Wss1 metalloprotease partners with Cdc48/Doa1 in processing genotoxic SUMO conjugates
Figures
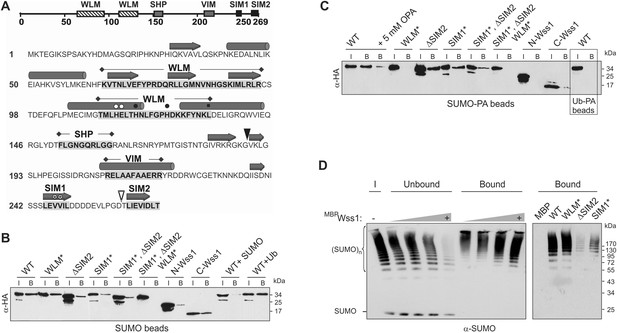
Wss1 is a SUMO-targeted metalloprotease.
(A) Structure of Wss1 protein. Amino acids of the active site are marked by circles; structural Leu 133 of the active site is marked by square; empty circles show HE115,116NK mutation in WLM* construct; asterisks show VV247,248AA mutation in SIM1* construct; empty triangle shows truncation in ΔSIM2 construct; filled triangle shows Wss1 self-cleavage site that splits protein into N-Wss1 and C-Wss1. (B) Wss1 binding to SUMO beads. Recombinant HA-Wss1 proteins were produced with pRSET-B vector in bacteria and purified (see Figure 1A and Supplementary file 4 for constructs description). SUMO beads were prepared by SUMO crosslinking to CNBr-activated Sepharose (‘Materials and methods’). The ‘input’ (I) and ‘bound’ (B) fractions were analyzed by western blotting with α-HA. For competition 10 mg/ml of SUMO or Ub was used. Input: 20%. (C) Wss1 binding to SUMO-phosphonic acid (SUMO-PA) beads. SUMO-PA was synthesized by native chemical ligation and coupled to CNBr-activated Sepharose (‘Materials and methods’). The binding assay was performed as in Figure 1B except that the concentration of Triton X-100 in binding buffer was ten times higher (1%). Addition of o-phenantroline is indicated as OPA. As a control experiment, the binding of Wss1 to Ub-PA beads is shown in the box. Input: 20%. (D) Wss1 affinity for SUMO chains. Recombined MBP-Wss1 proteins were expressed with pMAL-c2 vector in bacteria and purified (‘Materials and methods’). Their capacity to bind preformed SUMO chains was analyzed by western blotting with α-SUMO (all fractions were analyzed at the same dilution). Left panel: increasing amount of MBP-Wss1 WT protein was used to pull down SUMO chains with amylose beads. The ‘input’ (I), ‘bound’, and ‘unbound’ fractions are shown. Right panel: comparative efficiency of SUMO chain pull-down by MBP-Wss1 mutants bound to the same amount of amylose beads. Prior the experiment, the beads was loaded with an excess of the indicated MBP protein.

Structural organization of WLM proteins.
(A) Wss1 domain organization was determined by BLOCKS program by analyzing 12 WLM proteins. (B) Conserved motifs shared by WLM proteins are shown. Amino acid residues potentially involved in the organization of the active site and in the regulation of enzymatic activity are marked by asterisk. (C) Structural comparison of WLM and SprT families using Wss1 and DVC1/Spartan as examples. DVC1/Spartan and 2 proteins from WLM and SprT families with similar domain organization are shown.

Structural comparison of WLM domain.
Molecular modeling suggests similar structural organization of WLM and SprT metalloprotease domains. 3D structures of Wss1 and DVC1/Spartan active sites were modeled using Phyre2 server. The folds predicted to be similar to abylysin (4JIU) of minigluzincin family of metalloproteases with high confidence score of 99.8 (Wss1) and 98.5 (DVC1/Spartan). The active site Zn ligands are shown in red. The conserved structural hydrophobic residue of the active site is shown in green. See also corresponding .pdb files: Supplementary files 5–7.
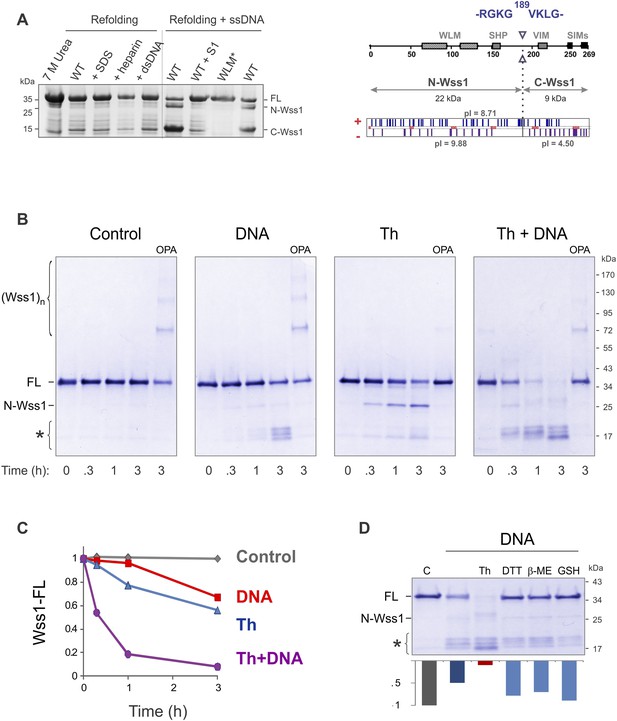
Protease activity of Wss1 protein.
(A) Wss1 self-cleavage is induced by ssDNA. Wss1-His6 protein was purified by Ni-NTA chromatography in denaturing conditions and refolded by gradient dialysis in the presence of various cofactors, including SDS (0.1%), heparin (200 μg/ml), plasmid DNA (100 μg/ml), and ssDNA (M13mp18, 100 μg/ml). The samples were analyzed by PAGE with Coomassie staining. Refolding in the presence of ssDNA produced soluble (C-Wss1) and insoluble fragments (FL and N-Wss1). The reaction was inhibited by pre-treatment of ssDNA with S1 nuclease (5 U/ml, 30 min at 37°C). No cleavage was observed with WLM* mutant. The diagram on the right shows the result of sequencing of soluble C-terminal fragment: site of cleavage, protein pI, and molecular weight as well as distribution of positively and negatively charged residues. (B) Activation of Wss1 cleavage by various conditions. Recombinant HA-Wss1 protein (200 μg/ml) was incubated for the indicated time at 25°C. Where indicated 2.5 μM DNA (DNA, 70b mbpTop1d oligonucleotide), 0.5 mM thiram (Th), or 3 mM OPA was added at the beginning of the incubation. The reaction was analyzed by non-reducing gel electrophoresis and Coomassie staining and quantified by ImageJ. The positions of full-length HA-Wss1 (FL), large N-terminal fragment (N-Wss1), small cleavage products (asterisk), and HA-Wss1 oligomers (Wss1)n are shown. (C) Kinetics of Wss1 cleavage. Diagram shows the time course of the level of full-length HA-Wss1 at various conditions. The data are obtained by ImageJ quantification of the experiments shown on Figure 2B. (D) Effect of thiol reagents on DNA-dependent Wss1 activation. Recombinant HA-Wss1 protein (200 μg/ml) was incubated for 3 hr at 25°C with (DNA) or without (Control) 2.5 μM DNA (DNA, 70b mbpTop1d oligonucleotide). Where indicated 0.5 mM thiram (Th), 1 mM dithiothreitol (DTT), 5 mM beta-mercaptoethanol (β-ME), of 10 mM glutathione (GSH) was added at the beginning of the incubation. The reaction was analyzed by gel electrophoresis and Coomassie staining and quantified by ImageJ.
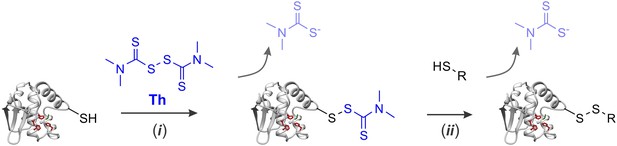
Modification of protein sulfhydryl groups by thiuram disulfides.
(i) Thiol-disulfide exchange reaction between protein sulfhydryl groups and thiram (Th) results in formation of protein–thiram mixed disulfide. When thiram is present in excess, this is the primary reaction product. (ii) With sub-stoichiometric amounts of thiram, the protein-thiram disulfide may further react with thiols producing inter- and intra-molecular disulfides. In general, protein modification by thiram can be fully reversed by an excess of thiols.
