The biological function of an insect antifreeze protein simulated by molecular dynamics
Figures
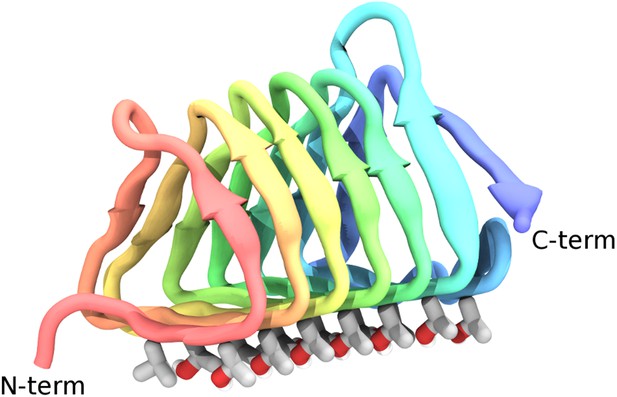
Cartoon representations of the spruce budworm AFP (sbwAFP) 501 isoform.
This shows the triangular cross section of parallel β-helix structure. The ice binding surface is drawn as sticks.
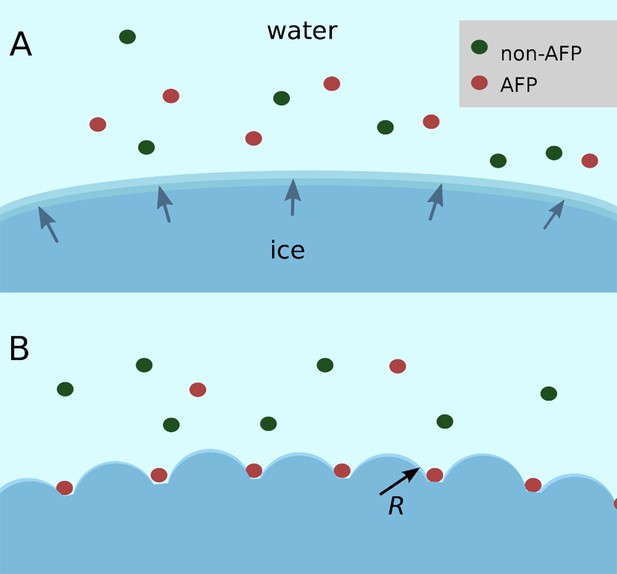
Schematic figure of antifreeze protein (AFP) ice inhibition via the Gibbs–Thomson effect.
(A) A mixture of AFP and non-AFP proteins ahead of a growing ice front. (B) AFPs selectively bind to the ice, while non-AFPs are excluded. AFP adsorption to the ice surface subdivides into smaller growth fronts which increases local curvature. If the induced curvature of the new ice fronts is less than the critical radius (R) of an ice embryo at a given temperature then the ice remains in equilibrium with the melt.
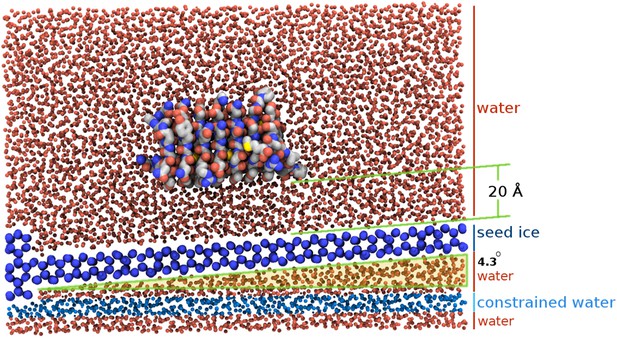
Section view of the sbwAFP simulation setup.
The seed ice crystal is shown in dark blue, constrained disordered water coloured cyan and unrestrained water is coloured red. The sbwAFP is centrally positioned 20 Å above the seed ice. The prism face of the seed ice crystals is angled approximately 4.3° with respect to the long axis of the simulation box, such that the periodic image of the seed ice is consistent with itself at the edges.
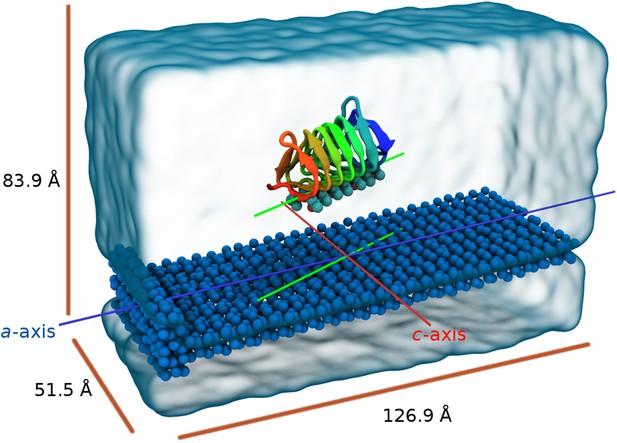
Perspective view of the model showing the seed ice as dark blue spheres and free waters as transparent surface.
