The return to water in ancestral Xenopus was accompanied by a novel mechanism for producing and shaping vocal signals
Figures
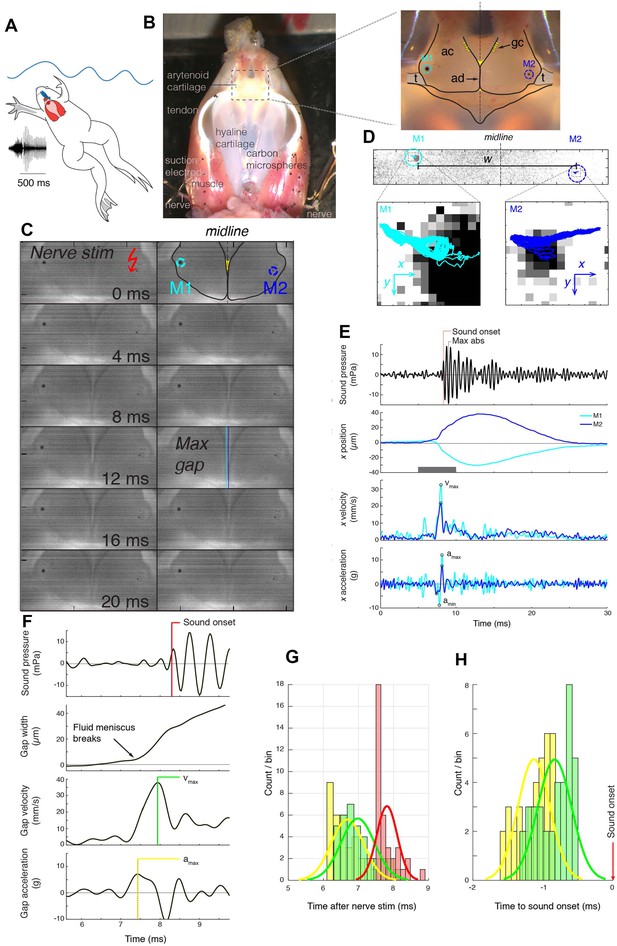
Arytenoid disc kinematics associated with underwater sound production in the ex vivo larynx of Xenopus laevis.
(A) Xenopus call while submerged. A ventral view of a reproductively active, X. laevis male (nuptial pads in grey on the inner surface of the forearms), underwater (blue waves); larynx in red and more dorsal brain in blue. This view of the larynx is schematic (i.e. the dorsal rather than the ventral side is illustrated) in order to correspond to the actual isolated larynx in (B). On the left, an oscillogram (sound intensity vs time) of a single, biphasic call that includes a fast and slow trill. Each vertical line indicates a sound pulse; ~60 pulses/s for fast trill and ~30 pulses/s for slow trill. (B) Dorsal aspect of an isolated X. laevis larynx, a cricoid box of hyaline cartilage flanked by muscles. Each effective contraction/relaxation of these paired laryngeal muscles produces a single sound pulse. In the preparation illustrated, sound pulses are evoked by electrical stimulation of both laryngeal nerves via suction electrodes. Inset: Each muscle contraction produces a transient increase in tension on the arytenoid discs (ad) located within the arytenoid cartilages (ac) via the tendons (t). Globule cells (gc) secrete a mucopolysaccharide onto the medial surfaces of the arytenoid discs (Yager, 1992). Carbon microspheres (e.g. M1 and M2) placed on the surface of the larynx track muscle and cartilage positions. (C) Still photo of arytenoid cartilage motion filmed at 10,000 fps and illustrated at 2 ms intervals. Nerve stimulation occurs in top left image. (D) Upper panel: Higher magnification images of each bead in B) during filming at 44,000 fps. Lower panel: The small motion of each bead (cyan, left; blue, right) during 40 consecutive stimulations at 40 Hz. (E) Sound (top panel, corrected for time of flight) and the position, velocity and acceleration of the two beads (color coded as in D) during a single pulse. Nerve stimulation occurs at t = 0. (F) Kinematic data for gap width (w) in C) in relation to sound onset. While the precise onset (red line) is hard to determine due to acoustic noise, sound production follows peak velocity (green) or acceleration (yellow). (G) The timing of sound onset (red), gap peak acceleration (yellow) and peak velocity (green) during 40 consecutive clicks for one larynx relative to nerve stimulation. (H) Sound onset relative to gap peak acceleration (yellow) and peak velocity (green) during 40 consecutive clicks for the larynx in G).