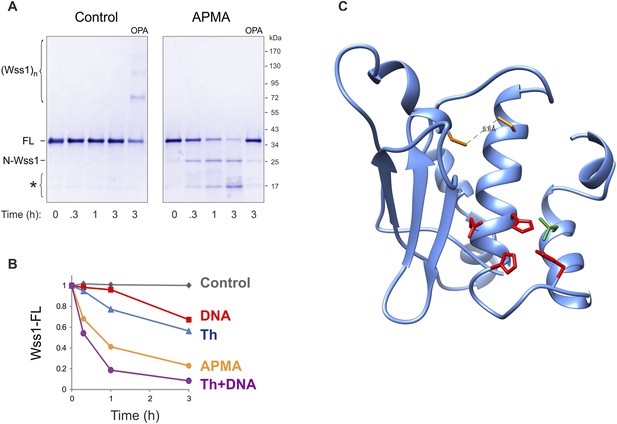
Activation of Wss1 cleavage by APMA & Wss1 cysteines.
(A) Activation of Wss1 cleavage by 4-aminophenylmercuric acetate (APMA). Recombinant HA-Wss1 protein (200 μg/ml) was incubated with or without 1 mM APMA for the indicated time at 25°C. Where indicated 3 mM OPA was added at the beginning of the incubation. The reaction was analyzed by non-reducing gel electrophoresis and Coomassie staining and quantified by ImageJ. The positions of full-length HA-Wss1 (FL), large N-terminal fragment (N-Wss1), small cleavage products (asterisk), and HA-Wss1 oligomers (Wss1)n are shown. (B) Kinetics of Wss1 cleavage. Diagram shows the time course of the level of full-length HA-Wss1 at various conditions. The data are obtained by ImageJ quantification of the experiments shown on Figure 3B and Figure 3—figure supplement 1A. (C) Localization of the cysteines C96 and C108 within the WLM domain. Their mutual orientation suggest possible disulfide bond, though the estimated distance of 5.6 A is too long. The cysteines are shown in yellow. The active site Zn ligands are shown in red. The conserved structural hydrophobic residue of the active site is shown in green. The same WLM structure as in Figure 1—figure supplement 2 is used.
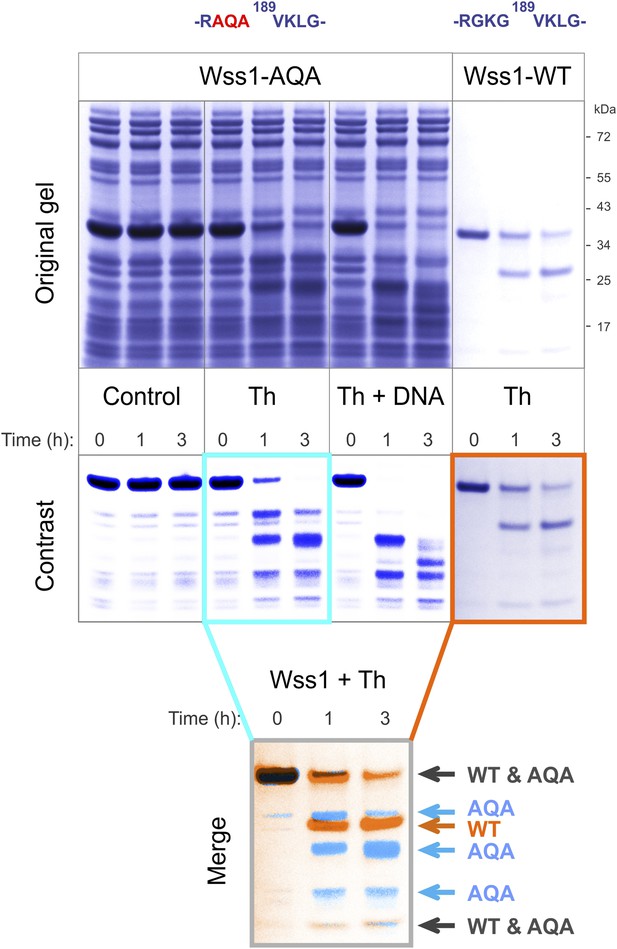
Cleavage of Wss1-AQA mutant.
Partially purified HA-Wss1-AQA mutant (500 μg/ml of total protein) and wild-type protein (100 μg/ml) were incubated for the indicated time at 25°C. Where indicated 2.5 μM DNA (DNA, 70b mbpTop1d oligonucleotide) or 0.5 mM thiram (Th) was added at the beginning of the incubation. The reaction was analyzed by gel electrophoresis and Coomassie staining and Wss1 protein fragments were analyzed using Adobe Photoshop software. The positions of common (black) and unique (false-colored) fragments are shown for AQA mutant (blue) and WT protein (red).
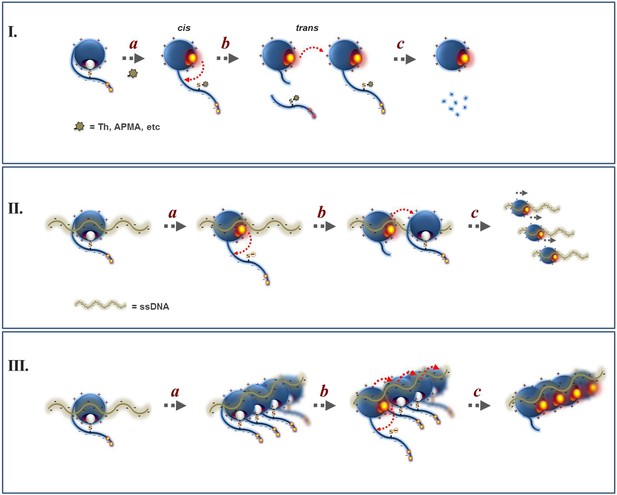
Proposed mechanism for the regulation of Wss1 protease activity by cysteine switch mechanism.
(I) Mechanism of Wss1 activation by thiol-reactive electrophiles. (a) Modification of the regulatory cysteine by thiram (Th) or APMA displaces the cysteine from the active site Zn, activates the metalloprotease and induces in-cis Wss1 cleavage. (b) Activated Wss1 may also proteolyze other Wss1 molecules acting in-trans as endopeptidase or caboxypeptidase. (c) In-trans proteolysis results in gradual degradation of Wss1 pool, the most persistent fragment being a compact WLM domain. (II) Activation of Wss1 proteolysis by ssDNA. The DNA may act in two ways. (a) First, interaction of a positively charged WLM domain with DNA may induce conformational changes facilitating displacement of the negatively charged C-terminal peptide with an inhibitory cysteine from the active site. This may promote the initial event of Wss1 activation. The process is not efficient and can be reversed by thiols such as DTT and glutathione (Figure 3D). (b) Then, DNA may facilitate Wss1 intermolecular interaction and greatly promote in-trans proteolysis. (c) This results in rapid propagation of proteolytic activity and degradation of the Wss1 pool. (III) Cooperative mechanism. The DNA may induce Wss1 oligomerization (a), whereby initial in-cis cleavage (b) is followed by in-trans proteolysis of the whole oligomer (c).
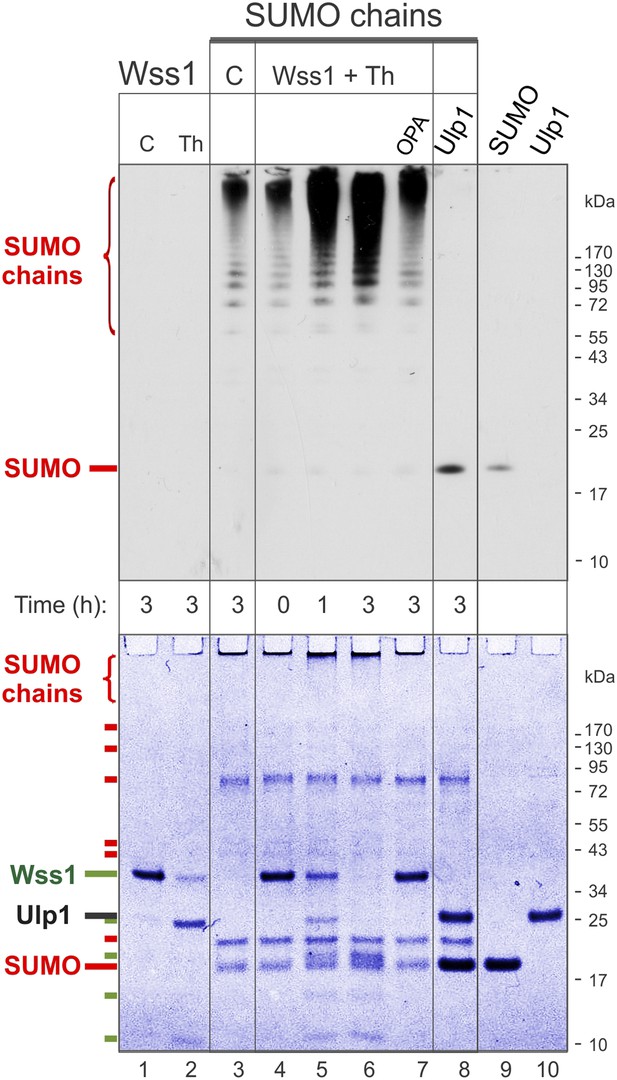
Processing of SUMO chains by Wss1.
