Dual recognition of multiple signals in bacterial outer membrane proteins enhances assembly and maintains membrane integrity
Figures
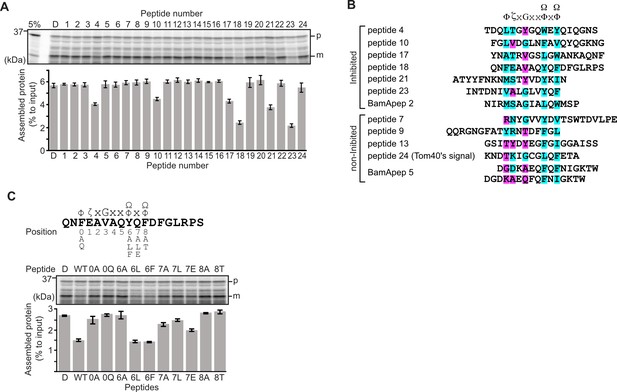
Peptides derived from β-strands of OmpC selectively inhibit outer membrane protein (OMP) assembly.
(A and C) 35S-labeled EspP was synthesized by rabbit reticulocyte lysate, then incubated with E. coli microsomal membrane (EMM), and samples prepared for analysis by SDS-PAGE and radio-imaging (see Materials and methods). EspP assembly into EMM was performed in the presence of DMSO (D) or specific inhibitor peptides. The precursor (p) and mature (m) form of EspP are indicated. (A) Peptides labeled 1 through 23 were derived from OmpC, while peptide 24 was taken from a mitochondrial β-barrel protein to serve as a negative control (see Figure 1—figure supplement 2). (B) Sequence comparison of inhibitor peptides identified in the EspP assembly assays. The signal comprises key hydrophobic (Φ), polar (ζ), glycine (G), and two aromatic (Ω) or hydrophobic amino acids. Amino acids highlighted in blue conform to the consensus signal, while those highlighted in purple deviate from the consensus signal. (C) Upper: sequence of the peptide 18 indicating residue codes for the mutated peptides, e.g., at position ‘6’, the native residue Y was mutated to A in peptide ‘6A’. Position 0 designates the first hydrophobic residue and position 8 designates the final aromatic or hydrophobic residue. DMSO (D) was used for control. Middle: SDS-PAGE of EspP assembly assay. Lower: data for inhibition of EspP assembly into EMM assayed in the presence of each of the indicated peptides.
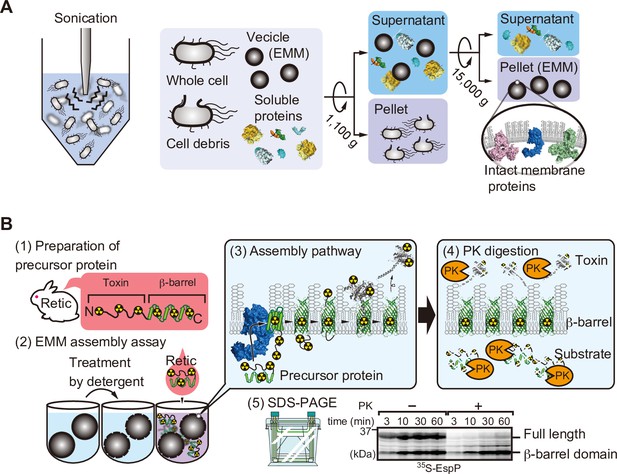
Schematic of E. coli microsomal membrane (EMM) isolation and EMM assembly assay.
(A) EMMs were isolated via cell disruption by sonication. Cell debris is removed via low-speed centrifugation, finally membranes are harvested after high-speed centrifugation. (B) 35S-labeled EspP EMM assembly is performed as follows: (1) Radiolabeled substrate proteins are translated using rabbit reticulocytes lysate, (2) EMMs are treated with detergent then radio-labeled substrate is added to the EMM. (3) Substrate and β-barrel assembly machinery (BAM) complex interacts with and assists the insertion of substrate into EMM. (4) EMMs were then incubated with protease. Unassembled EspP, as well as the passenger domain, are digested, only membrane-integrated barrel domain is protected from the protease. (5) Samples are boiled and subjected to SDS-PAGE for analysis.
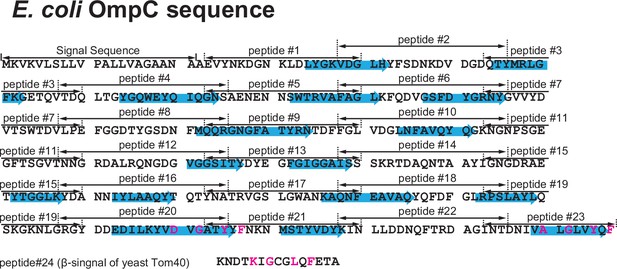
Design of peptide inhibitor library from the E. coli outer membrane protein (OMP) OmpC.
Primary sequence of the E. coli OMP OmpC divided into peptide regions. The sequence was divided into 23 peptides made up of 15 amino acids. Highlighted in the blue arrows are the 16 β-sheets which make up OmpC. Below OmpC sequence, the canonical β-signal of yeast-Tom40, a mitochondrial β-barrel protein, used as peptide #24 control.
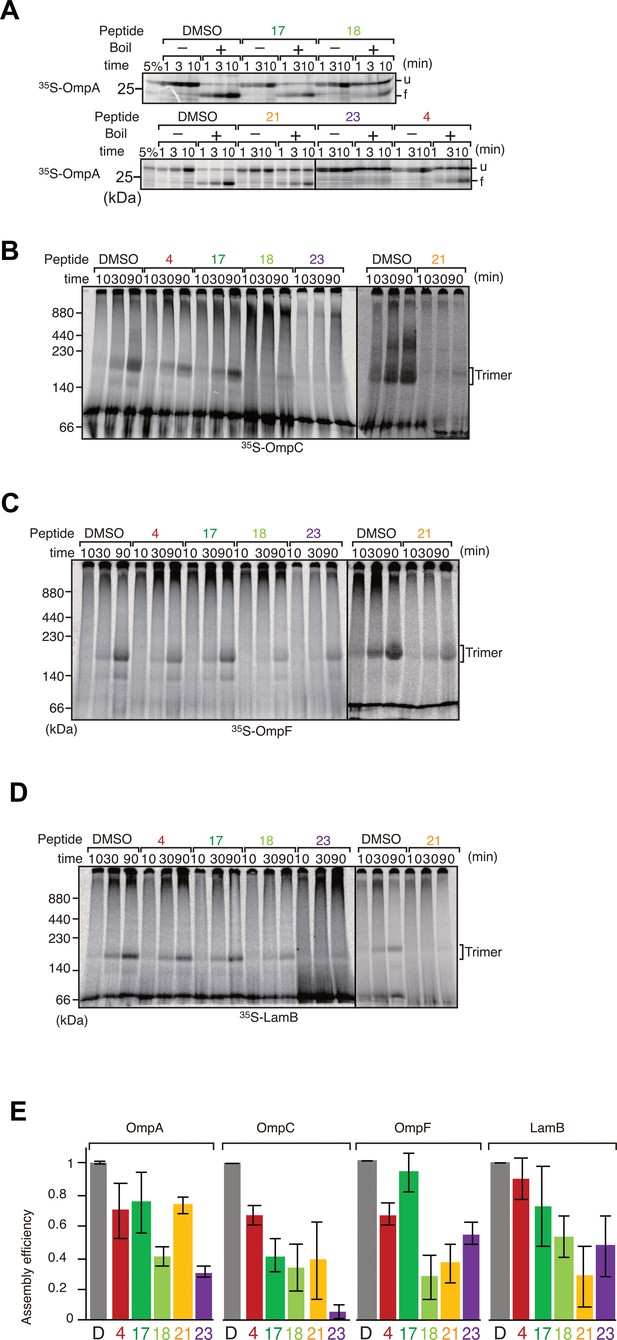
Peptide inhibition of multiple β-barrel assembly machinery (BAM) complex substrate assembly.
