Protein phase separation and its role in tumorigenesis
Figures
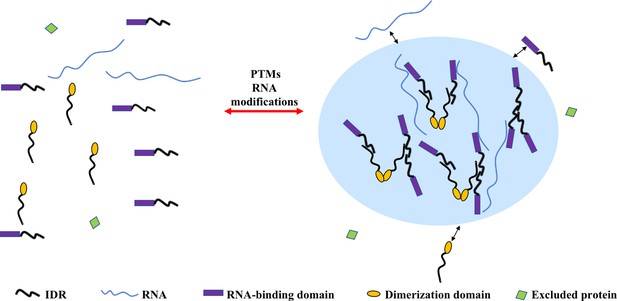
Multivalent interactions drive the assembly of condensates.
The diagram depicts different multivalent interactions among proteins or proteins and RNA, which drive the formation of liquid droplets by phase separation. Post-translational modifications (PTMs) (e.g. phosphorylation, acetylation, arginine methylation, and SUMOylation) or RNA modifications (such as m6A modification) can increase or decrease the driving forces for phase separation and thus regulate the assembly or disassembly of liquid droplet by phase separation, respectively. Components inside the droplet can exchange dynamically with the dilute phase (indicated by the two-sided black arrows). Some proteins are excluded from the condensate because they do not interact with the molecules in the dense phase (molecule with green domain).
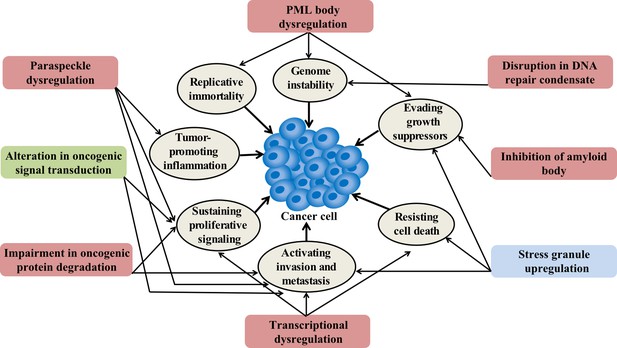
Condensation-related cellular process involved in cancer.
Cancer-related proteins may regulate condensate assembly and cancer-associated mutations may alter condensate assembly, thus driving aberrant cellular processes that promote tumorigenesis and cancer progression. Additionally, condensate dysfunction could also play important roles in the development of cancer. Potential links between aberrant condensates and hallmarks of cancer are indicated by arrows. The color of the box indicates the localization of condensates. Red means localize in the nuclear, blue means localize in the cytoplasm, and green means localize in both locations.
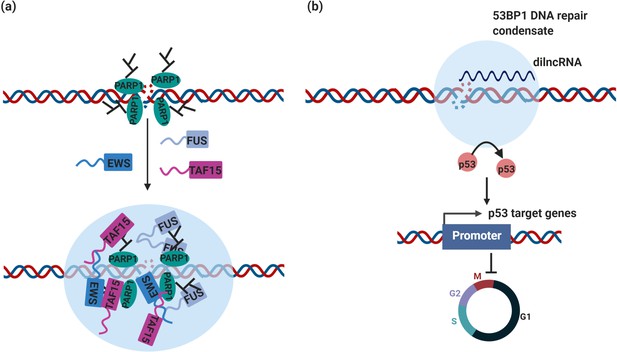
DNA repair condensates in the maintenance of genomic stability.
(a) DNA damage induces PARylation by PARP around the DSB area, which creates a platform for protein assembly. The recruitment of RBPs containing prion-like domain, such as FUS, EWS, TAF15, drives the assembly of DNA damage condensates at DNA double strand break sites. (b) Synthesis of dilnc RNA promotes the assembly of 53BP1 repair condensates at DNA double strand breaks. These condensates recruit p53, which stabilizes p53 and promotes activation of p53 target genes and cell cycle arrest. It is possible and has not been tested yet that these condensates are different stages of DNA damage condensates, condensates in (a) are the early stage of DNA damage condensates, condensates in (b) are the late stage of DNA damage condensates.