Comparative analysis of SUMO chains processing. Preformed SUMO-chains (200 μg/ml of SUMO monomer) were incubated with 100 μg/ml of recombinant HA-Wss1 (Wss1) or 100 μg/ml of Ulp1 for the indicated time at 25°C. Where indicated 0.5 mM thiram (Th) or 3 mM OPA was added. Control reactions (C) contain only Wss1 or polySUMO as indicated. The reaction was analyzed by western blotting with α-SUMO (top panel) and by gel electrophoresis with Coomassie staining (bottom panel). The positions of full-length HA-Wss1 (Wss1) and HA-Wss1 cleavage fragments are indicated in green. The positions of SUMO, SUMO chains, and SUMO E1–E3 components are shown in red.
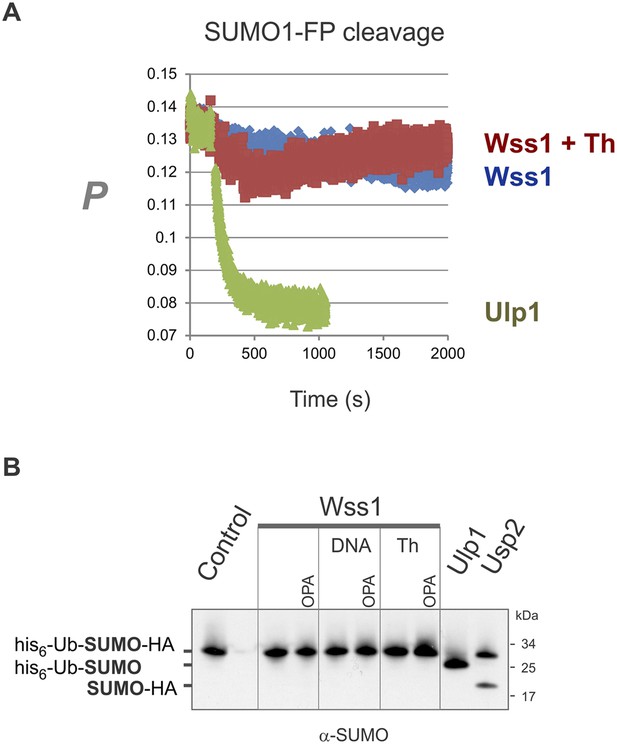
Wss1 cleavage of SUMO1-FP and His6-Ub-SUMO-HA substrates.
(A) Proteolysis of SUMO1-FP substrate. Fluorescence anisotropy measurements with 100 nM SUMO1-FP substrate were performed on a MOS-450 spectrometer (BioLogic, Inc.) in a 150-μl quartz cuvette at 25°C essentially as described (Geurink et al., 2012). The proteolysis was initiated at 200 s by addition of 1 μg/ml (final concentration) of SUMO isopeptidase Ulp1, or 20 μg/ml of HA-Wss1 with or without 0.5 mM thiram (Th). (B) Proteolysis of His6-Ub-SUMO-HA substrate. Purified His6-Ub-SUMO-HA substrate (100 μg/ml) was incubated with 100 μg/ml of recombinant HA-Wss1 (Wss1), 50 μg/ml of SUMO isopeptidase Ulp1, or 50 μg/ml of Ub isopeptidase Usp2 (catalytic domain) for 3 hr at 25°C. Where indicated 2.5 μM DNA (DNA, 70b mbpTop1d oligonucleotide), 0.5 mM thiram (Th), or 3 mM OPA was added. The reaction was analyzed by western blotting with α-SUMO antibody.
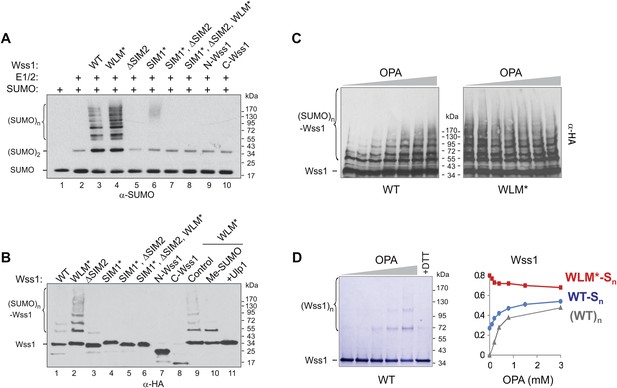
SUMO ligase activity of Wss1 protein.
(A) Wss1 promotes SUMO chain formation. In vitro sumoylation reaction with HA-Wss1 constructs purified from bacteria (‘Materials and methods’). The reaction was analyzed by western blotting with α-SUMO. (B) Auto-sumoylation of Wss1. In vitro sumoylation reaction with HA-Wss1 constructs analyzed by western blotting with α-HA. Lanes 9–11 show auto-sumoylation of WLM mutant. Lane 10: reaction with per-methylated SUMO. Lane11: Ulp1 was added after reaction. (C) Effect of OPA on Wss1 auto-sumoylation. The reactions were performed in the presence of increasing concentration of OPA (0–3 mM, see diagram below in Figure 4D). The level of Wss1-SUMO conjugates (Wss1-Sn) was analyzed by western blotting with α-HA and quantified by ImageJ. (D) OPA induces oligomerization of WT-Wss1. Recombinant HA-Wss1 protein (200 μg/ml) was incubated for 2 hr at 25°C with increasing concentration of OPA (0–3 mM, see diagram on the right). The reaction was analyzed by non-reducing gel electrophoresis and Coomassie staining and quantified by ImageJ. In the last lane, 200 mM DTT was added. Diagram on the right summarizes the effect of OPA on the level of sumoylated Wss1 (WT-Sn and WLM*-Sn) and Wss1 oligomers (WT)n. The data are normalized to the initial value at 0 mM OPA.
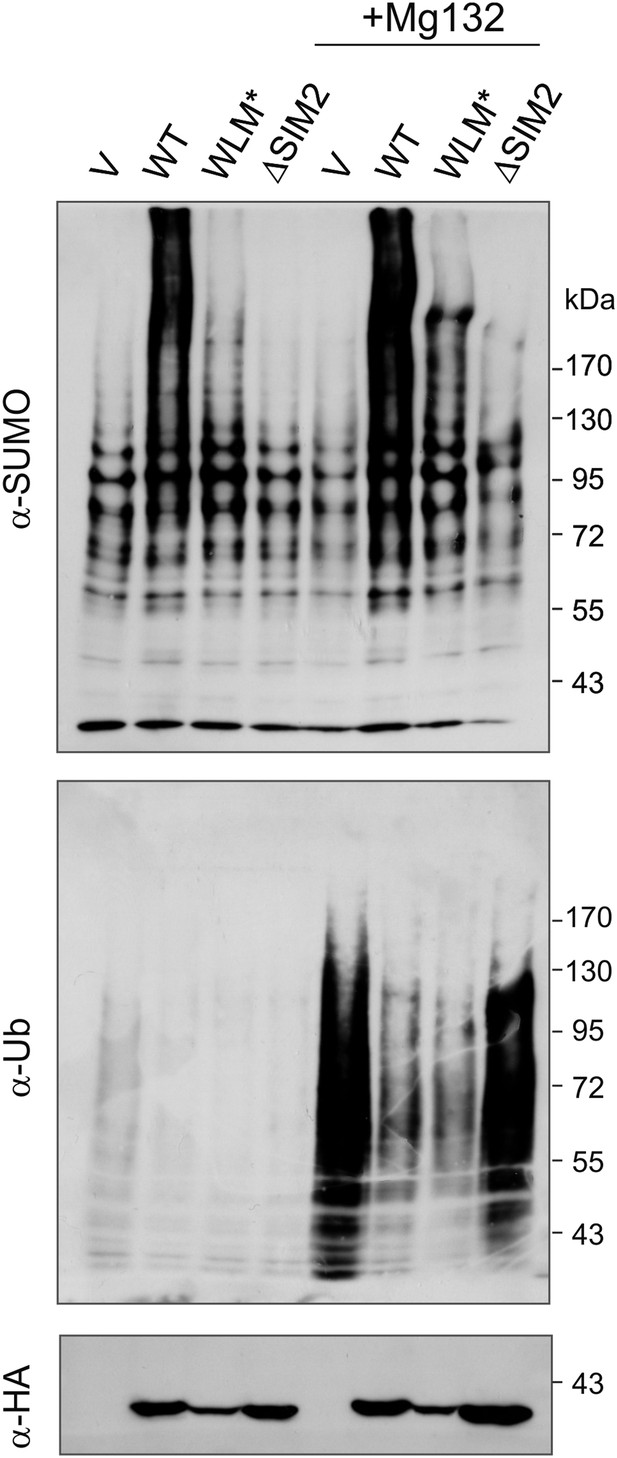
Wss1 and STUbL may have common cellular substrates.