(A) The eight β-strand outer membrane protein (OMP), OmpA, was incubated with E. coli microsomal membrane (EMM) then shifted onto ice to halt the assembly reaction at indicated times. Samples were split and incubated at either 25°C or 99°C for 10 min, before analysis by SDS-PAGE and radio-imaging. (u) and (f) indicate unfolded and folded, respectively. (B–D) Peptide inhibition of assembly reactions for OmpC (B), OmpF (C), and LamB (D). 35S-labeled proteins were incubated as above for indicated time, shifted onto ice, and solubilized in Blue native (BN)-PAGE lysis buffer containing 1.5% DDM. Solutions were clarified via centrifugation and analyzed by BN-PAGE and radio-imaging. The assembled, trimeric form of each porin is indicated. (E) Densitometry data for the endpoints of the assays on all assembled proteins (D–G), from three independent experiments.
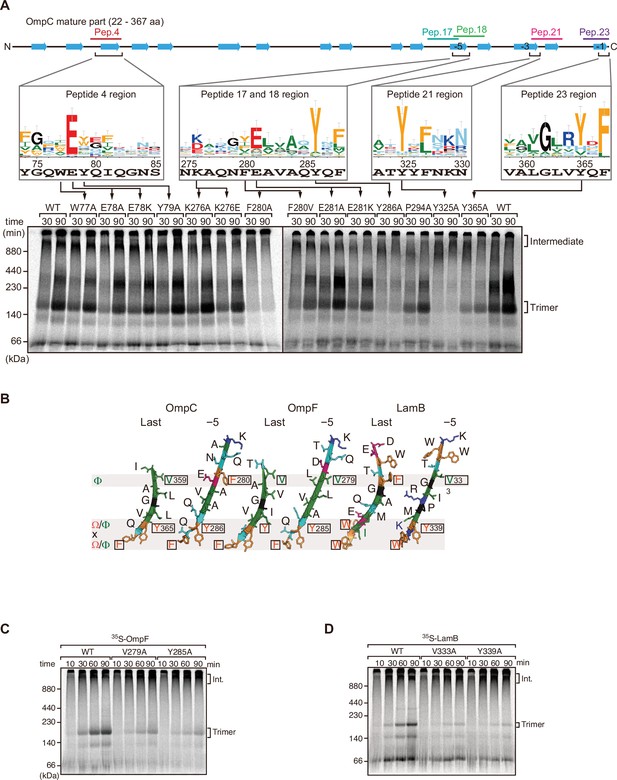
Mutations in β-signals inhibit outer membrane protein (OMP) assembly in vitro.
(A) Representation of OmpC (upper panel) annotated to show the position of each β-strand (blue arrows) and the region mimicked in the indicated peptides. Sequence logos show the conservation of sequence for the residues in these regions (see Materials and methods), where the height of letter corresponds to the degree of conservation across bacterial species, residues are color-coded: aromatic (orange), hydrophobic (green), basic (blue), acidic (red), polar (sky blue), and proline and glycine (black). In the lower panel, data from the E. coli microsomal membrane (EMM) assembly assay of OmpC mutants is organized to show effects for key mutants: 35S-labeled OmpC wild-type or mutant proteins were incubated with EMM for 30 or 90 min, and analyzed by Blue native (BN)-PAGE and radio-imaging. (B) Comparison of amino acids in the final and fifth to final (–5) β-strands of OmpC, OmpF, and LamB. All three OMPs contain the putative hydrophobic residue near the N-terminal region of the β-strands, highlight with a black box. A hydrophobic and an aromatic residue is also found to be highly conserved between these OMP β-strands at the C-terminal amino acids of the β-strands. (C–D) Assembly of 35S-labeled OmpF (C) or LamB (D) with mutations to the –5 β-strand or final β-strand into EMM. Samples were prepared as in (A).

Mutations to internal β-signal-like motif results in loss of assembly in trimeric β-barrel assembly machinery (BAM) complex substrate, OmpC.
(A) Assembly of indicated BAM complex substrates with mutations to the –5 β-strand or final β-strand. Substrates were incubated with E. coli microsomal membrane (EMM) for 10, 30, or 90 min, then analyzed by Blue native (BN)-PAGE and radio-imaging. Int. indicates high-molecular-weight intermediate form.
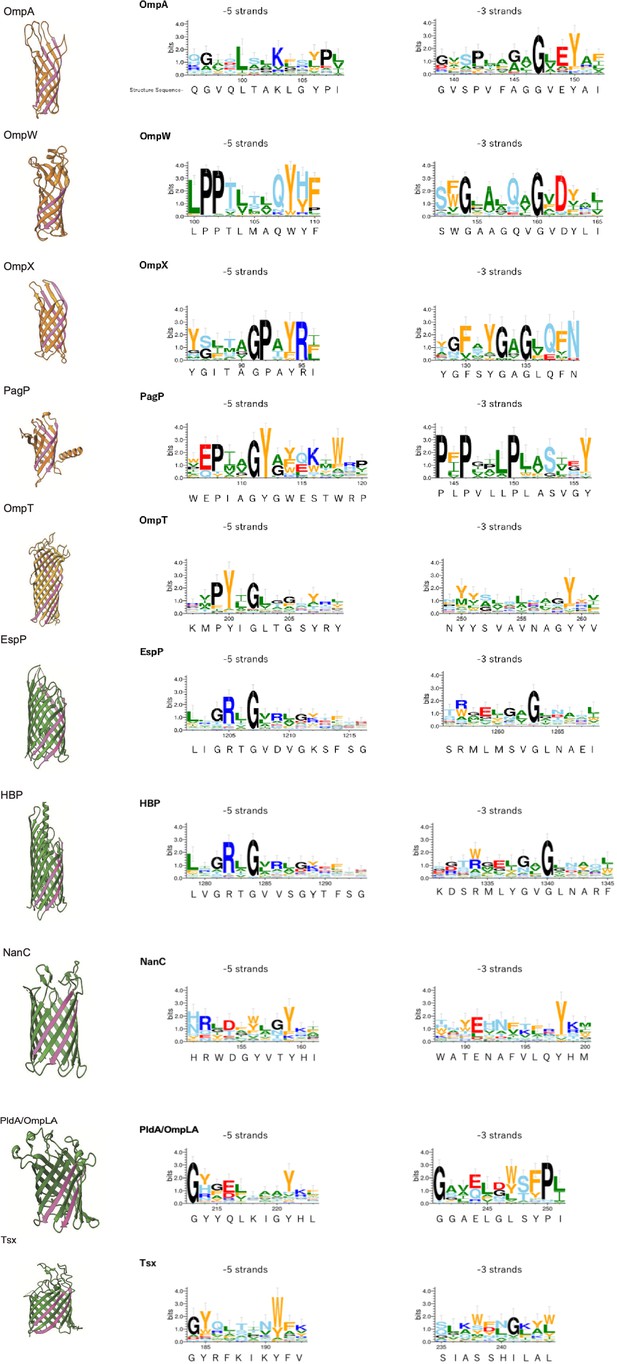
Multiple alignment of –5 β-strand of outer membrane proteins (OMPs) with 8, 10, or 12 β-strands.
Sequence logos –5 and –3 β-strand were generated using WebLogo 3. Sequence from structure used is shown below the WebLogo. Aromatic, hydrophobic, positively charged, negatively charged, hydrophilic, and secondary structure breaker residues (P, G) are shown by orange, green, blue, red, sky blue and black, respectively. The –5 and –3 strands are highlighted in pink. PDB access codes of proteins are as follows: 8 β-strand; OmpA (1BXW), OmpW (2F1T), OmpX (1QJ8), PagP (3GP6), 10-strand; OmpT (1I78), 12 β-strand; EspP (2QOM), HBP (3AEH), NanC (2WJQ), PldaA/OmpLA (1QD5), Tsx (1TLW).
