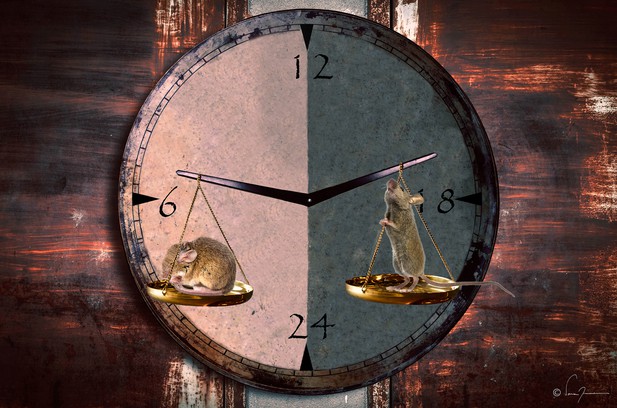
A clock with each half of the face in different colors representing the sleep and wake times, with a sleeping mouse slowing the clock and an awake mouse accelerating the clock. Image credit: Sonia Jimenez (CC BY 4.0)
Circadian rhythms are daily cycles in behavior and physiology which repeat approximately every 24 hours. The master regulator of these rhythms is located in a small part of the brain called the supra-chiasmatic nucleus. This brain structure regulates the timing of sleep and wakefulness and is also thought to control the daily rhythms of cells throughout the body on a molecular level. It does this by synchronizing the activity of a set of genes called clock genes.
Under normal conditions, the levels of proteins coded for by clock genes change throughout the day following a rhythm that matches sleep-wake patterns. However, keeping animals and humans awake at their preferred sleeping times affects the protein levels of clock genes in many tissues of the body. This suggests that, in addition to the supra-chiasmatic nucleus, sleep-wake cycles may also influence clock-gene rhythms throughout the body.
To test this theory, Hoekstra, Jan et al. measured the levels of PERIOD-2, a protein coded for by the clock gene Period-2, while tracking sleep-wake states in mice. They did this by imaging a bioluminescent version of the PERIOD-2 protein in the brain and the kidneys, at the same time as they recorded the brain activity, movement and muscle response of animals. Results showed that PERIOD-2 increased on waking and decreased when mice fell asleep. Additionally, in mice lacking a circadian rhythm in sleep-wake behavior – whose changes in PERIOD-2 levels with respect to time were greatly reduced – imposing a regular sleep-wake cycle restored normal PERIOD-2 rhythmicity.
Next, Hoekstra, Jan et al. developed a mathematical model to understand how sleep-wake cycles together with circadian rhythms affect clock-gene activity in the brain and kidneys. Computer simulations suggested that sleep-wake cycles and circadian factors act as forces of comparable strength driving clock-gene dynamics. Both need to act in concert to keep clock-genes rhythmic. The model also predicted the large and immediate effects of sleep deprivation on PERIOD-2 levels, giving further credence to the idea that waking accelerated clock-gene rhythms while sleeping slowed them down. Modelling also suggested that having regular clock-gene rhythms protects against sleep disturbances.
In summary, this work shows how sleep patterns contribute to the daily rhythms in clock genes in the brain and body. The findings support the idea that well-timed sleep-wake schedules could help people to adjust to new time zones. It might also be useful to inform other strategies to reduce the health impacts of shift work.