Wss1 counteracts ubiquitylation of SUMO conjugates by STUbL. HA-Wss1 constructs or pYEPGAP-URA3 vector alone (V, see Supplementary file 4 for constructs description) was transformed into EJY251-11b cells that express HIS6-FLAG-tagged (HF)-SUMO as the only SUMO source (Supplementary file 3). Cells were grown to early exponential phase in galactose and proline-containing medium and treated or not with 75 μM MG-132 for 3 hr. Whole cell lysates (WCLs) were prepared under denaturing conditions and SUMO conjugates were isolated on Ni-NTA agarose. The fractions were analyzed by western blotting with specific antibodies (α-SUMO and α-Ub). Bottom western blot shows HA-Wss1 constructs in WCL.

Proposed mechanism for Wss1 SUMO-ligase activity.
(A) Wss1 protein has a positively charged N-terminal WLM domain and negatively charged C-terminal extension that contains two SIMs and conserved cysteine C226. This cysteine may be linked to the active site Zn counteracts, the interaction facilitated by charge interaction of the two protein parts. Wss1 E3-like ligase activity (Figure 2A,B) results from the juxtaposition of an E2∼SUMO thiol ester and an acceptor SUMO due to SUMO binding by two carboxy-terminal SIMs. The tendency of Wss1 to form oligomers further promotes Wss1-dependent sumoylation by multiplying SUMO binding sites. (B) Extraction of the active site Zn by o-Phenanthroline (OPA) release the cysteine 226, which is prone to produce intermolecular disulfide crosslinks with its homolog (forming Wss1 dimer) or one of the two cysteines localized in the WLM domain (forming Wss1 oligomers). Multiplication of the SIMs in Wss1 oligomers further promotes SUMO ligase activity.
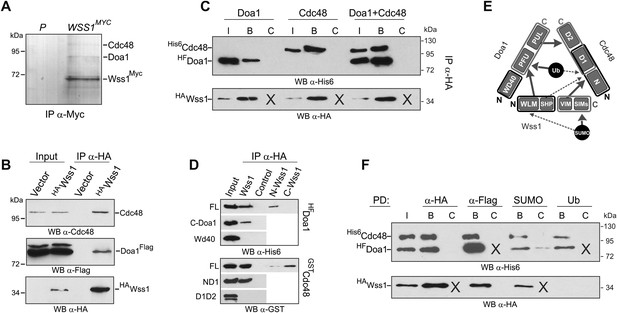
Wss1 forms SUMO-specific ternary complex with Cdc48 and Doa1.
(A) Purification of Wss1-interacting proteins. Gel electrophoresis of the proteins isolated on anti-Myc beads from parental (P, BY4742) and WSS1-MYC13 strains (MBY17, Supplementary file 3). The gel was stained with Coomassie blue. (B) Wss1 interacts with Cdc48 and Doa1. Cell lysates from DOA1-FLAG cells (MBY18, Supplementary file 3) transformed with HA-Wss1 or vector alone (pYEPGAP-URA3) were pulled down with anti-HA beads and analyzed by western blotting. Input: 10%. (C) Wss1 forms a ternary complex with Cdc48 and Doa1. HA-Wss1 protein was mixed with molar excess of His6-Cdc48 and his6-Flag (HF)-Doa1 and pulled down with anti-HA beads. The input (I) and bound (B) fractions were analyzed by western blotting. Control experiments (C) show protein binding in the absence of HA-Wss1 (crosses). Input: 20%. (D) Interaction within the Wss1/Cdc48/Doa1 ternary complex. The purified full-length (FL) or truncated versions of GST-Cdc48 and HF-Doa1 proteins were interacted with FL and truncated HA-Wss1 constructs. The proteins were pulled down with anti-HA beads and analyzed by western blotting. Input: 50%. (E) Schematic representation of protein interactions within Wss1/Cdc48/Doa1 complex. (F) Wss1/Cdc48/Doa1 ternary complex is SUMO specific. Stoichiometric amounts of HA-Wss1, His6-Cdc48, and HF-Doa1 proteins were mixed together and pulled-down with affinity beads. The ternary complex bound only to SUMO. In control experiments (C) either HA-Wss1 or HF-Doa1 protein was omitted (crosses). Input: 20%.
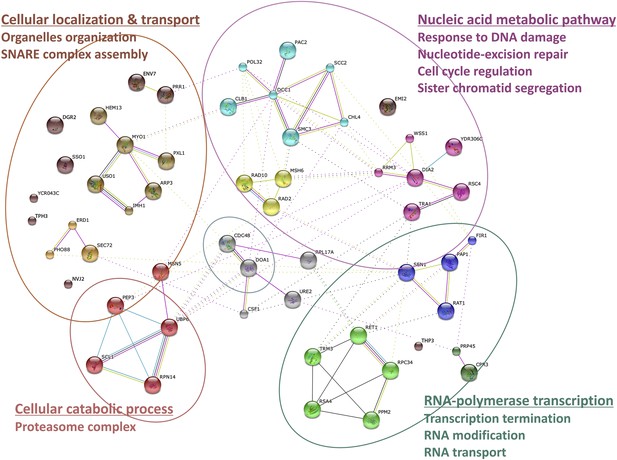
Wss1 interactome.
Wss1-interacting proteins identified by mass spectrometry, connected in network with STRING and classified using gene ontology (GO) database (see also Supplementary file 1).
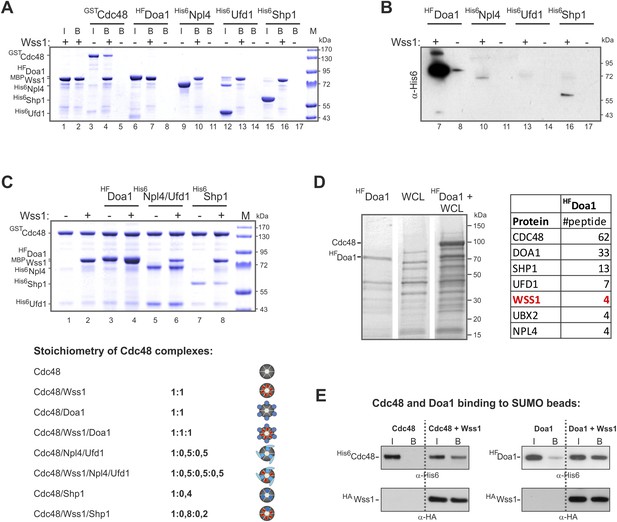
Characterization of Wss1-Cdc48-Doa1 interaction.
(A, B) Interaction of Wss1 with Cdc48 and adaptor proteins reveals Wss1 specificity for Doa1. All proteins were expressed in bacteria and purified to homogeneity. The proteins were pulled down with amylose beads in the presence or absence of recombinant MBP-Wss1 protein. Panel A: the ‘input’ (I) and ‘bound’ fractions (B) were analyzed by PAGE with Coomassie staining (volume of the ‘bound’ fraction was adjusted to have the same dilution as in ‘input’ fraction). Because HF-Doa1 migrates at the same position as MBP-Wss1 no additional band was seen in MBP-Wss1 bound fraction (lane 7). To ascertain that HF-Doa1 binds to MBP-Wss1 all bound fractions were additionally analyzed by western blotting with α-His6 antibodies (panel B). (C) Interaction of Wss1 with preformed Cdc48 complexes confirms ternary 1:1:1 Wss1/Doa1/Cdc48 complex. The Cdc48 complexes were preformed from purified GST-Cdc48 with a double molar excess of the indicated protein adaptors. Next, MBP-Wss1 was added in stoichiometric amount and Cdc48 complexes were separated on glutathione beads. The bound complexes were analyzed by PAGE with Coomassie staining, and quantified by ImageJ. The bottom picture shows the models of Cdc48 complexes resulted from ImageJ measurements. (D) Mass spectrometry analysis of Doa1-interacting proteins identifies Wss1. Purified HF-Doa1 protein was mixed with whole cell lysate and retrieved, along with the partner proteins, on Ni-NTA resin (see ‘Materials and methods’). In the control experiment, the lysate was passed through the resin without HF-Doa1. The proteins specifically retrieved with HF-Doa1 were identified by mass spectrometry. Top seven interactors are shown in the table (see also Supplementary file 2 for complete list). (E) Wss1-dependent binding of Cdc48 and Doa1 to SUMO beads. Purified of His6-Cdc48 and his6-Flag (HF)-Doa1 proteins were pulled down with SUMO beads with (right part of the blots) or without (left part) HA-Wss1 protein. The input (I) and bound (B) fractions were analyzed by western blotting. Input: 20%.