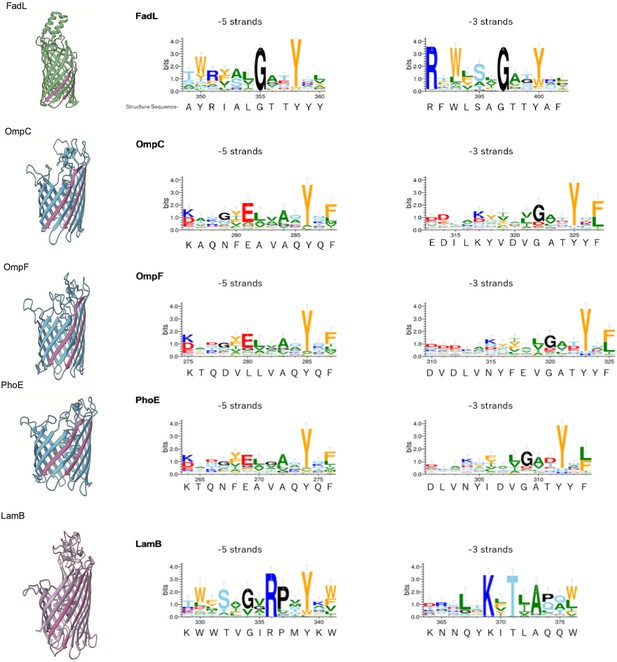
Multiple alignment of –5 β-strand of outer membrane proteins (OMPs) with 14, 16, or 18 β-strands.
Sequence logos of –5 and –3 β-strand were generated and colored as explained in Figure 2—figure supplement 2. PDB access codes of proteins are as follows: 14 β-strand; FadL (1T16) 16 β-strand; OmpC (2J1N), OmpF (1BT9), PhoE (1PHO), 18 β-strand; LamB (1MPM).
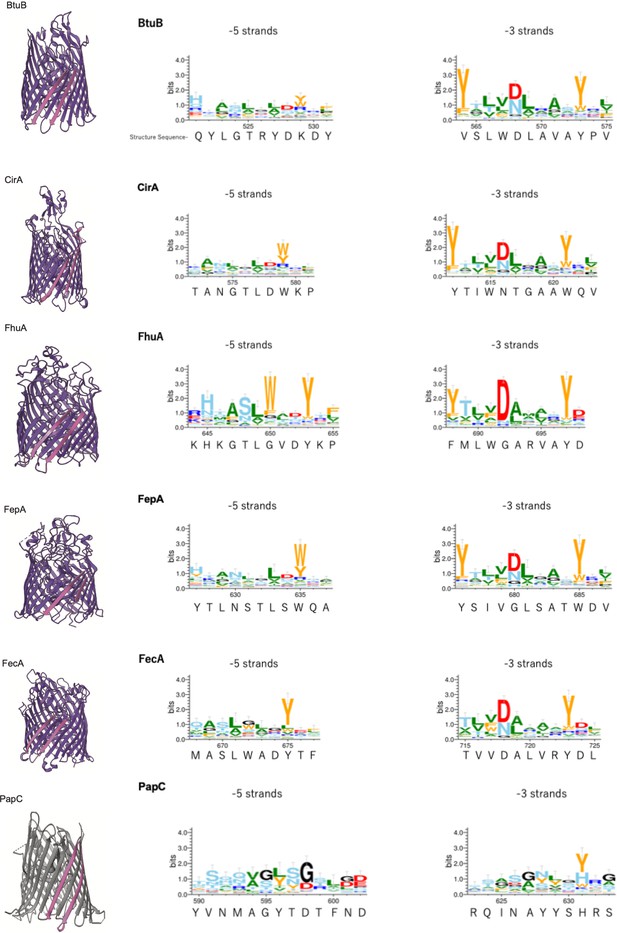
Multiple alignment of –5 β-strand of outer membrane proteins (OMPs) with 22 or 24 β-strands.
Sequence logos of –5 and –3 β-strand were generated and colored as explained in Figure 2—figure supplement 2. PDB access codes of proteins are as follows: 22 β-strand; BtuB (1NQE), CirA (2HDI), FhuA (1BY3), FepA (1FEP), FecA (1KMO), 24 β-strand; PapC (2VQI).
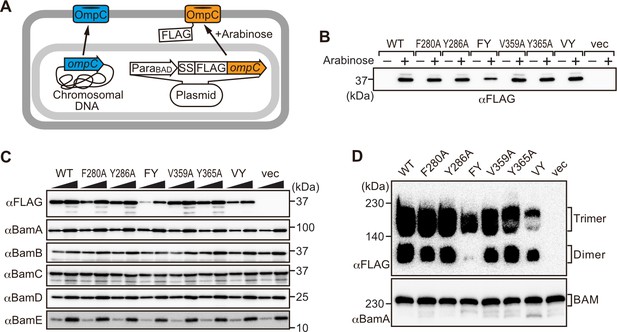
Modifications in the putative internal signal slow the rate of outer membrane protein (OMP) assembly in vivo.
(A) Schematic model of FLAG-OmpC expression system in E. coli. The plasmid-borne copy of OmpC was mutated and the mutant proteins are selectively detected with antibodies to the FLAG epitope. (B) Total cell lysates were prepared from the variant E. coli strains expressing FLAG-OmpC(WT), –5 strand mutants: F280A, Y286A, double mutant F280A/Y286A (FY), or final β-strand mutants: V359A, Y365A, double mutant V359A/Y365A (VY) with (+) or without (-) arabinose. The steady-state levels of the indicated proteins were assessed after SDS-PAGE by immunoblotting with anti-FLAG antibody. (C) After growth with arabinose induction, E. coli microsomal membrane (EMM) fraction was isolated from each of the FLAG-OmpC variant expressing E. coli strains to assess steady-state levels of OmpC variants and β-barrel assembly machinery (BAM) complex subunits: BamA, BamB, BamC, BamD, and BamE by SDS-PAGE and immunoblotting. Triangles indicate the total protein amount of EMM in each sample: Left; 5 μg, right; 15 μg. (D) EMM fractions were solubilized with 1.5% DDM and subjected to Blue native (BN)-PAGE and immunoblotting. The indicated proteins were detected by anti-FLAG (top) and anti-BamA antibodies (bottom), respectively.
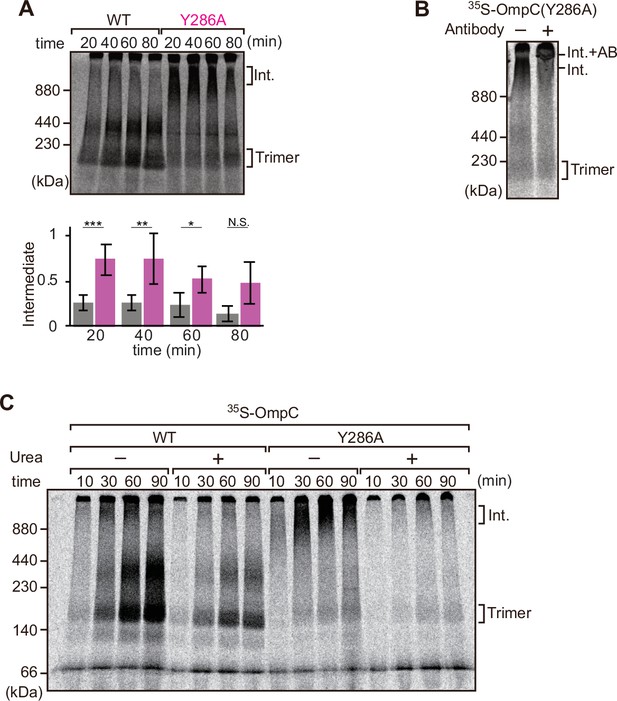
Mutation that stalls outer membrane protein (OMP) assembly in a high-molecular-weight assembly intermediate.
