytoplasm to the plasma membrane (cream shapes). This inhibition of TRPV4 decreases intracellular calcium levels, causing cell volume to shrink, which is a process associated with enhanced cellular invasiveness. The subsequent increase in TRPV4 at the plasma membrane also primes the cell for later activation of TRPV4 to compensate for the loss of calcium ions. These findings suggest that TRPV4 redistribution plays a critical role in the response of cancer cells to mechanical stress, potentially contributing to tumor progression. Created with BioRender.com.
Finally, analyzing patient-derived breast cancer tissue confirmed that TRPV4 is primarily localized to the plasma membrane in high-grade DCIS, but not in lower-grade DCIS or less aggressive cases. These findings suggest that TRPV4 plays a crucial role in influencing crowded cancer cells to become more invasive, as well as serving as a biomarker for identifying high-grade DCIS cells. This could open possibilities for targeting mechanosensitive pathways in cancer treatments and developing diagnostic tools for high-risk DCIS and other cancers.
While the findings of Bu et al. highlight a role for TRPV4 in invasiveness, other ion channels may also be involved (Ranade et al., 2015). Mass spectrometry screening revealed increases in two channel proteins – SCN11 and KCNN4 – caused by cell crowding. Additionally, an ion channel known as PIEZO1 was also found to relocate to the cell membrane under cell crowding conditions. Future research could explore how these channels interact and contribute to cancer adaptation. Other interesting questions include: why do different cell types respond differently to cell crowding, and what molecular mechanisms govern TRPV4 relocation? Addressing these questions could provide deeper insights into how ion channels contribute to cancer progression and help identify potential therapeutic targets.
References
-
Cellular mechanotransduction in health and diseases: from molecular mechanism to therapeutic targetsSignal Transduction and Targeted Therapy 8:282.https://doi.org/10.1038/s41392-023-01501-9
-
BookMolecular mechanisms of TRPV4 gatingIn: Liedtke WB, editors. TRP Ion Channel Function in Sensory Transduction and Cellular Signaling Cascades. Boca Raton: CRC Press. pp. 113–124.https://doi.org/10.1201/9781420005844
-
VRACs and other ion channels and transporters in the regulation of cell volume and beyondNature Reviews Molecular Cell Biology 17:293–307.https://doi.org/10.1038/nrm.2016.29
-
Unveiling the intricate connection: cell volume as a key regulator of mechanotransductionAnnual Review of Biophysics 53:299–317.https://doi.org/10.1146/annurev-biophys-030822-035656
Article and author information
Author details
Publication history
Copyright
© 2025, Hua and Jiang
This article is distributed under the terms of the Creative Commons Attribution License, which permits unrestricted use and redistribution provided that the original author and source are credited.
Metrics
-
- 251
- views
-
- 51
- downloads
-
- 0
- citations
Views, downloads and citations are aggregated across all versions of this paper published by eLife.
Download links
Downloads (link to download the article as PDF)
Open citations (links to open the citations from this article in various online reference manager services)
Cite this article (links to download the citations from this article in formats compatible with various reference manager tools)
Further reading
-
- Cancer Biology
- Cell Biology
Testicular microcalcifications consist of hydroxyapatite and have been associated with an increased risk of testicular germ cell tumors (TGCTs) but are also found in benign cases such as loss-of-function variants in the phosphate transporter SLC34A2. Here, we show that fibroblast growth factor 23 (FGF23), a regulator of phosphate homeostasis, is expressed in testicular germ cell neoplasia in situ (GCNIS), embryonal carcinoma (EC), and human embryonic stem cells. FGF23 is not glycosylated in TGCTs and therefore cleaved into a C-terminal fragment which competitively antagonizes full-length FGF23. Here, Fgf23 knockout mice presented with marked calcifications in the epididymis, spermatogenic arrest, and focally germ cells expressing the osteoblast marker Osteocalcin (gene name: Bglap, protein name). Moreover, the frequent testicular microcalcifications in mice with no functional androgen receptor and lack of circulating gonadotropins are associated with lower Slc34a2 and higher Bglap/Slc34a1 (protein name: NPT2a) expression compared with wild-type mice. In accordance, human testicular specimens with microcalcifications also have lower SLC34A2 and a subpopulation of germ cells express phosphate transporter NPT2a, Osteocalcin, and RUNX2 highlighting aberrant local phosphate handling and expression of bone-specific proteins. Mineral disturbance in vitro using calcium or phosphate treatment induced deposition of calcium phosphate in a spermatogonial cell line and this effect was fully rescued by the mineralization inhibitor pyrophosphate. In conclusion, testicular microcalcifications arise secondary to local alterations in mineral homeostasis, which in combination with impaired Sertoli cell function and reduced levels of mineralization inhibitors due to high alkaline phosphatase activity in GCNIS and TGCTs facilitate osteogenic-like differentiation of testicular cells and deposition of hydroxyapatite.
-
- Cancer Biology
TAK1 is a serine/threonine protein kinase that is a key regulator in a wide variety of cellular processes. However, the functions and mechanisms involved in cancer metastasis are still not well understood. Here, we found that TAK1 knockdown promoted esophageal squamous cancer carcinoma (ESCC) migration and invasion, whereas TAK1 overexpression resulted in the opposite outcome. These in vitro findings were recapitulated in vivo in a xenograft metastatic mouse model. Mechanistically, co-immunoprecipitation and mass spectrometry demonstrated that TAK1 interacted with phospholipase C epsilon 1 (PLCE1) and phosphorylated PLCE1 at serine 1060 (S1060). Functional studies revealed that phosphorylation at S1060 in PLCE1 resulted in decreased enzyme activity, leading to the repression of phosphatidylinositol 4,5-bisphosphate (PIP2) hydrolysis. As a result, the degradation products of PIP2 including diacylglycerol (DAG) and inositol IP3 were reduced, which thereby suppressed signal transduction in the axis of PKC/GSK-3β/β-Catenin. Consequently, expression of cancer metastasis-related genes was impeded by TAK1. Overall, our data indicate that TAK1 plays a negative role in ESCC metastasis, which depends on the TAK1-induced phosphorylation of PLCE1 at S1060.