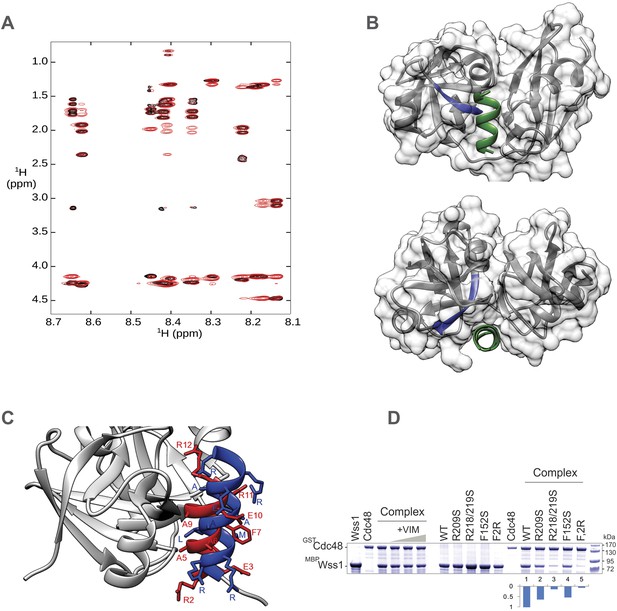
Structural basis for Wss1-Cdc48 interaction.
(A) RMN spectrum of Wss1 VIM (209–219) peptide used for structure determination. Fingerprint region of 800 MHz TOCSY (black) and NOESY (red) spectra recorded at T = 278 K. (B) Different views showing positioning of Wss1 VIM (green) and Cdc48 SIM (blue) within the central groove separating Nc and Nn-Cdc48 subdomains. (C) Identification of Wss1 VIM residues involved in Cdc48 binding. The RMN structure of Wss1 VIM peptide (in red) was docked into a model of N-Cdc48 domain based on the gp78 VIM-p97N complex (see Supplementary file 8). Both Wss1 VIM (red) and gp78 VIM show (blue) similar positioning of the side chains of the key amino acids implicated in interaction. (D) Conserved Wss1 residues from VIM and SHP domain are involved in Cdc48 binding. The MBP-Wss1/GST-Cdc48 complexes was pre-formed from purified proteins and isolated on amylose beads. Left part of the gel: Wss1 VIM peptide (209–219) does not inhibit the formation of stoichiometric 1:1 MBP-Wss1/GST-Cdc48 complex at concentrations up to 5 mg/ml, probably because it is too short to compete out Wss1, and/or because another part of Wss1 (SHP domain) is also involved in Cdc48 interaction. Right part of the gel: mutation of the conserved residues from VIM and SHP domains inhibits Cdc48 binding. F2R construct has apart from R218,219S VIM mutation, the mutation of conserved phenylalanine residue in SHP domain (F152S) known to be important for Cdc48 binding (see Figure 1—figure supplement 1B). Histograms shows GST-Cdc48: MBP-Wss1 ratio within the complexes quantified with ImageJ. See also corresponding .pse file: Supplementary file 8.
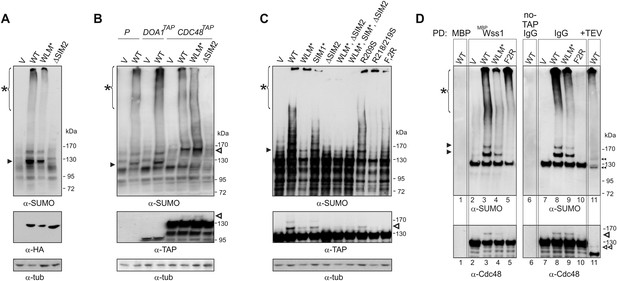
Wss1 sumoylates cellular proteins and promotes their binding to Cdc48.
(A) Wss1 sumoylates cellular proteins. HA-Wss1 constructs were expressed from pYEPGAP-URA3 vector (V) in BY4742 cells, and cell lysates were analyzed by western blotting. Abbreviations of the constructs are the same as in Figure 1A. The asterisk indicates HMW-SUMO, and the black arrowhead shows the 120 kD SUMO species. (B) Wss1 sumoylates Cdc48. Sumoylation was analyzed in parental (P, S288C) and DOA1-TAP and CDC48-TAP cells (Supplementary file 3) transformed with pYEPGAP-URA3 constructs as in Figure 6A. The asterisk indicates HMW-SUMO, and the black arrowhead shows the 120 kD SUMO species. The white arrowhead shows the 150 kD SUMO-Cdc48-TAP species. (C) Effect of Wss1 mutations on Cdc48 sumoylation in CDC48-TAP cells. The cells were transformed with pYEPGAP-URA3 constructs and analyzed by western blotting. The asterisk indicates HMW-SUMO, and the black arrowhead shows the 150 kD SUMO species. The white arrowhead shows the 150 kD SUMO-Cdc48-TAP species. (D) Wss1 promotes binding of SUMO conjugates to Cdc48. Lysates from CDC48-TAP cells transformed with Wss1 constructs were pulled down either with Wss1-beads (Wss1, ‘Materials and methods’) or IgG agarose and analyzed by western blotting. Control lanes show pull-down of CDC48-TAP cells lysate with MBP-beads (lane 1, MBP) and IgG immuno-precipitation of a lysate from non-tagged parental CDC48 cells (lane 6, no-TAP IgG, S288C). The last lane shows the eluate from WT-IgG beads (the same as lane 8) treated with 50 µg/ml TEV protease (lane 11, TEV). The asterisk indicates HMW-SUMO, the black arrowheads show the SUMO-Cdc48-TAP species, and 130 kD band is Cdc48-TAP cross-reacting with α-SUMO. The double black arrowheads show the SUMO-Cdc48 species after the cleavage of TAP-tag by TEV. The white arrowheads show SUMO-Cdc48 species before (simple) and after (double) TAP-tag cleavage by TEV.
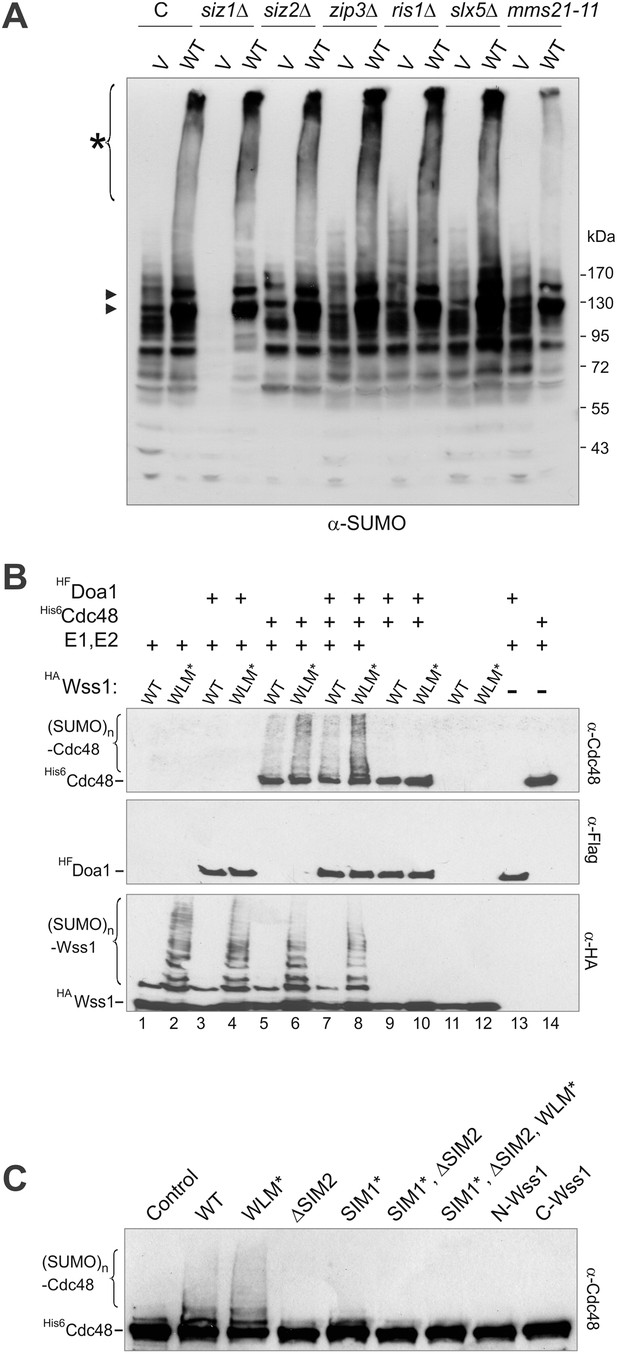
Wss1 promotes sumoylation of Cdc48 and other cellular proteins.