(A) Upper panel, assembly assay of 35S-OmpC wild-type (WT) and mutant Y286A. After incubation in the E. coli microsomal membrane (EMM) assays for indicated time points, 35S-proteins were resolved via Blue native (BN)-PAGE. OmpC trimer and intermediate (int) are indicated. The lower panel shows a quantification of the intermediate form compared to total assembled protein (average of three independent experiments). Statistical significance was indicated by the following: N.S. (not significant), p<0.05; *, p<0.005; **, p<0.001; ***. Exact p values of intermediate formed by WT vs Y286A at each time point were as follows: 20 min: p=0.00101, 40 min: p=0.006, 60 min: p=0.0371, 80 min: p=0.1283. (B) 35S-labeled OmpC(Y286A) was incubated with EMM for 60 min, then the EMM fraction was solubilized with 1.5% DDM and incubated with (+) or without (-) anti-BamC antibody for 40 min. These samples were then analyzed by BN-PAGE. The presumptive translocation intermediate (Int) and the gel shift species (Int. +AB) are indicated. (C) 35S-labeled OmpC was incubated with EMM and then analyzed by BN-PAGE and radio-imaging. EMMs were then washed and solubilized in the presence (+) or absence (-) of 6 M urea, after which the membrane fraction was re-isolated via ultracentrifugation for 45 min. Isolated membranes were solubilized in 1.5% DDM and analyzed by BN-PAGE.

In vitro folding of OmpC β-signal mutants into detergent micelles.
(A) Aromatic residues at the position C-terminus, not the 0Φ position, of the β-signal influence the folding rate of OmpC in vitro. Urea-denatured OmpC variants were folded into DDM-micelles in 10 mM phosphate buffer (pH 8.0), 2 mM EDTA, and 1 mM glycine. Samples were incubated at 37°C for up to 24 hr then split with half the samples (B), boiled and half at 25°C. OmpC showed three individual species, a high-molecular-weight assembled trimer, a middle-molecular-weight dimer, and monomeric unfolded OmpC.
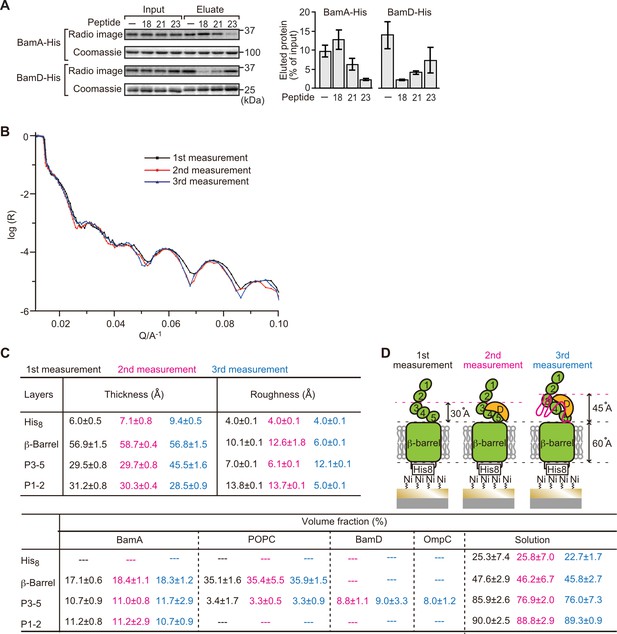
Proximity and dependence of BamD with OmpC(Y286A) revealed by neutron reflectometry (NR) analysis.
(A) Ni-NTA was used to affinity purify His6-BamA or His6-BamD. The protein-Ni-NTA bead complex was then incubated in the presence of DMSO or the indicated peptides resuspended in DMSO, and 35S-labeled OmpC was incubated with these beads. After washing, bound proteins were eluted with 400 mM imidazole buffer. The graph represents densitometry analysis of data from three independent experiments. (B) NR profiles for BamA in membrane (first measurement, square black line), after addition of BamD (second measurement, circle red line), and after addition of the OmpC(Y286A) substrate (third measurement, triangle blue line). The experimental data was fitted using a seven-layer model: chromium - gold - NTA - His8 - β-barrel - P3-5 - P1-2. (C) Table summaries of the thickness, roughness, and volume fraction data of each layer from the NR analysis. The thickness refers to the depth of layered structures being studied as measured in Å. The roughness refers to the irregularities in the surface of the layered structures being studied as measured in Å. First, second, and third measurement displayed in black, magenta, and blue, respectively. P3-5: POTRA3, POTRA4, and POTRA5, P1-2: POTRA1 and POTRA2, lipid: POPC, solution: D2O, gold match water (GMW), and H2O. Details were described in Supplementary file 8 to Supplementary file 10. (D) NR schematic of BamA (green), BamD (yellow), and OmpC(Y286A) (magenta). Numbers indicate corresponding POTRA domains of BamA. Note that the cartoon is a depiction of the results and not a scale drawing of the structures.
-
Figure 5—source data 1
NR data of the first mesurement.
- https://cdn.elifesciences.org/articles/90274/elife-90274-fig5-data1-v1.zip
-
Figure 5—source data 2
NR data of the second mesurement.
- https://cdn.elifesciences.org/articles/90274/elife-90274-fig5-data2-v1.zip
-
Figure 5—source data 3
NR data of the third mesurement.
- https://cdn.elifesciences.org/articles/90274/elife-90274-fig5-data3-v1.zip
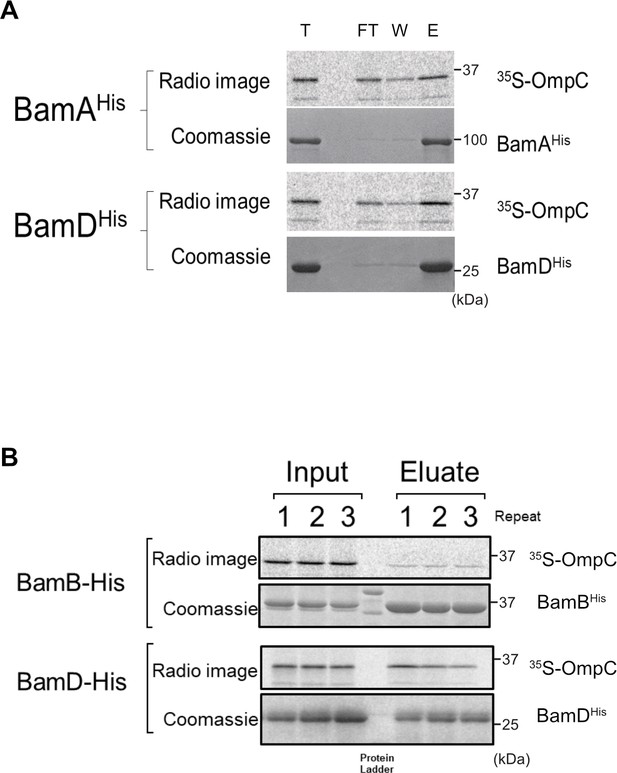
Ni-NTA pull-down of 35S-OmpC with purified β-barrel assembly machinery (BAM) complex substrate-binding subunits.
(A) 35S-OmpC was incubated with purified BamA (top) or BamD (bottom). Samples were rocked for 60 min at 4°C. Ni-NTA was added and samples were incubated at 4°C for an additional 30 min. Ni-NTA was pelleted by low-speed centrifugation, flow through (FT) was collected and Ni-NTA was washed (W) with Buffer A containing 20 mM imidazole. BAM protein and substrate were eluted (E) with Buffer A and 400 mM imidazole. Proteins in FT, W, and E were precipitated using trichloroacetic acid, washed with acetone. Protein pellets were resuspended in SDS loading dye, boiled, and resolved using SDS-PAGE. (B) 35S-OmpC Ni-NTA was incubated with BAM complex lipoproteins proposed to be associated with substrate binding, BamB (top) and BamD (bottom). Experiment was performed as in (A), numbers above indicate replicate number.