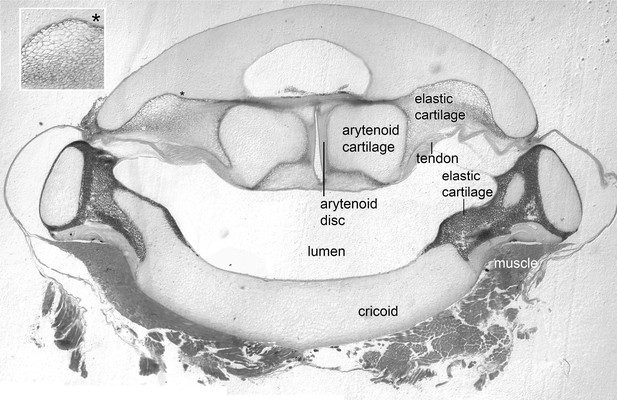
The arytenoid discs are suspended in elastic cartilage.
Transverse section (5 μm) through a decalcified, osmicated, epon-embedded larynx of a male X. laevis at the anterior-posterior level of the arytenoid discs (ad, Figure 1B inset); dorsal is up. As in X. borealis, (Yager, 1992) the anterior arytenoid cartilages and arytenoid discs are suspended in elastic tissue including elastic cartilage identifiable by its characteristic ‘Swiss-cheese’ appearance (inset at left). Seams of elastic cartilage also insert bilaterally onto the cricoid box (cricoid), composed of hyaline cartilage, and form the septa between the lateral and medial chambers more posteriorly (illustrated in Figure 4C). Laryngeal muscle inserts via the tendon onto the arytenoid cartilages just posterior to the level of this section (see Figure 1B and inset).
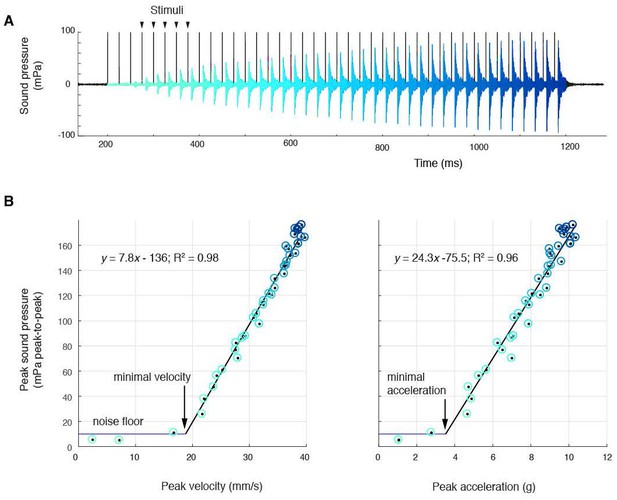
Sound amplitude correlates linearly with disc kinematics, (A) Sound pressure across 40 consecutive stimulations (black vertical lines) at 40 Hz (aqua, first stimulus; blue, last stimulus).