The blue line is parallel to the a-axis of ice (prism face) while the red line is parallel to the c-axis of ice. The green lines represent the relative alignment of the sbwAFP with the ice.
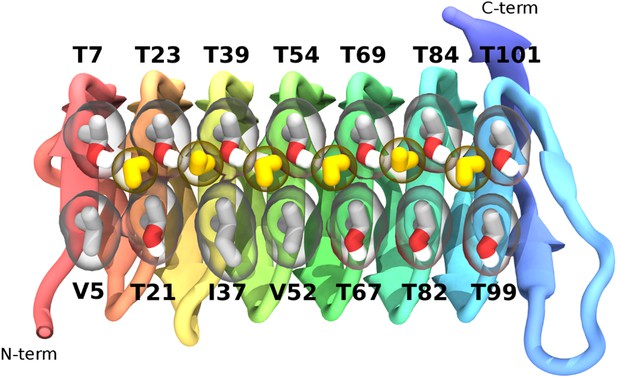
The ice-binding surface of sbwAFP.
Ordered water molecules are shown in yellow hydrogen bonded between adjacent threonine residues of the top row.
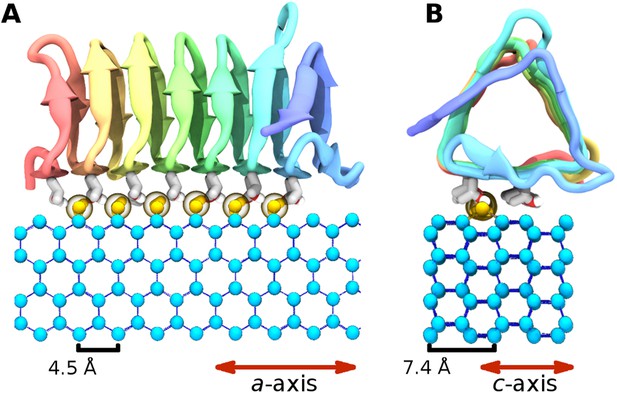
The ice binding arrangement of spruce budworm AFP in relation to ice.
(A) c-axis view of ice (cyan spheres) and the arrangement of the sbwAFP ordered water (yellow spheres). (B) a-axis view of the sbwAFP binding orientation showing the relative arrangement of the ordered water to ice. The ice lattice repeats approximately 4.5 Å and 7.4 Å along the a axis and c axis respectively.
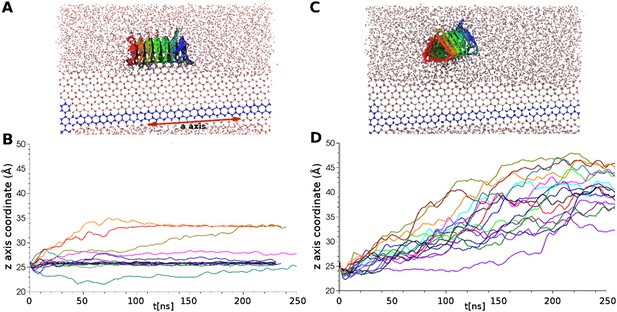
Simulations of ice docking of wildtype and inactive mutant sbwAFP against a growing ice surface.
(A) Representative side view of final docking orientation of wild type sbwAFP. (B) Overlay of 16 independent wild type sbwAFP docking simulations mapping the centre of mass z-axis coordinate of the AFP over time, an unchanging z-coordinate shows ice binding. (C) Representative side view of mutant sbwAFP in simulation. (D) Overlay of 16 independent mutant sbwAFP docking simulations showing all AFPs diffusing ahead of the ice front and no ice binding events. The mutant form of the sbwAFP consisted of 4 Thr to Leu mutations at positions 7, 21, 39 and 69 which disrupts the ice binding surface.
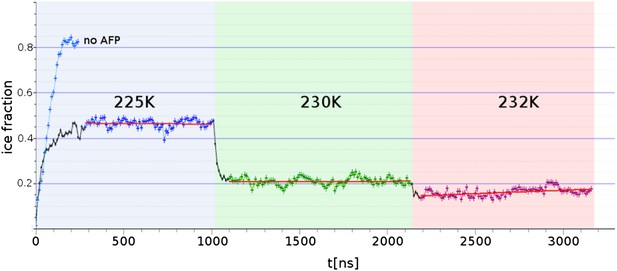
Freezing and melting inhibition of ice by adsorbed sbwAFP.
Ice fraction of 3 contiguous ∼1 microsecond simulation temperatures 225 K (approx. 5 K below melting point Tm), 230 K (approx. at Tm) and 232 K (approx. 2 K above Tm). Linear regression of equilibrated states shows no appreciable growth or melting (red lines). A simulation containing no AFP (blue line on left) shows rapid freezing of the entire model.