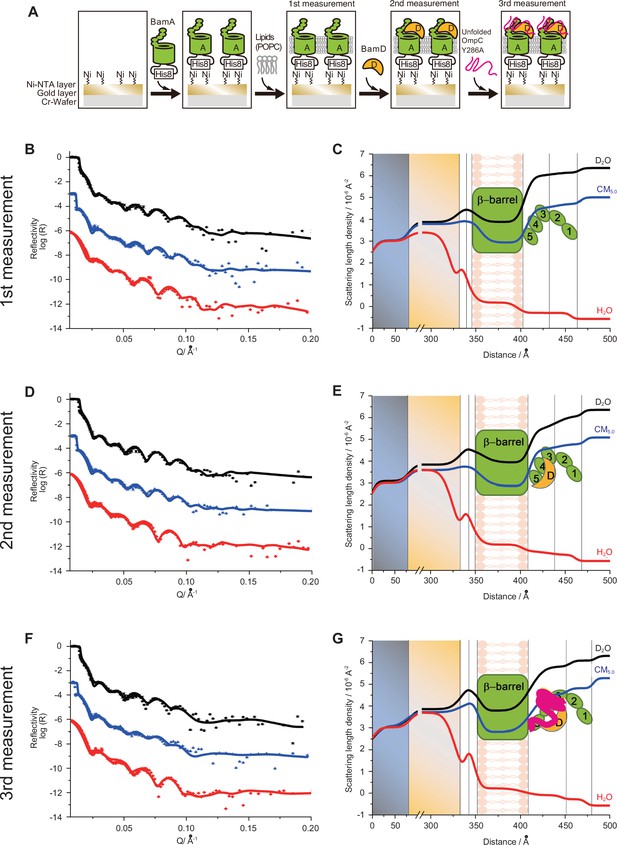
Characterization of BamA-POPC, BamA-POPC BamD, and BamA-POPC-BamD-OmpC (Y286A) interaction via neutron reflectometry (NR).
(A) Flowchart of NR experiment. Chromium (Cr) and gold-coated silicon wafers were initially treated with Ni-NTA followed by the addition of purified His6-BamA. Lipid membrane, POPC, was added for the first measurement, after which BamD was incubated for 30 min for the second measurement. Finally, unfolded OmpCY286A was added to the wafer for the third measurement. (B, D, F) The neutron reflectometry profiles (symbols) and fits (lines) for BamA in the POPC bilayer (B), the addition of BamD in the BamA-POPC (D), and the addition of OmpC in the BamA-POPC-BamD complex (F) under D2O (black line), GMW (blue line), and H2O (red line) contrasts. (C, E, G) The real space nSLD profiles for BamA in the POPC bilayer (C), the addition of BamD in the BamA-POPC (E), and the addition of OmpC in the BamAPOPC-BamD complex (G) under D2O (black line), GMW (blue line), and H2O (red line) contrasts. (D–E) The addition of BamD will not change the conformation of BamA and the volume fraction of BamD is found to be around 8.8% in P3-5 layer. (F–G) The further addition of OmpC (Y286A) will not change the conformation of BamAD complex and OmpC was located in the P3-5 layer with volume fraction of 8.0%.

Characterization of BamA-POPC and BamA-POPC-BamD complex formation via neutron reflectometry (NR).
( A) NR profiles for BamA in membrane (first measurement, black line), BamAD (second measurement, red line), and BamAD-OmpC (WT) (third measurement, blue line). (B, D) The NR profiles (symbols) and fits (lines) for BamA in the POPC bilayer (B) and the addition of BamD in the BamA-POPC (D) under D2O (black line) and GMW (blue line) contrasts. (C, E) The real space nSLD profiles for BamA in the POPC bilayer (C) and the addition of BamD in the BamA-POPC (E) under D2O (black line), GMW (blue line), and H2O (red line) contrasts. (D, E) The addition of BamD did not change the conformation of BamA and the volume fraction of BamD was found to be around 8.8% in P3-5 layer. (F) Table summary of thickness, volume fraction, and roughness determined during the NR analysis. First and second displayed in black and magenta, respectively. P3-5: POTRA3, POTRA4, and POTRA5, P1-2: POTRA1 and POTRA2, lipid: POPC, solution: D2O and gold match water (GMW).
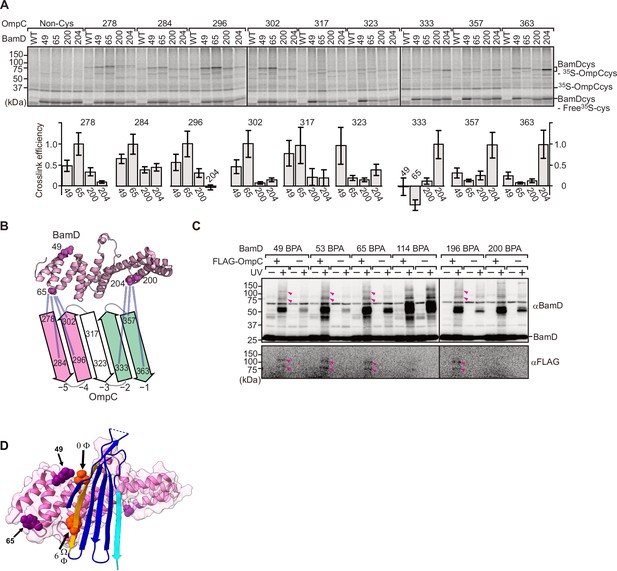
BamD acts as the receptor for internal β-signals.
(A) BamD-cys mutant proteins were purified and incubated with 35S-OmpC with cysteine mutations from the –5 to the final β-strand. After the addition of the oxidizing agent, CuSO4, samples were purified with Ni-NTA and analyzed by SDS-PAGE. (B) BamD cysteine mutants, highlighted in magenta, formed disulfide bonds at distinct locations of OmpC. (C) p-Benzoyl-L-phenylalanine (BPA) containing BamD was expressed in the presence (+) or absence (-) of FLAG-OmpC overexpression. Pink arrows indicate BamD-FLAG-OmpC cross-link products. (D) Outer membrane protein (OMP) substrate superimposed on BamD. BamD cross-linking positions corresponding to internal signal are marked in purple and substrate cross-linking regions are marked in orange.
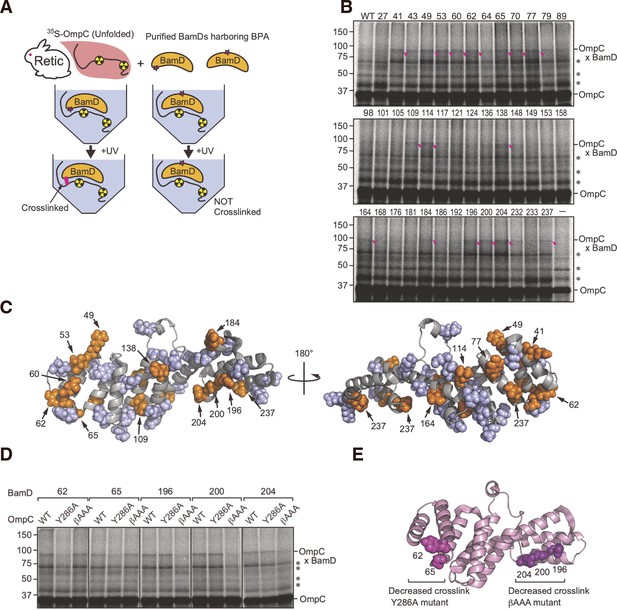
In vitro p-benzoyl-L-phenylalanine (BPA) cross-link analysis of substrate-binding regions within BamD.