(B) Peak sound pressure per stimulus (color-coded as in A) increases linearly with peak velocity (linear regression for three individuals: y = 5.2x-210.5, R2 = 0.96; y = 1.8x-35.1, R2 = 0.89; y = 7.8–136.3, R2 = 0.98) and peak acceleration (linear regression for three individuals: y = 14.3x-106.9, R2 = 0.91; y = 5.6x-6.1, R2 = 0.89; y = 24.3–75.5, R2 = 0.96). A minimal velocity and acceleration is required before sound is radiated
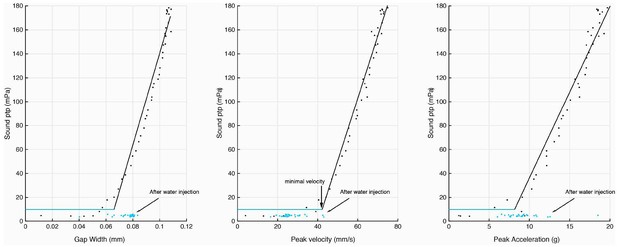
Injection of water into the supra-disc space via the glottis abolishes sound pulse production.
Disc gap width, peak velocity and peak acceleration in relation to sound production before (black) and after (blue) water introduction. Following water injection, only disc velocity did not attain the minimal value associated with detection of sound pulses above the noise floor (as measured before water was injected).
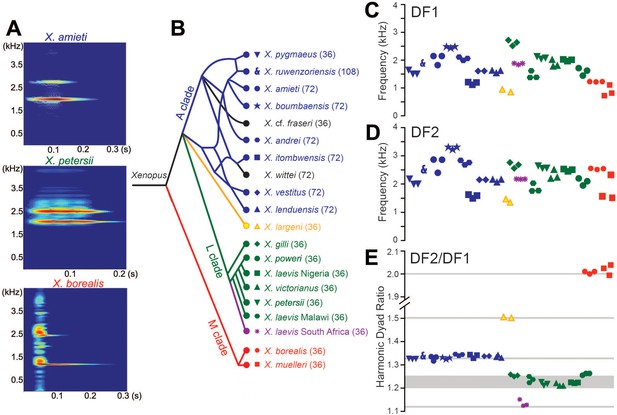
Harmonic dyad ratios are specific to, and highly conserved within, each Xenopus clade (blue: Clade A, green: Clade L; red: Clade M).
(A) Each sound pulse in an advertisement call includes two dominant frequencies (DFs). Top: Spectrogram of multiple sound pulses in a X. amieti (A clade) advertisement call. Middle: multiple sound pulses in a X. petersii (L clade) advertisement call. Bottom: One sound pulse in a X. borealis (M clade) advertisement call. (B) Phylogenetic relationships of Xenopus species in this study. Clades and the DF2/DF1 ratios are: 1.33 (blue, A clade), 1.21–1.26 (green, L clade), and 2.0 (red, M clade). X. allofraseri (black) sound pulses are harmonic stacks. X. laevis South Africa (purple) and X. wittei (black) sound pulses are exceptions to their species group ratios. The ploidy level (number of chromosomes) is in parentheses; the DF2/DF1 (dyad) ratio for individual male calls for each species is indicated by a unique combination of symbol and color (B–E). The value of the lower dominant frequency (DF1; C) and the higher dominant frequency (DF2; D) respectively, in advertisement call sound pulses across Xenopus. (E) Harmonic dyad ratios fall into three major bands, one for each clade.
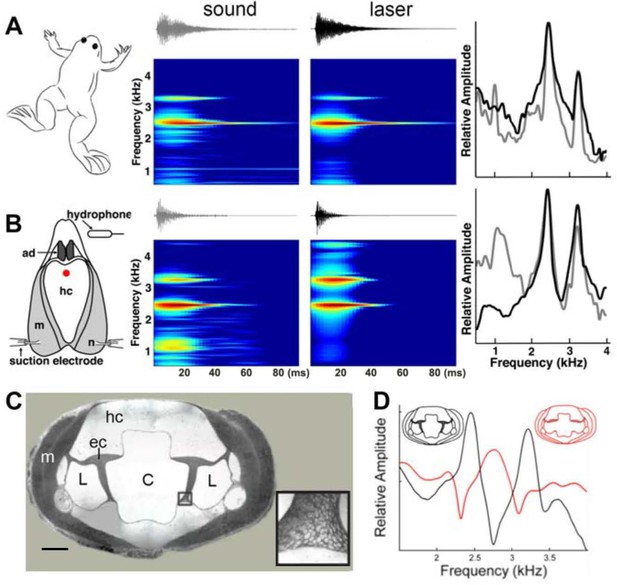
Harmonic dyad production is intrinsic to the isolated larynx and requires intact elastic cartilage septa.