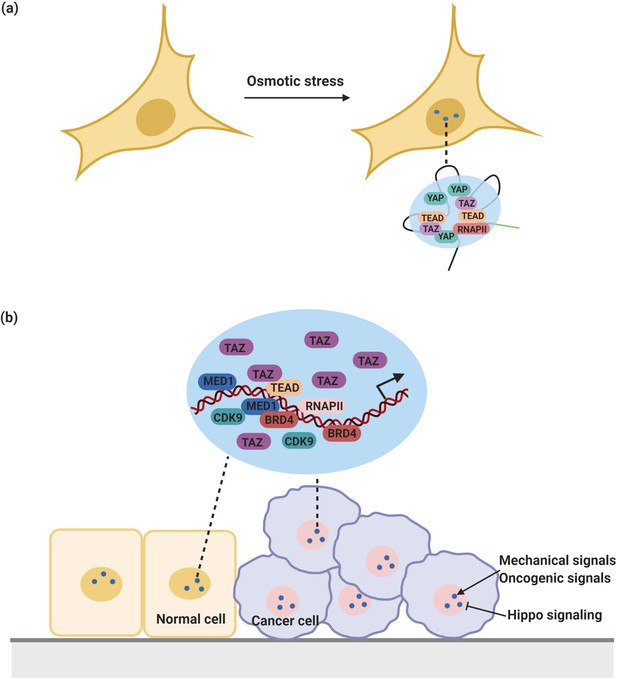
YAP/TAZ condensates in the regulation of transcription.
(a) YAP forms nuclear condensates during osmotic stress. These YAP condensates co-localize with TAZ and TEAD and recruit RNA Pol II to trigger transcription of YAP target gene. (b) TAZ also forms nuclear condensates which compartmentalize TEAD4, BRD4 and MED1, RNAPII, and the transcription elongation factor CDK9 for transcription. These condensates are negatively regulated by Hippo signaling and sensitive to mechanical signals and oncogenic signals from around the environment.
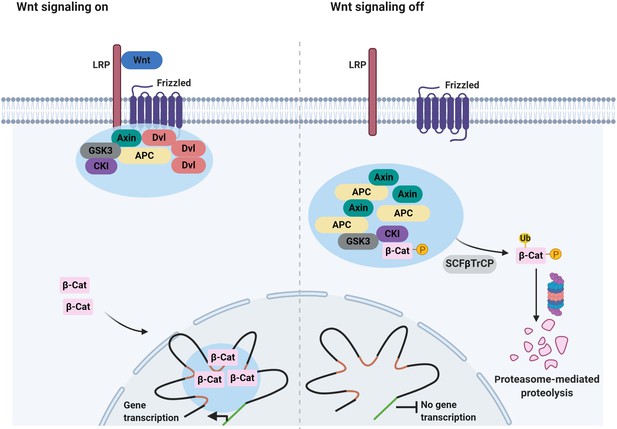
Signaling-associated condensates that may form in the Wnt/β-catenin signaling pathway.
(Left) Wnt signaling triggers the assembly of 2D membrane clusters containing the receptors Frizzled and LRP as well as Dvl, Axin, and other components of destruction complex, thus disrupting destruction complex regulating degradation of β-catenin. Consequently, β-catenin accumulates and enters the nucleus, where it may localize to condensates at super-enhancers to elicit the transcription of target genes. (Right) In the absence of a Wnt ligand, Axin, and APC assemble into a destruction complex condensate that recruits kinases such as GSK3 and casein kinase I (CKI). This in turn promotes phosphorylation of β-catenin and subsequent ubiquitin-mediated degradation of β-catenin by the proteasome. Ubiquitination of phosphorylated β-catenin is mediated by the ubiquitin ligase SCFβTrCP.