(A) Schematic of in vitro cross-linking of purified BamD and 35S-labeled OmpC. (B) BamD expressing unnatural amino acids was purified, incubated with purified OmpC substrate, UV-irradiated, and analyzed by SDS-PAGE. Numbers above each lane indicate the amino acid position that was mutated to BPA. Pink arrows designate the cross-link products of BamD-OmpC, while asterisks signify non-specific cross-link products. (C) Summary of cross-link study in (B) on the structure of BamD. Positions highlighted in orange spheres indicate amino acid positions of BPA incorporation that cross-linked with OmpC, while positions which did not cross-link are highlighted in violet spheres. (D) Comparison cross-linking products of OmpC WT, Y286A, and β-AAA (final β-strand) signal mutant and BamD. OmpC-BamD cross-link products are highlighted with a dash and non-specific cross-link products are as above. Experiment was performed as in (B, E) Highlighted sites of BamD which showed decreased cross-linking products in OmpC signal mutations compared to WT. Amino acids that showed decreased cross-linking with OmpC Y286A are shown in magenta (BamD 62 and 65), decreased cross-linking with OmpC β-AAA are shown in dark purple (BamD 196, 200, 204).
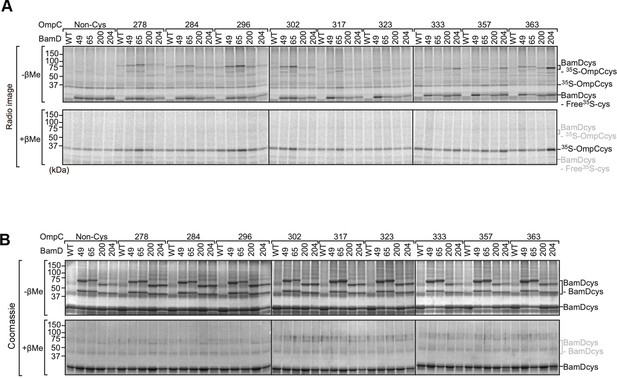
Analysis of the interaction between BamD and OmpC by intermolecular disulfide cross-linking.
(A) Purified BamDcys mutant proteins were incubated with 35S-OmpC with cysteine mutations from the –5 to the final β-strand. After the addition of CuSO4 samples were purified with Ni-NTA and analyzed by SDS-PAGE and radio-imaging. Samples of the bottom gels were prepared in the presence of the reducing agent (β-mercaptoethanol [β-Me]). (B) Coomassie-stained gels from (A).
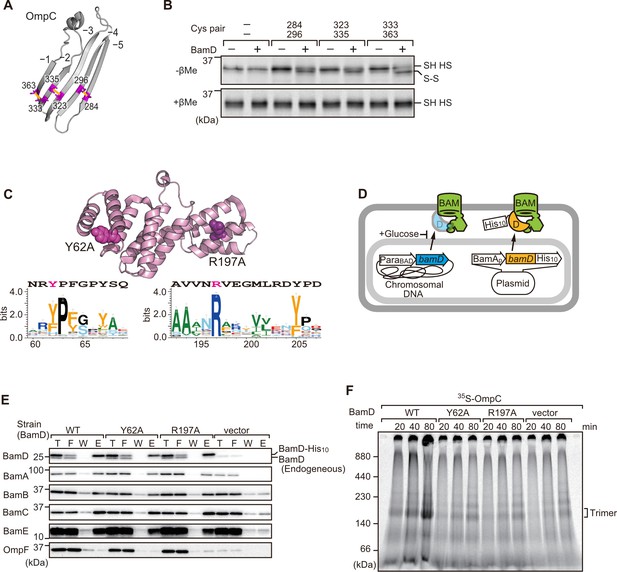
Key residues in two structurally distinct regions of BamD promote β-strand formation and outer membrane protein (OMP) assembly.
(A) Double cysteine residues were mutated into OmpC between anti-parallel β-strands to form artificial disulfide bonds. (B) 35S-OmpC-cys variants were translated then incubated with (+) or without (-) BamD. The samples were analyzed by reducing or non-reducing condition. Disulfide bond-specific bands (S-S) are indicated to the right of the gel. (C) Sequence conservation analysis of BamD. Target residues, Y62 and R197, are indicated with dark pink spheres on the crystal structure of BamD, sequence logos represent conservation in the immediate region of these residues. Amino acids listed above logo sequence are from crystal structure. Sequence conservation of BamD (bottom). (D) Schematic depiction of the BamD depletion strain of E. coli used to express mutant BamD proteins. (E) Pull-down assay of the variants of BamD-His8. The E. coli microsomal membrane (EMMs) as in (C) were solubilized with 1.5% DDM and then subjected to Ni-NTA. T - 5% total input, F - unbound fraction, W - wash fraction, E - eluted fraction. Each fraction was analyzed by SDS-PAGE and immunoblotting against indicated antibodies. (F) Assembly of OmpC was reduced in EMMs containing BamD mutated at Y62 and R197. 35S-labeled OmpC was incubated with EMM as in (C), and then analyzed by Blue native (BN)-PAGE and radio-imaging.
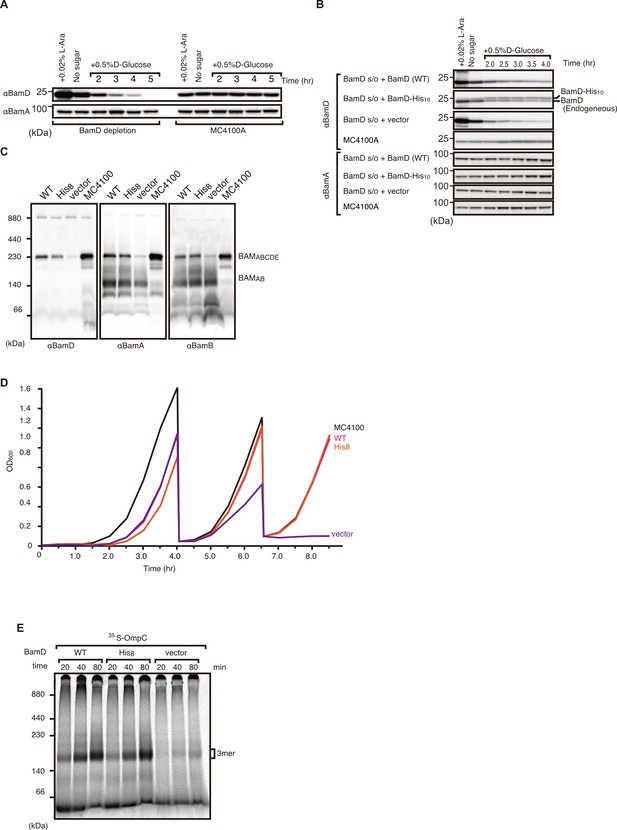
Validation and characterization of BamD depletion strain.
(A) Optimization of BamD depletion as compared to parent strain MC4100A. BamD levels were monitored by immunoblotting after growth in media containing glucose to repress chromosomal BamD expression as shown in Figure 6E. (B) Expression of plasmid-borne BamD and BamD harboring poly-histidine tag versus empty vector and MC4100A strain. Both tagged and non-tagged BamD were detected at similar levels. (C) BamA expression remained unchanged between all samples. Blue native (BN)-PAGE western blot analysis of β-barrel assembly machinery (BAM) complex formation in BamD depletion strain. Expression of non-tagged and His-tagged BamD did not affect BAM complex formation. BamD-depleted cells were unable to from full BAM complex (BamABCDE), but did form a smaller BamAB complex. (D) Growth curve of BamD depletion strain expressing His-tagged and non-tagged BamD as followed by optical density at 600 nm. Cells were grown in non-permissive conditions (+ glucose) and were sub-cultured into fresh LB media containing glucose two times, after which BamD depletion strain was incapable of growing. (E) Assembly of 35S-labeled OmpC in BamD depletion strain expressing plasmid-borne tagged and non-tagged BamD via E. coli microsomal membrane (EMM) assembly assay. OmpC was able to be assembled by both tagged and non-tagged BamD, while BamD-depleted cells were unable to assemble into OmpC into EMMs.
