Pathogen Evolution: Exploring accidental virulence
Not all microbes, such as bacteria and fungi, display virulent properties that allow them to infect host cells and cause disease. While some disease-causing pathogens need a host in order to replicate and survive, some can also live freely in the environment. However, how these pathogens go from innocuously living outside of a host to being able to cause disease within one remains an open question.
One leading theory suggests that these are ‘accidental’ pathogens. According to this ‘accidental virulence theory’, environmental factors cause microbes to gain properties that allow them to survive without a host. While these microbes can exist independently, the adaptations can also make them better equipped to survive, replicate and cause disease if they happen to encounter a potential host (Casadevall and Pirofski, 2007; Figure 1A). For example, microbes that have adapted to living in acidic environments, may also be able to survive in the acidic conditions of animal immune cells, enabling them to infect a host (Hackam et al., 1999). This theory challenges the idea that microbes only acquire the ability to causes disease through exposure and adapting to conditions in a specific host. Now, in eLife, Helen Murphy and colleagues from the College of William and Mary and Vanderbilt University – including Luke Ekdahl and Juliana Salcedo as joint first authors – report evidence supporting the accidental virulence theory using the traditionally non-pathogenic fungus Saccharomyces cerevisiae (Figure 1B; Ekdahl et al., 2023).
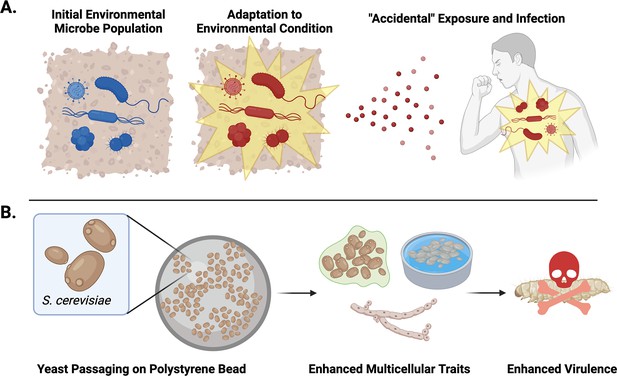
Testing the accidental virulence hypothesis.
(A) Environmental microbes (blue) adapt to new conditions and stressors in their surroundings. If these adapted microbes (red) come into contact with a potential host, their new adaptations may better equip them to infect and cause disease, despite not having encountered the host previously. (B) Ekdahl et al. demonstrated that repeatedly selecting Saccharomyces cerevisiae yeast that could grow on polystyrene beads led to the yeast evolving more multicellular traits that resulted in them growing more regularly into a biofilm, flor, or forming pseudohyphae. The enhanced frequency of multicellular traits made the yeast better at infecting wax moth larvae.
Image credit: Figure created using elements from Biorender.com.
Despite S. cerevisiae, which is commonly referred to as baker’s yeast, being considered non-pathogenic, there are some instances in which it can infect humans (Enache-Angoulvant and Hennequin, 2005). This makes it an interesting model for studying how adaptations not related directly to virulence may coincidentally allow the yeast to infect a host. Additionally, there are already many readily available tools for teasing apart the mechanism of evolution and increased pathogenic potential in this model organism.
Ekdahl et al. investigated how S. cerevisiae characteristics were affected by exposure to plastic – a common component of modern environments due to microplastic pollution. The authors grew two separate S. cerevisiae strains on polystyrene beads for 350–400 generations. Throughout this period, only cells that adhered to the beads were transferred to each new bead culture. By doing this, the team created a selection pressure that was specific to the yeast’s interaction with the plastic and not related to how it interacts with the immune system of a potential host. To ensure genetic diversity and mixing of genes amongst the population, fungi that were in their sexual stage were mated during the process.
The experiments showed that selection of plastic-adhering S. cerevisiae led to the evolution of the fungus to display multicellular traits more frequently. Typically, S. cerevisiae is found in its single-celled oval yeast form. Here, the fungus made more elongated branch-like structures called pseudohyphae, better biofilms (durable structures that help microbial communities adhere to surfaces), and multicellular floating structures called ‘flors’.
All three of these multicellular phenotypes have been associated with increased ability of fungi, including S. cerevisiae, to infect mammals by allowing fungal communities to persist and ‘stick’ within the host (Roig et al., 2013). Ekdahl et al. found that some of these multicellular phenotypes, namely the flor and biofilm formation, correlated with plastic adherence. However, pseudohyphae formation was observed even when the fungi displayed low levels of adherence, indicating that it developed independently of this trait and was instead a result of nutrient limitation.
To investigate how these multicellular adaptations impact how well the fungus can cause disease, Ekdahl et al. infected wax moth larvae with S. cerevisiae. As well as allowing relatively rapid screening of a large number of microbes at once, results from this insect model often correspond with infections in mice (Smith and Casadevall, 2021). Interestingly, the findings showed that fungi that had adapted to display multicellularity also showed increased virulence compared to the original strains and adapted isolates that were non-multicellular. This serves as a striking proof of principle that non-living environmental factors, such as plastic surfaces, can coincidentally enhance the pathogenic potential of a microbe.