A singing male and an isolated larynx with intact elastic cartilage septa produce the same dyad in sound and laser recordings (A) Recordings from a X. boumbaensis male. Spectrograph (frequency vs time) of one pulse obtained from sound and laser vibrometry recordings. At right: Power spectra from the sound (grey) and laser (black) spectrographs. (B) Recordings from the isolated larynx of a X. boumbaensis male. Spectrograph (frequency vs time) of one pulse obtained from sound and laser vibrometry recordings, At right: Power spectra from the sound (grey) and laser (black) spectrographs. Note that the broad band frequency peak ~1 kHz in the sound recordings (gray) is not present in the laser recordings (black) and is thus an artifact of recording conditions (glass aquaria or Petri dish). Spectrographs are color graded to show increased intensity (blue to red). C - D Intact elastic cartilage is required for the production of frequency dyads. (C) Transverse section of an osmicated, epon-embedded male X. laevis larynx just anterior to the nerve (n) entry point in B; dorsal is up. The hyaline cartilage (hc) frame is flanked laterally by paired, bipennate laryngeal dilator muscles (m). Elastic cartilage sheets divide the lumen of the larynx into a central chamber (C) and two symmetrical lateral chambers (L). Elastic cartilage is recognizable by its unique, lace-like cellular morphology (inset). Scale bar 1 mm. (D) When elastic cartilage of the isolated larynx is disrupted bilaterally (red) via puncture (see Figure 1—figure supplement 1), the two DFs characteristic of the intact larynx (in black) are replaced by a single, intermediate DF in red).
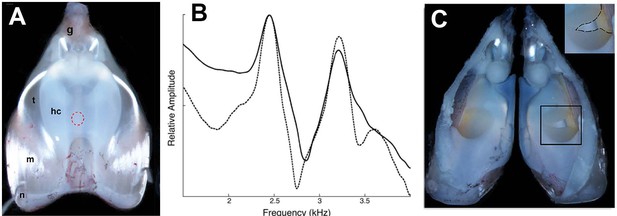
Disruption of the elastic cartilage in an isolated larynx; the hole used to access arytenoid cartilages does not, by itself, disrupt dyads.
(A) Dorsal surface of an isolated larynx from X. laevis. The placement of the hole through which elastic cartilage septa were accessed is indicated by the dashed red circle. hc = hyaline cartilage, m = muscle, n = nerve, t = tendon, g = glottis. (B) Dyad frequencies before (solid line) and after (dashed line) creation of the hole. (C) Interior of a larynx after puncture of the elastic cartilage (right side; see inset). The elastic cartilage presents a yellowish appearance.
Videos
Motion of arytenoid discs filmed at 10,000 fps during 40 Hz nerve stimulation.
Video playback is slowed down 40x and resampled. The sound pressure waveform is displayed below the image and the sound associated with each frame is in the red area. Two carbon spheres can be seen on each arytenoid (large sphere about 80 µm diameter).
Tables
Spectral features of DF1 and DF2 across Xenopus species.
Average values (Standard Error). DF1: lower dominant frequency, DF2: higher dominant frequency. Additional broadband frequencies present at the attack of each sound pulse are not summarized here because they are not sustained. For both DF1 and DF2, the Q-value is the bandwidth at −6 dB divided by the peak frequency. Musical interval descriptors were assigned if the value of DF2/DF1 fell within 0.02 of a small integer ratio (e.g., 2:1 = Octave, 3:2 = Fifth, 4:3 = Perfect 4th, 5:4 = Major Third, 6:5 = Minor Third).