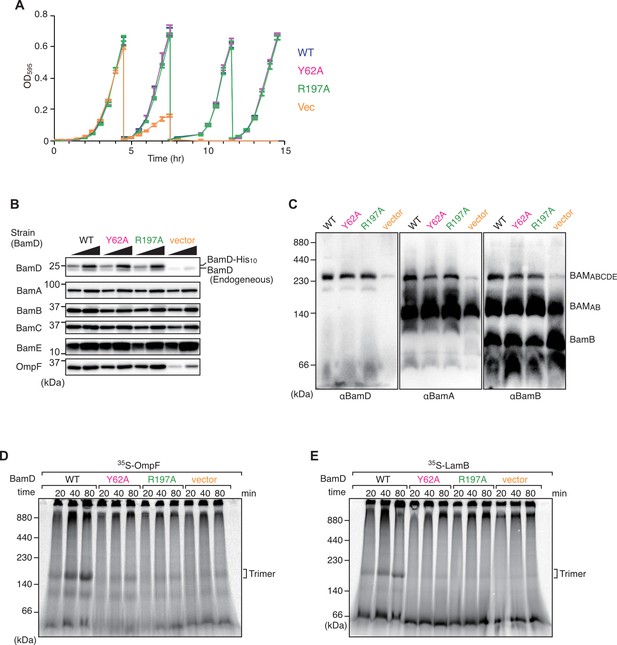
Effect of BamD mutations on cell growth and β-barrel assembly machinery (BAM) complex formation in vivo and in vitro assembly ability.
(A) Growth curve of BamD depletion strain expressing BamD and mutant strains from plasmids. Cells were grown in glucose-containing media to repress endogenous BamD expression. WT: blue line, Y62A: magenta, R197A: green, and empty vector: orange. (B) Steady-state levels of indicated proteins in E. coli microsomal membrane (EMM) isolated from optimized time cultured bamD shut-down strains. BAM complex proteins showed no difference in steady-state levels, while the outer membrane protein (OMP), OmpF, showed a decrease in empty vector. (C) Blue native (BN)-PAGE western blot analysis of BAM complex formation in BamD depletion expressing mutant BamD. Both BamD mutations were able to form full BAM complex. (D–E) EMM assembly assay of BamD variants using OmpF (D) or LamB (E) as substrates, respectively. Assembly of both substrates was impaired in EMM containing BamD mutations.

Schematic model of BamD depletion strain construction.
Indicated four DNA fragments were amplified respectively, and then combined by overlap PCR method. The PCR fragment was introduced into MC4100A strain harboring pKD46 plasmid. Final chromosomal DNA map is shown at the bottom.
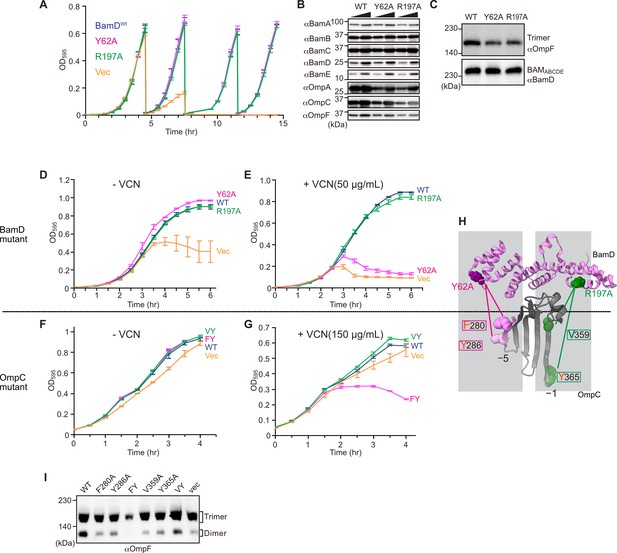
Substrate recognition by BamD is essential to maintain membrane integrity.
(A) Strains of E. coli with a depletion of chromosomal bamD, complemented by expression of the indicated forms of BamD, were grown in restrictive media (LB supplemented with 0.5% glucose) for 4 hours, and then cultures were diluted into freash same media every 4 hours and measured growth curve. (B) Quantitation of steady-state protein levels of OmpF and the indicated subunits of the β-barrel assembly machinery (BAM) complex in WT, Y62A, R197A mutants were determined after SDS-PAGE and immunoblot analysis. Wedge indicates that in each case, two samples were assessed corresponding to either 4 or 12 μg of total protein. (C) Quantitation of the level of trimeric OmpF formed in the indicated E. coli microsomal membrane (EMM) assays. The immunoblot analysis is shown after Blue native (BN)-PAGE analysis and immunoblotting with antibodies recognizing OmpF (upper panel) or BamD (lower panel). (D, E) E. coli bamD depletion cells expressing mutations at residues, Y62A and R197A, in the β-signal recognition regions of BamD were grown in the presence of VCN. (F, G) E. coli cells expressing mutations to OmpC internal signal, as shown in Figure 3, grown in the presence of VCN. Mutations to two key residues of the internal signal were sensitive to the presence of VCN. (H) Structure of BamD (top) and the final five β-strands of OmpC (bottom). Gray boxes indicate relative regions of interaction between BamD and substrates. (I) OmpF complex assembly in vivo of cells expressing mutant OmpC. OmpF assembly was greatly reduced in cells expressing double mutations to the –5 internal β-signal.
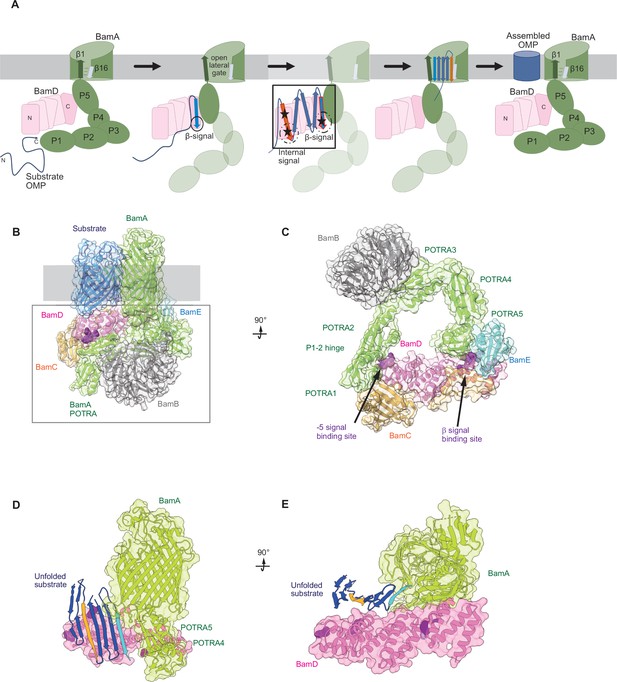
Model for the recognition of internal and canonical β-signals by the β-barrel assembly machinery (BAM) complex.