The work of Ekdahl et al. illustrates several important concepts and has implications for future work on fungal pathogens. First, the findings support the accidental virulence theory by showing that adaptation to an environmental factor, independent of a host, can incidentally increase a microbe’s ability to cause disease. Second, while the genetic basis of the increased multicellularity was not elucidated, the fungal specimens that were generated can be studied in the future to fully understand how the fungi gained these new characteristics. Lastly, showing that plastic adhesion can enhance fungal virulence serves as a warning as environmental pollution with microplastics becomes increasingly ubiquitous (Hale et al., 2020). Consequently, environmental fungi may become better adapted to living on plastic surfaces, resulting in them gaining virulent traits which could potentially contribute to emergence of new types of fungal infection.
References
-
Invasive Saccharomyces infection: a comprehensive reviewClinical Infectious Diseases 41:1559–1568.https://doi.org/10.1086/497832
-
BookPhagosomal acidification mechanisms and functional significanceIn: Gordon S, editors. In Advances in Cellular and Molecular Biology of Membranes and Organelles. Phagocytosis The Host. pp. 299–319.
-
A global perspective on microplasticsJournal of Geophysical Research 125:e2018JC014719.https://doi.org/10.1029/2018JC014719
Article and author information
Author details
Publication history
- Version of Record published: January 3, 2024 (version 1)
Copyright
© 2024, Smith
This article is distributed under the terms of the Creative Commons Attribution License, which permits unrestricted use and redistribution provided that the original author and source are credited.
Metrics
-
- 729
- views
-
- 76
- downloads
-
- 1
- citations
Views, downloads and citations are aggregated across all versions of this paper published by eLife.
Download links
Downloads (link to download the article as PDF)
Open citations (links to open the citations from this article in various online reference manager services)
Cite this article (links to download the citations from this article in formats compatible with various reference manager tools)
Further reading
-
- Biochemistry and Chemical Biology
- Evolutionary Biology
Stramenopiles form a clade of diverse eukaryotic organisms, including multicellular algae, the fish and plant pathogenic oomycetes, such as the potato blight Phytophthora, and the human intestinal protozoan Blastocystis. In most eukaryotes, glycolysis is a strictly cytosolic metabolic pathway that converts glucose to pyruvate, resulting in the production of NADH and ATP (Adenosine triphosphate). In contrast, stramenopiles have a branched glycolysis in which the enzymes of the pay-off phase are located in both the cytosol and the mitochondrial matrix. Here, we identify a mitochondrial carrier in Blastocystis that can transport glycolytic intermediates, such as dihydroxyacetone phosphate and glyceraldehyde-3-phosphate, across the mitochondrial inner membrane, linking the cytosolic and mitochondrial branches of glycolysis. Comparative analyses with the phylogenetically related human mitochondrial oxoglutarate carrier (SLC25A11) and dicarboxylate carrier (SLC25A10) show that the glycolytic intermediate carrier has lost its ability to transport the canonical substrates malate and oxoglutarate. Blastocystis lacks several key components of oxidative phosphorylation required for the generation of mitochondrial ATP, such as complexes III and IV, ATP synthase, and ADP/ATP carriers. The presence of the glycolytic pay-off phase in the mitochondrial matrix generates ATP, which powers energy-requiring processes, such as macromolecular synthesis, as well as NADH, used by mitochondrial complex I to generate a proton motive force to drive the import of proteins and molecules. Given its unique substrate specificity and central role in carbon and energy metabolism, the carrier for glycolytic intermediates identified here represents a specific drug and pesticide target against stramenopile pathogens, which are of great economic importance.
-
- Evolutionary Biology
- Genetics and Genomics
A protein’s genetic architecture – the set of causal rules by which its sequence produces its functions – also determines its possible evolutionary trajectories. Prior research has proposed that the genetic architecture of proteins is very complex, with pervasive epistatic interactions that constrain evolution and make function difficult to predict from sequence. Most of this work has analyzed only the direct paths between two proteins of interest – excluding the vast majority of possible genotypes and evolutionary trajectories – and has considered only a single protein function, leaving unaddressed the genetic architecture of functional specificity and its impact on the evolution of new functions. Here, we develop a new method based on ordinal logistic regression to directly characterize the global genetic determinants of multiple protein functions from 20-state combinatorial deep mutational scanning (DMS) experiments. We use it to dissect the genetic architecture and evolution of a transcription factor’s specificity for DNA, using data from a combinatorial DMS of an ancient steroid hormone receptor’s capacity to activate transcription from two biologically relevant DNA elements. We show that the genetic architecture of DNA recognition consists of a dense set of main and pairwise effects that involve virtually every possible amino acid state in the protein-DNA interface, but higher-order epistasis plays only a tiny role. Pairwise interactions enlarge the set of functional sequences and are the primary determinants of specificity for different DNA elements. They also massively expand the number of opportunities for single-residue mutations to switch specificity from one DNA target to another. By bringing variants with different functions close together in sequence space, pairwise epistasis therefore facilitates rather than constrains the evolution of new functions.