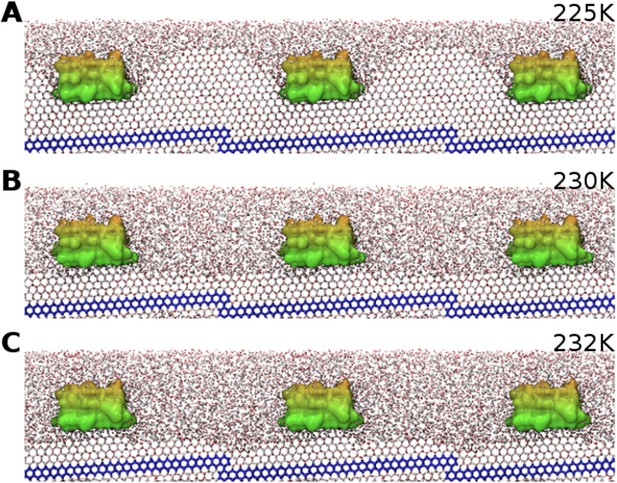
SbwAFP simulation side-profile snapshots equilibrated at different temperatures.
(A) Representative snapshot of cross-section view of equilibrated simulation (with adjacent periodic boundary images) at 225 K showing curvature of ice between adsorbed sbwAFP (seed ice is blue). (B) Side view of equilibrated simulation at 230 K showing a relatively flat ice front. (C) The simulation equilibrated at 232 K showing still adsorbed sbwAFP stabilizing ice protrusions on the superheated ice.
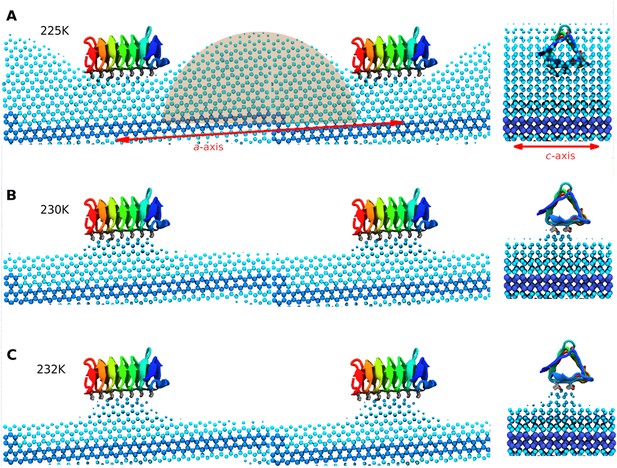
Side and end views of steady-state equilibrated ice fronts inhibited by adsorbed sbwAFP.
Cyan spheres represent the averaged ice front (defined as water occupation of greater than 85% averaged over approximately 1 microsecond simulation for each temperature). Dark blue spheres represent the constrained ice seed. (A) At 225 K the ice front shows curvature around the adsorbed sbwAFP. The middle shaded circle segment has a radius of 46 Å equal to the theoretical Gibbs–Thomson cylindrical curvature of TIP4P water at 5 K supercooling. (B) At 230 K the ice retreats, but is stabilized directly beneath the bound sbwAFP. (C) At 232 K, (approximately 2°C above Tm), the bound sbwAFP remains in place stabilizing an ice protrusion, pinning back further ice retreat. The side profile views are made from two adjacent simulation periodic images.
Videos
Representative simulation of spruce budworm AFP (sbwAFP) docking to ice.
Side view of unconstrained sbwAFP docking to the prism face of a growing ice surface at 228 K. (250 nanosecond total simulation.) The seed ice crystal is in dark blue.
Representative simulation of mutant sbwAFP.
Unconstrained sbwAFP mutant simulated at 228 K for 250 nanoseconds. Ice binding ability has been lost by mutation of 4 threonine residues (7, 21, 39 and 69) of the ice binding surface to leucine.
Ice-bound sbwAFP simulated for 1000 nanoseconds at 232 K, showing stabilization of the ice front by the presence of the bound sbwAFP.
https://doi.org/10.7554/eLife.05142.015Simulation of sbwAFP binding to the prism ice front at 225 K.
The adsorption of the sbwAFP to the prism face causes curvature to the ice consistent with the Gibbs–Thomson effect.
Continuation of Video 4 showing the ice curvature with periodic boundary conditions applied.
The second half of the video highlights the positions of the bound water molecules and shows how they migrate to the ice binding surface from the beginning of the simulation.
Additional files
-
Supplementary file 1
sbwAFP ice/water interface model. A pdb files of the initial sbwAFP ice water model is included. Constrained water molecules of the ice seed and disordered waters have a 1.0 value in the beta column.
- https://doi.org/10.7554/eLife.05142.018