(A) BAM complex-assisted outer membrane protein (OMP) assembly is initiated when substrate OMPs encounter the periplasmic domain of the BAM complex. The canonical β-signal (blue arrow) of the OMP engages with the C-terminal TPR motifs of BamD (pink), after which the internal β-signal (orange arrow) is marshalled by the N-terminal TPR motif. The internal signal interacts with BamD R49 and N60 (black stars) while the canonical β-signal interacts with BamD D204 (black star). The proximity of BamD substrate to POTRA5 and the organization of the C-terminal β-strands of OMPs can then stimulate the entrance to the lateral gate of BamA. Finally, barrel formation and release into the membrane follows as previously published. (B) Structure of substrate engaged BAM complex (PDBID: 6V05). (C) Bottom-up view of periplasmic domain of complex as shown in (B). Arrows indicate the position of the residues on BamD that function in binding sites for –5 signal and the β-signal. (D) A cavity formed by POTRA1-2 and POTRA5 of BamA and BamD is sufficient to accommodate the C-terminal five β-strands of substrate OMP. Substrates –1 strand and –5 β-strand are indicated by light blue strand and orange strand, respectively. The signal-binding region of BamD is emphasized by deep purple (PDBID; Bam complex: 7TT5 and 7TT2). (E) Bottom-up view of complex as shown in (D).

Pull down assay of radio-labelled OmpC with indicated protein or Ni-NTA alone (Ni-NTA) .
T; total, FT; Flow throw, W; wash, E; Elute.

Experimental (symbols) and fitted (curves) NR profiles of BamA not oriented well in the POPC bilayer in D2O (black), GMW (blue) and H2O (red) buffer.

Experimental (symbols) and fitted (curves) NR profiles of BamA+D embedding together in the POPC bilayer in D2O (black), GMW (blue) and H2O (red) buffer.
Tables
Reagent type (species) or resource | Designation | Source or reference | Identifiers | Additional information |
---|---|---|---|---|
Gene (Escherichia coli) | Mg1655 | Lithgow lab | Template for cloning | |
Strain, strain background (Escherichia coli) | BL21(DE3)* | Invitrogen | Protein expression | |
Strain, strain background (Escherichia coli) | bamD depletion | This paper | bamD depletion | For analysis of bamD function |
Recombinant DNA reagent | pTnT-f (primer) | Thewasano et al., 2023 | PCR primers for in vitro transcription/translation | ACTTAATA CGACTCAC TATAGGCT A |
Recombinant DNA reagent | pTnT-r (primer) | Thewasano et al., 2023 | PCR primer for in vitro transcription/translation | GGATCCAA AAAACCCC TCAAGACC C |
Recombinant DNA reagent | pET22-BamAL4H8 (Plasmid) | Ding et al., 2020 | For protein expression for NR | |
Recombinant DNA reagent | pHIS-BamD (Plasmid) | Chen et al., 2021 | For protein expression for NR | |
Recombinant DNA reagent | pET15b-OmpC (Plasmid) | This paper | For analysis of in vitro folding of OmpC | |
Recombinant DNA reagent | pBAD-FLOmpC (Plasmid) | This paper | For analysis of OmpC assembly in vivo | |
Recombinant DNA reagent | pHIS-BamD (X) amber (Plasmid) | This paper | For in vitro photo-cross-linking | |
Recombinant DNA reagent | pHIS-BamD (X) cys (Plasmid) | This paper | For in vitro disulfide cross-linking | |
Recombinant DNA reagent | pAp-BamD (Plasmid) | This paper | For analysis of bamD function | |
Antibody | Anti-DYKDDDDK (Mouse monoclonal) | FUJIFILM-Wako | Cat# 014-22383 | SDS-WB(1:5000) BN-WB(1:5000) |
Antibody | Anti-BamA (Rabbit polyclonal) | Lithgow lab | SDS-WB(1:10,000) BN-WB(1:10,000) | |
Antibody | Anti-BamB (Rabbit polyclonal) | Lithgow lab | SDS-WB(1:10,000) BN-WB(1:5000) | |
Antibody | Anti-BamC (Rabbit polyclonal) | Shiota lab | SDS-WB(1:20,000) Ab-Shift(1:100) | |
Antibody | Anti-BamD (Rabbit polyclonal) | Lithgow lab | SDS-WB(1:10,000) BN-WB(1:5000) | |
Antibody | Anti-BamE (Rabbit polyclonal) | Lithgow lab | SDS-WB(1:10,000) | |
Antibody | Anti-OmpF (Rabbit polyclonal) | Lithgow lab | SDS-WB(1:10,000) BN-WB(1:5000) | |
Antibody | Anti-OmpA (Rabbit polyclonal) | Shiota lab | SDS-WB(1:10,000) | |
Antibody | Anti-OmpC (Rabbit polyclonal) | Lithgow lab | SDS-WB(1:10,000) | |
Antibody | Anti-Mouse IgG-Peroxidase (Produced in goat) | Sigma-Aldrich | Cat# A4416 | 1:20,000 |
Antibody | Anti-Rabbit IgG-Peroxidase (Produced in goat) | Sigma-Aldrich | Cat# A6154 | 1:20,000 |
Peptide, recombinant protein | Peptides containing a partial sequence of OmpC | Mimotopes | Custom peptide ‘Pepset’ | |
Commercial assay or kit | Rabbit Reticulocyte Lysate, Nuclease-Treated | Promega | Cat# L4960 | |
Commercial assay or kit | EXPRESS35S Protein Labeling Mix | Perkin Elmer | Cat# NEG072 | |
Commercial assay or kit | SP6 RNA Polymerase | Clontech | Cat# 2520A | |
Commercial assay or kit | Can Get Signal Immunoreaction Enhancer Solution | TOYOBO | Cat# NKB-101 | |
Commercial assay or kit | Chemi-Lumi One L | Nacalai tesque | Cat# #07880 | 0.03 ml/cm2 |
Chemical compound, drug | n-Dodecyl-β-D-maltoside | Dojindo | Cat# #34106161 | |
Chemical compound, drug | p-Benzoyl-L-phenylalanine | Watanabe Chemical Industries | Cat# #H00146 | |
Other | Gold-coated Si-Wafer | Yamanaka Hutech | Custom ordered | Gold coat was performed by Melbourne Centre for Nanofabrication |
-
E. coli strains and growth conditions.
-
E. coli strains used in this study are listed in Supplementary file 1. All strains were grown in LB (1% tryptone, 1% NaCl, and 0.5% yeast extract). E. coli strains used for the in vivo photo-cross-linking experiments were grown in XB (1% tryptone, 1% NaCl, and 0.1% yeast extract). Unless otherwise specified, ampicillin (amp; 50 mg/ml), chloramphenicol (Cm; 10 mg/ml), or kanamycin (Kan, 30 mg/ml) were added to media for plasmid selection.
Additional files
-
Supplementary file 1
Bacterial strains.
- https://cdn.elifesciences.org/articles/90274/elife-90274-supp1-v1.docx
-
Supplementary file 2
Primers.
- https://cdn.elifesciences.org/articles/90274/elife-90274-supp2-v1.docx
-
Supplementary file 3
Primers for p-benzoyl-L-phenylalanine (BPA).
- https://cdn.elifesciences.org/articles/90274/elife-90274-supp3-v1.docx
-
Supplementary file 4
Plasmids.
- https://cdn.elifesciences.org/articles/90274/elife-90274-supp4-v1.docx
-
Supplementary file 5
Plasmids for recombinants.
- https://cdn.elifesciences.org/articles/90274/elife-90274-supp5-v1.docx
-
Supplementary file 6
Plasmids in vivo.
- https://cdn.elifesciences.org/articles/90274/elife-90274-supp6-v1.docx
-
Supplementary file 7
Structural data.
- https://cdn.elifesciences.org/articles/90274/elife-90274-supp7-v1.docx
-
Supplementary file 8
Characterization of BamA.
- https://cdn.elifesciences.org/articles/90274/elife-90274-supp8-v1.docx
-
Supplementary file 9
Characterization of BamAD.
- https://cdn.elifesciences.org/articles/90274/elife-90274-supp9-v1.docx
-
Supplementary file 10
Characterization of BamAD-OmpC.
- https://cdn.elifesciences.org/articles/90274/elife-90274-supp10-v1.docx
-
MDAR checklist
- https://cdn.elifesciences.org/articles/90274/elife-90274-mdarchecklist1-v1.docx