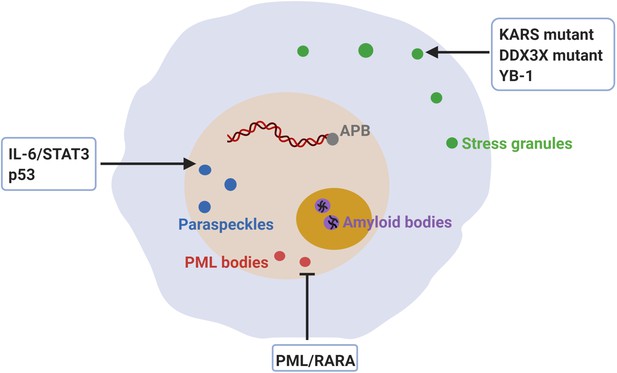
Dysregulation of membraneless compartments in cancer.
Aberrant assembly of SGs, PML bodies or paraspeckles may arise from dysregulation of or mutations in cancer-related proteins, thus promoting tumorigenesis. For instance, KRAS mutations promote SG hyper-assembly and this has been shown to confer a fitness advantage to cancer cells (Grabocka and Bar-Sagi, 2016). Likewise, mutations in DDX3X cause SG hyper-assembly and this has been shown to impair protein synthesis in medulloblastomas (Valentin-Vega et al., 2016). Moreover, YB-1 promotes SG assembly, which has been linked to increased invasion and metastasis (Somasekharan et al., 2015). The expression of PML/RARA leads to disruption of PML body assembly and deregulated transcriptional control of senescence and differentiation in acute promyelocytic leukemia (APL) (de Thé and Chen, 2010; Dos Santos et al., 2013). Furthermore, disruption of PML bodies contributes to APL pathogenesis by increasing genome instability (Voisset et al., 2018). Recombination-based alternative lengthening of telomeres (ALT) is a key mechanism for telomerase-negative cancer cells to maintain the telomere stability and the capability for unlimited proliferation (Bryan et al., 1997). ALT-associated PML bodies (APB) facilitate telomere maintenance and thus promote cancer cell immortality (Zhang et al., 2020). IL-6/STAT3 signaling promotes paraspeckles formation, which favors overactivation of STAT3 in human hepatocellular carcinoma (HCC) (Wang et al., 2018c). Paraspeckle assembly induced by p53 has been shown to inhibit cancer initiation in pancreatic cancer models (Mello et al., 2017). Finally, inhibition of amyloid body assembly has been shown to promote tumor tissue growth (Audas et al., 2016).
Tables
Cancer-related proteins involved in formation and regulation of condensates.
Biomolecular condensates | Cancer-related protein or RNA molecular | Verification of phase behavior | References |
---|---|---|---|
SPOP/DAXX bodies | SPOP | Yes | Bouchard et al., 2018 |
DNA repair condensates | 53BP1 | Yes | Kilic et al., 2019 |
DNA damage condensates | PARP-1 | Yes | Altmeyer et al., 2015; Patel et al., 2015 |
DNA damage condensates | FUS | Yes | Altmeyer et al., 2015; Patel et al., 2015 |
DNA damage condensates | EWS | Yes | Altmeyer et al., 2015; Patel et al., 2015 |
DNA damage condensates | TAF15 | Yes | Altmeyer et al., 2015; Patel et al., 2015 |
Transcriptional condensates | EWS-FLI1 | Predicted | Boulay et al., 2017 |
Transcriptional condensates | YAP | Yes | Cai et al., 2019 |
Transcriptional condensates | TAZ | Yes | Lu et al., 2020 |
PRC1 condensates | CBX2 | Yes | Plys et al., 2019; Tatavosian et al., 2019 |
Transcriptional condensates | β-catenin | Yes | Zamudio et al., 2019 |
p62 bodies | p62 | Yes | Cloer et al., 2018; Sun et al., 2018; Zaffagnini et al., 2018 |
Stress granules | KRAS | Unconfirmed | Grabocka and Bar-Sagi, 2016 |
Stress granules | DDX3X | Yes (only in vitro) | Hondele et al., 2019; Valentin-Vega et al., 2016 |
Stress granules | YB-1 | Unconfirmed | Somasekharan et al., 2015 |
PML NBs | PML/RARA | Unconfirmed | de Thé et al., 2017; Dos Santos et al., 2013 |
Paraspeckles | NEAT1_2 | Yes | Yamazaki et al., 2018 |