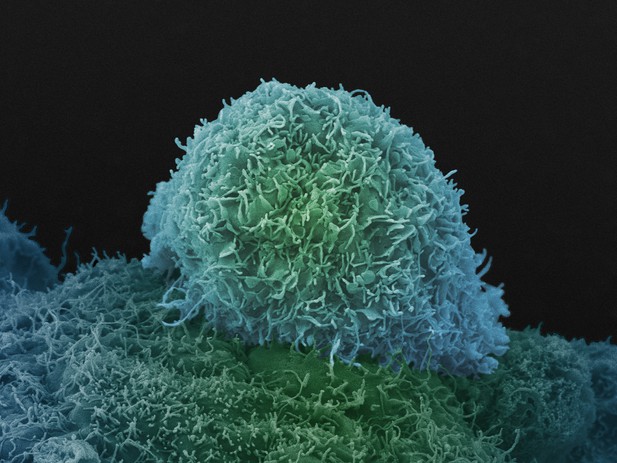
A single prostate cancer cell. Image credit: Anne Weston (CC BY-NC 4.0)
Cancers often begin as cells that grow in connected sheets or clumps known as epithelial cells. To spread, the cancer cells need to change into cells that can break away from the group and move through the tissues. In prostate cancer, this process can happen years after successful treatment, but researchers are not sure why.
Prostate cancer grows in response to testosterone. This hormone circulates around the body, and when it goes into a cell it helps select which genes are switched on or off. Testosterone-blocking drugs can help slow prostate cancer growth by changing this switching on and off of genes. But, over time, some cancers become resistant to the effects of these drugs and start to spread. This may be down to complexities in how testosterone controls gene activity.
To produce a protein, a human cell first makes a copy of the corresponding gene. This copy is then modified, cutting and pasting different parts of the sequence (a process called ‘splicing’) before the protein is produced. The patterns of splicing a cell exhibits depend on splicing regulator proteins.
Testosterone can change splicing patterns in prostate cancer cells, but researchers did not know how. To find out, Munkley et al. examined a set of genes that turn off in response to testosterone-blocking drugs in people with prostate cancer. This revealed that testosterone controls a master splicing regulator called ESRP2, which is normally present in epithelial cells. In prostate cancer cells in mice, extra ESRP2 slowed tumour growth. But, although ESRP2 levels are high in human prostate cancer cells to begin with, they drop in response to testosterone-blocking drugs. In the laboratory grown cells, the result was a switch away from 'epithelial-like' gene splicing patterns. Some of the new splicing patterns correlated with better patient prognosis, but other splicing patterns might help cancer cells to spread around the body.
These results raise the possibility that blocking testosterone may impair prostate cancer growth, but also inadvertently prepare cancer cells to break away from tumours. A more complete understanding of how testosterone controls splicing could help explain why some tumours initially shrink when testosterone is blocked, but then later spread. Identifying the genes controlled by ESRP2 may reveal new drug targets to improve prostate cancer treatment.