(A) Wss1-induced sumoylation of cellular proteins does not depend on SUMO E3-ligases. Control cells (BY4742) and cells knocked out for known and putative SUMO E3-ligase genes (YKO clones, Open Biosystems) were transformed with wild-type HA-Wss1 (WT) or pYEPGAP-URA3 -vector alone (V) and sumoylation profile was examined by WCL western blotting with SUMO -specific antibodies (80 μg of protein per lane for each sample). The asterisk indicates HMW-SUMO conjugates, and the black arrowheads show sumoylated Cdc48. (B) In vitro sumoylation with His6-Cdc48, HF-Doa1, and HA-Wss1 proteins. The proteins were expressed in bacteria and affinity purified. In vitro sumoylation reaction was performed with recombinant SUMO and SUMO E1–E2 enzymes (see ‘Materials and methods’). The reaction was analyzed by western blotting with specific antibodies. (C) Effect of Wss1 mutations on in vitro sumoylation of Cdc48. Recombinant HA-Wss1 proteins were used in in vitro sumoylation reaction with His6-Cdc48 protein. The reaction was analyzed by western blotting with Cdc48-specific antibodies.
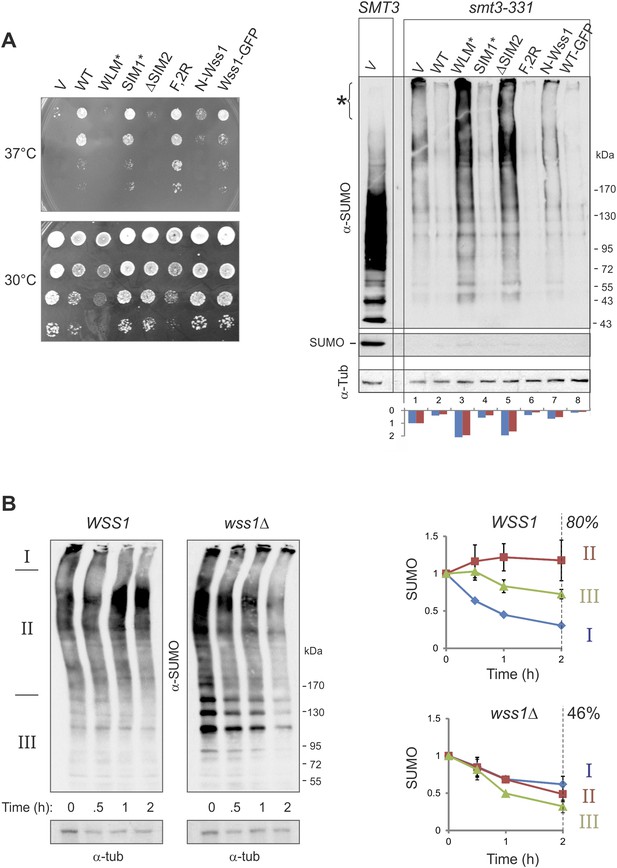
Wss1 regulates HMW-SUMO in smt3-331 cells.
(A) Suppression of Smt3-331 phenotype by Wss1. Wss1 constructs were expressed in smt3-331 wss1Δ cells (MBY13, Supplementary file 3) as HA-fusions using pYEPGAP-URA3 vector (V, etc) or as GFP fusion using pUG35 vector (Wss1-GFP, Supplementary file 4). Left panel: ts-suppression assay on SD-ura plates. Right panel: western blot analysis of SMT3 (first lane labeled SMT3, MBY14 strain) and smt3-331 cell lysates with α-SUMO. Asterisk shows HMW-SUMO. Histogram: ImageJ quantification of total (blue) or HMW-SUMO (red) conjugates, normalized to the value obtained with vector alone (V). (B) Wss1 controls HMW-SUMO. Cellular protein synthesis was inhibited in exponentially growing smt3-331 WSS1 (SBY331, Supplementary file 3) and smt3-331 wss1Δ cells (MBY13) by 50 μg/ml cycloheximide (CHX). WCL was analyzed by western blotting with α-SUMO. SUMO signal was measured by ImageJ in three zones I–III and normalized to the initial value. The plots show the kinetics of SUMO change (error bars represent standard deviations). The percentage on the right indicates total amount of SUMO signal remained after 2 hr.
-
Figure 7—source data 1
Western blot quantification for Figures 7A and 7B.
- https://doi.org/10.7554/eLife.06763.023
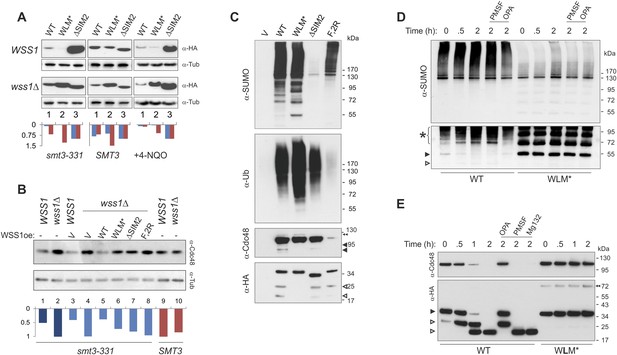
Wss1 protease is activated by DNA damage.
(A) Wss1 degradation is stimulated by DNA damage. WSS1 and wss1Δ cells (smt3-331, BY4742, and BY4742 + 0.2 μg/ml 4-NQO) were transformed with HA-Wss1 constructs (pYEPGAP-URA3 vector) and grown at 30°C. The WCLs were analyzed by western blotting with α-HA and quantified by ImageJ. Histograms show the level of HA-Wss1 constructs in WSS1 (blue) and wss1Δ (red) strains normalized to the value of ΔSIM2 protein. (B) Wss1 promotes Cdc48 degradation in smt3-331 cells. WSS1 and wss1Δ cells (smt3-331 and BY4742 strains, Supplementary file 3) were transformed with HA-Wss1 constructs and grown at 30°C. The WCLs were analyzed by western blotting with α-Cdc48 and quantified by ImageJ. Histogram shows Cdc48 signal normalized to the maximum value. (C) Composition of the Wss1 complexes. The complexes were isolated with α-HA beads from WCL of wss1Δ cells (MBY15, Supplementary file 3) transformed with HA-Wss1 constructs and treated with 0.2 μg/ml 4-NQO. Pulled down proteins were analyzed by western blotting. The double black arrowhead shows SUMO-Cdc48, black arrowheads show Cdc48 fragments, and white arrowheads show Wss1 fragments. (D) Proteolysis of SUMO-conjugates by Wss1. HA-WT and HA-WLM* complexes were isolated with α-HA beads, incubated at 30°C with or without inhibitors, and analyzed by western blotting with α-SUMO. The lysis buffer contained 30 mM NEM. The bottom panel is taken at higher exposure. The asterisk indicates HMW-SUMO-derived fragments, the black arrowhead shows SUMO-Wss1, and the white arrowhead shows SUMO-Wss1 fragment. (E) Degradation of Cdc48 and self-proteolysis. The same conditions as in Figure 8D, except that α-Cdc48 and α-HA were used for western blotting. The black arrowhead indicates full-length Wss1, and white arrowheads show Wss1 fragments. The double black arrowhead shows WLM* dimers.
-
Figure 8—source data 1
Western blot quantification for Figure 8 supplement 1A.
- https://doi.org/10.7554/eLife.06763.025
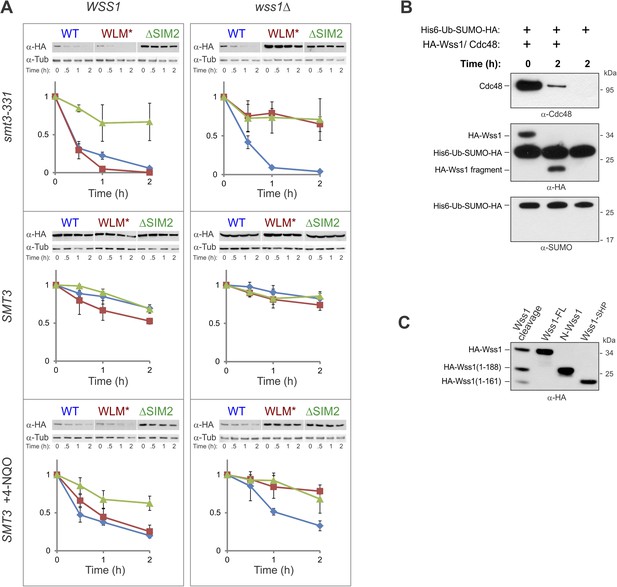
Wss1 proteolysis.