Species | N | DF1 hz (SE) | DF1 Q-Value (SE) | DF2 hz (SE) | DF2 Q-Value (SE) | DF2/DF1 Ratio (SE) | Musical interval |
---|---|---|---|---|---|---|---|
X. pygmaeus | 3 | 1591 (40) | 16.6 (0.8) | 2124 (54) | 18.1 (2.6) | 1.34 (0.009) | Perfect 4th |
X. ruwenzoriensis | 1 | 1937 | 21.6 | 2575 | 27.8 | 1.33 | Perfect 4th |
X. amieti | 3 | 2043 (107) | 22.4 (1.3) | 2715 (152) | 27.2 (2.4) | 1.33 (0.005) | Perfect 4th |
X. boumbaensis | 3 | 2475 (16) | 27.6 (0.2) | 3291 (29) | 33. 9 (0.4) | 1.33 (0.004) | Perfect 4th |
X. allofraseri | 1 | N/A | N/A | N/A | N/A | N/A | Harmonic stack |
X. andrei | 3 | 2062 (30) | 20.8 (1.5) | 2759 (51) | 26.7 (2.7) | 1.34 (0.006) | Perfect 4th |
X. itombwensis | 3 | 1179 (32) | 12.4 (0.6) | 1573 (38) | 15.3 (2.1) | 1.33 (0.006) | Perfect 4th |
X. wittei | 3 | 1319 (35) | 13.9 (0.7) | 1545 (38) | 15.0 (1.1) | 1.17 (0.003) | Non-consonant |
X. vestitus | 2 | 1609 (2) | 17.7 (0.1) | 2150 (2) | 23.3 (0.3) | 1.34 (0.003) | Perfect 4th |
X. lenduensis | 3 | 1595 (28) | 17.4 (0.2) | 2131 (33) | 22.8 (0.2) | 1.34 (0.004) | Perfect 4th |
X. largeni | 2 | 929 (45) | 10.0 (0.2) | 1387 (59) | 14.7 (0.4) | 1.49 (0.010) | Perfect 5th |
X. gilli | 3 | 2114 (45) | 22.7 (1.3) | 2641 (62) | 28.7 (1.3) | 1.25 (0.003) | Major Third |
X. poweri | 3 | 1714 (28) | 19.1 (0.4) | 2159 (38) | 22.7 (0.1) | 1.26 (0.004) | Major Third |
X. laevis Nigeria | 3 | 2029 (17) | 22.5 (0.2) | 2481 (22) | 26.6 (0.2) | 1.22 (0.004) | Minor Third |
X. victorianus | 3 | 1891 (78) | 21.3 (0.9) | 2295 (102) | 22.4 (1.5) | 1.21 (0.005) | Minor Third |
X. petersii | 3 | 2124 (24) | 23.6 (0.3) | 2576 (22) | 27.7 (0.3) | 1.21 (0.004) | Minor third |
X. laevis Malawi | 3 | 1482 (80) | 15.8 (0.3) | 1831 (75) | 17.3 (0.7) | 1.24 (0.016) | Major Third |
X. laevis South Africa | 3 | 1879 (21) | 16.0 (0.9) | 2154 (9) | 18.9 (2.2) | 1.15 (0.008) | Non-consonant |
X. borealis | 3 | 1253 (5) | 13.9 (0.1) | 2504 (11) | 27.8 (0.4) | 2.00 (0.000) | Octave |
X. muelleri | 2 | 1107 (29) | 12.2 (0.5) | 2262 (23) | 16.0 (6.1) | 2.04 (0.032) | Octave |
Additional files
-
Transparent reporting form
- https://doi.org/10.7554/eLife.39946.012