(A) Cellular stress destabilizes Wss1 protein. Kinetics of Wss1 degradation was studied on WSS1 and wss1Δ background in smt3-331 and SMT3 (BY4742) cells. The cells were transformed with HA-Wss1 constructs (pYEPGAP-URA3-WT, WLM*, and ΔSIM2) and grown at 30°C. Cellular protein synthesis was inhibited in mid-exponential phase by 50 μg/ml cycloheximide (CHX), and Wss1 protein was analyzed in WCL by western blotting with α-HA. When indicated 0.2 μg/ml 4-NQO was added 3 hr before CHX treatment. The plots show α−HA signal quantified by ImageJ and normalized to initial level for WT (blue diamonds), WLM*(red squares), and ΔSIM2 (green triangles) proteins. Error bars represent standard deviations from triplicate observations. (B) Wss1 does not cleave His6-Ub-SUMO-HA substrate. Affinity isolated activated HA-Wss1protein (see also Figure 8E) undergoes self-processing and degrades Cdc48 protein but not His6-Ub-SUMO-HA substrate. (C) Mapping of Wss1 fragments with recombinant truncated constructs purified from bacteria. See also corresponding source file: Figure 8—source data 1.xlsx.
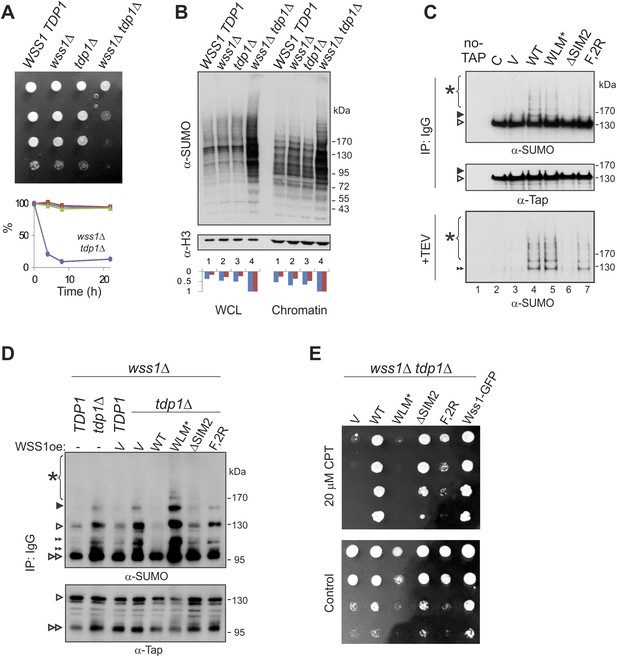
Wss1 processes Top1 topoisomerase.
(A) Wss1 is required for normal growth of tdp1Δ cells. Same number of parental (BY4742) and mutant (MBY15, MBY31, and MBY37, Supplementary file 3) cells was spotted on YPD plates and incubated for 2 days at 30°C (without CPT). Diagram shows the viability of cells treated with 20 μM CPT for indicated times (estimated by colony-forming assay): WSS1 TDP1 (red squares), wss1Δ (green triangles), tdp1Δ (blue diamonds), and wss1Δ tdp1Δ (magenta circles). (B) The wss1Δ tdp1Δ cells accumulate SUMO conjugates. WCL and chromatin fractions were analyzed by western blotting with α-SUMO. Asterisk shows HMW-SUMO. Histograms show ImageJ quantification of total (blue) or HMW (red) SUMO conjugates, normalized to the maximum value. (C) Wss1 promotes sumoylation of Top1. Top and central panels: Western blot analysis of Top1-TAP complexes isolated on IgG beads from TOP1-TAP wss1Δ cells (MBY35) transformed or not (C, control lane) with HA-Wss1 constructs (pYEPGAP-URA3 vector). Control pull-down from non-tagged parental TOP1 cells (S288C) is also shown (lane 1, no-TAP). Bottom panel: Western blot analysis of the eluates from IgG beads (the same as above) treated with 50 µg/ml TEV protease. Asterisk indicates HMW-SUMO. The black arrowheads show the position of SUMO-Top1-TAP, white arrowheads show Top1-TAP protein, and double black arrowhead shows SUMO-Top1 after cleavage by TEV. (D) Accumulation of sumoylated Top1 in wss1Δ tdp1Δ cells. Top1-TAP was isolated on IgG beads from TOP1-TAP wss1Δ (MBY35) or TOP1-TAP wss1Δ tdp1Δ (MBY39) cells transformed or not with HA-Wss1 constructs (pYEPGAP-URA3, V indicates vector alone) and analyzed by western blotting. Asterisk shows HMW-SUMO, white arrowheads show the position of Top1-TAP overlapping with one of the SUMO species, black arrowhead: SUMO-Top1-TAP, double black arrowheads: SUMO species and double white arrowheads: Top1-TAP fragment. (E) Suppression of wss1Δ tdp1Δ sickness by Wss1. The wss1Δ tdp1Δ cells (MBY39) were transformed with Wss1 constructs, spotted on SD- ura plates with or without 20 μM CPT and incubated at 30°C.

Wss1 protects cells from genotoxic threats.
(A) Wss1 overexpression (Wss1oe, pYEPGAP-URA3-WT construct) suppresses accumulation of HMW-SUMO conjugates in smt3-331 cells (SBY331 and MBY13 strains, Supplementary file 3). Whole cell lysate (WCL) and chromatin fraction were analyzed by western blotting with SUMO-specific antibodies (80 μg of protein per lane for each sample). Asterisk indicates HMW–SUMO conjugates. Histograms represents ImageJ quantification of total (blue) or HMW (purple)–SUMO conjugates, normalized to the maximum value. Bottom panel: Wss1 expression suppresses temperature sensitivity of smt3-331 cells. Smt3-331 WSS1, smt3-331 wss1Δ, and smt3-331 wss1Δ cells overexpressing Wss1 protein (Wss1oe, pYEPGAP-URA3-WT construct) were spotted on SD-ura plates, and incubated at 30°C or 37°C. (B) Wss1 protects cells from Top1-associated cytotoxicity. The wss1Δ tdp1Δ mutant sickness depends on Top1 expression. The model stains expressing MBP-tagged Top1 protein under control of GAL promoter were constructed on BY4742 and MBY24 (WSS1-GFP) genetic backgrounds (MBY42-44,49 and MBY46,47 correspondingly, Supplementary file 3). The cells were spotted on selective media and incubated at 30°C. The bottom western blots for each panel show the corresponding expression of MBP-Top1 protein detected with MBP-specific antibody. As expected, no MBP-Top1 protein was detected in cells grown on glucose medium. (C) Wss1 prevents accumulation of HMW-SUMO conjugates in UV-irradiated cells (BY4742 and MBY15 strains, Supplementary file 3). Single UV dose (254 nm, 200 J/m2) induces increase in sumoylation of cellular proteins as shown by western blotting with SUMO-specific antibodies (80 μg of protein per lane for each sample). Both whole cell lysate (WCL) and chromatin fraction were analyzed. Asterisk indicates HMW SUMO conjugates. Histograms represents ImageJ quantification of total (blue) or HMW (purple) SUMO conjugates, normalized to the maximum value. Bottom panel: Wss1 protects cells from UV-induced DNA damage. WSS1 (BY4742) and wss1Δ (MBY15) cells were spotted on YPD plates, exposed (UV) or not (C) to a single UV dose (254 nm, 100 J/m2), and incubated for 2 days at 30°C.
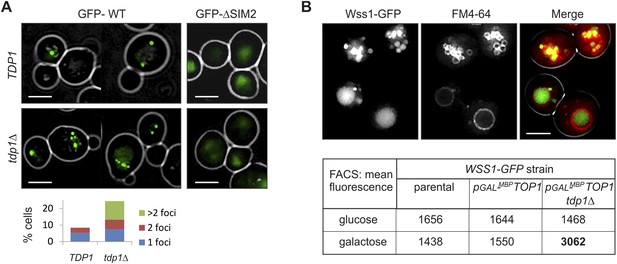
Wss1 localization upon DNA damage.
(A) Increased incidence of GFP-Wss1 foci in tdp1Δ cells. Live cells (BY4742 and MBY31 strains) expressing GFP-Wss1 WT and ΔSIM2 constructs (pUG36 vector with inducible MET25 promoter, Supplementary file 4) were analyzed by fluorescence microscopy in SD-Ura-Met medium. Histogram shows percentage of cells with Wss1 foci in TDP1 (n = 220) and tdp1Δ (n = 342) cells. Scale bar is 2 µm. (B) Top1-induced stress in tdp1Δ cells induces accumulation of Wss1-GFP in vacuole. Localization of Wss1 protein was studied in cells expressing Wss1-GFP from endogenous loci (MBY24, MBY46, and MBY47 strains, Supplementary file 3). In pGAL-MBP-TOP1 cells TOP1 promoter was replaced by GAL promoter and Top1 was expressed as MBP-Top1 fusion. Top panels show pGAL-MBP-TOP1 WSS1-GFP tdp1Δ cells (MBY47) upon induction of MBP-Top1 expression in galactose medium. Vacuole was stained with FM4-64 dye. Scale bar is 2 µm. The table shows mean GFP fluorescence of parental (MBY24) and pGAL-MBP-TOP1 cells (MBY46, and MBY47) measured by FACS (n = 20,000). The cells were grown in glucose or galactose medium at 30°C.
-
Figure 10—source data 1
IF microscopy and FACS data for Wss1 expression in TDP1 and tdp1Δ cells.
- https://doi.org/10.7554/eLife.06763.030
-
Figure 10—source data 2
IF microscopy data for Wss1 localization upon DNA damage.
- https://doi.org/10.7554/eLife.06763.031
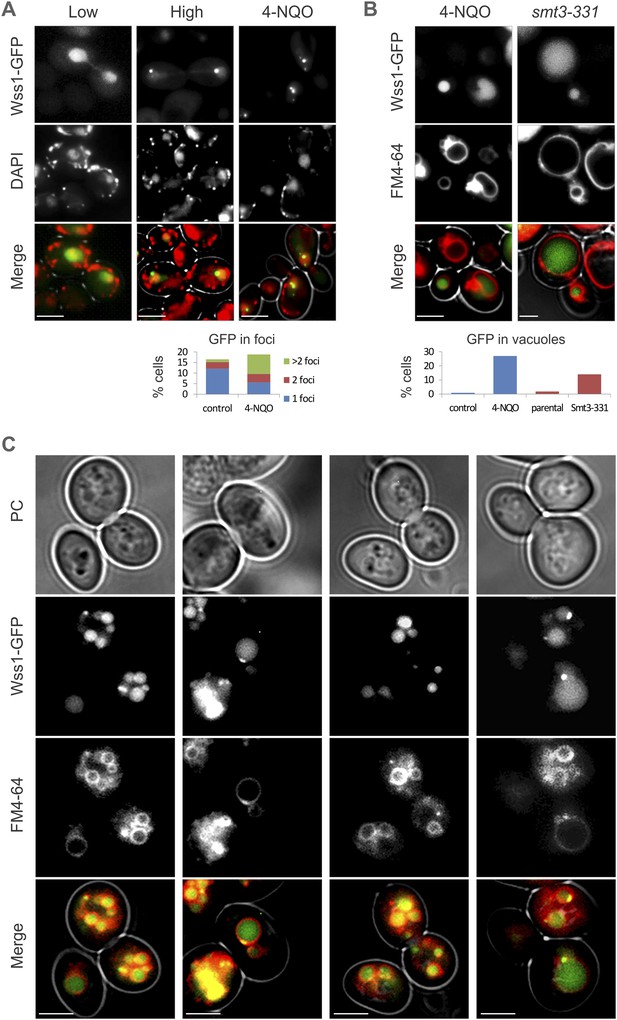
Wss1 localization upon DNA damage.
(A) Intracellular localization of Wss1. Wss1-GFP fusion protein was expressed from pUG35 plasmid under control of the inducible MET25 promoter. Live cells (BY4742) were stained with DAPI and analyzed by fluorescence microscopy. Low (SD-Ura medium) and high (SD-Ura-Met) level of expression conditions are shown. Right column shows cells pre-incubated with 0.2 µg/ml 4-NQO. Histogram shows percentage of cells containing Wss1 foci in control (n = 628) and 4-NQO-treated (n = 498) cells. Scale bar is 2 µm. (B) Cellular stress induces vacuolar accumulation of Wss1. Wss1-GFP fusion protein was expressed in BY4742 and smt3-331cells from pUG35 plasmid. BY4742 cells were treated or not with 0.2 µg/ml 4-NQO for 3 hr. The cells were then stained with FM4-64 and analyzed by fluorescence microscopy. Histogram shows percentage of cells with vacuolar GFP signal in control (n = 638) and 4-NQO-treated (n = 513) BY4742 cells as well as in smt3-331 (n = 201, SBY331) cells and parental SMT3 strain (n = 316, SBY21). Scale bar is 2 µm. (C) Top1 overexpression induces accumulation of Wss1 in vacuole. The images show accumulation of Wss1-GFP in vacuole in pGAL-MBP-TOP1 WSS1-GFP tdp1Δ cells (MBY47 strain) after induction of MBP-Top1 protein in galactose medium. Live cells were stained with vacuolar marker FM4-64 and analyzed by fluorescence microscopy. All selected images show bright fluorescent spots within the cytoplasm and on the vacuolar membrane suggesting the activation of specific autophagic pathway. Scale bar is 2 µm. See also corresponding source files: Figure 10—source data 2.xlsx (for supplement A and B) and Figure 10—source data 1.xlsx (for supplement C).
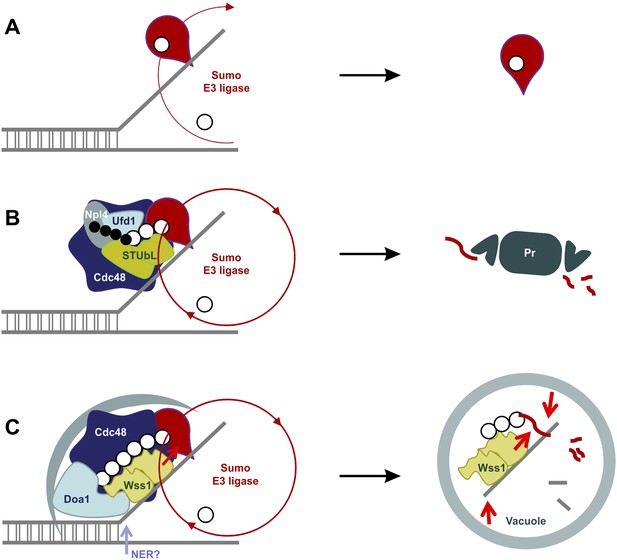
SUMO-dependent extraction of proteins from the chromatin.
(A) ssDNA-activated SUMO E3 ligase sumoylates DNA-bound protein and induces its dissociation. (B) Delay in dissociation results in SUMO chain formation through multiple rounds of protein sumoylation. Subsequent ubiqutylation by STUbL promotes Cdc48/Npl4/Ufd1 loading, protein extraction and degradation via proteasome. (C) When the extraction is compromised (e.g., covalent protein–DNA adduct), the protein is processed by Cdc48/Wss1/Doa1 complex. Wss1 is targeted to sumoylated protein via its SIMs and promotes extension of SUMO chain that in return could further stimulate Wss1 accumulation and oligomerization at the site of DNA damage (Wss1 foci). Binding to ssDNA and oligomerization triggers metalloprotease activity of Wss1 and initiates substrate processing. The process is assisted by Cdc48 and Doa1 and finally ends in the vacuole.
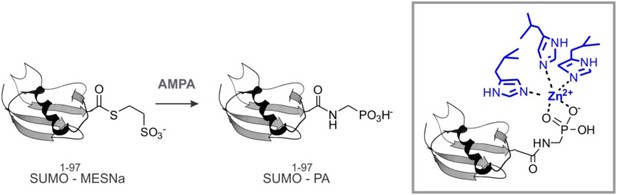
SUMO-PA and Ub-PA synthesis.
https://doi.org/10.7554/eLife.06763.044Additional files
-
Supplementary file 1
Wss1-interacting proteins identified by mass spectrometry.
- https://doi.org/10.7554/eLife.06763.034
-
Supplementary file 2
Doa1-interacting proteins identified by mass spectrometry.
- https://doi.org/10.7554/eLife.06763.035
-
Supplementary file 3
Yeast strains used in this study.
- https://doi.org/10.7554/eLife.06763.036
-
Supplementary file 4
Plasmids used in this study.
- https://doi.org/10.7554/eLife.06763.037
-
Supplementary file 5
Model of the WLM domain of Wss1. The structure of the WLM domain of Wss1 was modeled using the Phyre2 server.
- https://doi.org/10.7554/eLife.06763.038
-
Supplementary file 6
Model of the SprT domain of DVC1/Spartan. The structure of the SprT domain of DVC1/Spartan was modeled using Phyre2 server.
- https://doi.org/10.7554/eLife.06763.039
-
Supplementary file 7
Structure of abylysin (4JIU). This is a minigluzincin family metalloprotease used for modeling the WLM and SprT structures.
- https://doi.org/10.7554/eLife.06763.040
-
Supplementary file 8
Model of the Wss1 VIM peptide docked to Cdc48. The yeast N-Cdc48 domain (residues 23–186, magenta) was superimposed on 3TIW, the human P97 structure (residues 23–186, cyan) bound to the gp78 VIM (blue) using the align command in PyMOL. The PatchDock web server (Schneidman-Duhovny et al., 2005) was then used to perform the docking of the Wss1 VIM NMR structure (red) determined above (Figure 5—figure supplement 3A) to Cdc48. This is a pyMOL .pse file.
- https://doi.org/10.7554/eLife.06763.041