The push–pull intercrop Desmodium does not repel, but intercepts and kills pests
Figures
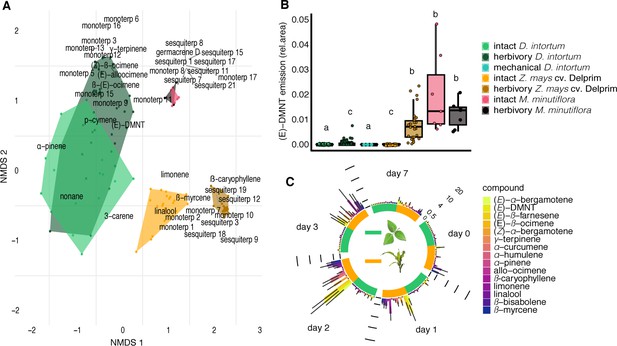
Desmodium intortum does not constitutively release terpene volatiles, and hardly following larval feeding.
(A) Non-metric multidimensional scaling (NMDS) analysis of volatiles emitted by D. intortum, Z. mays cv. Delprim, and M. minutiflora plants, intact and 48 hr following S. frugiperda feeding (stress value = 0.138). (E)-4,8-Dimethyl-1,3,7-nonatriene ((E)-DMNT), (Z)-β-ocimene, (E)-β-ocimene, and (E)-alloocimene were not constitutively released, and only in low quantities in response to herbivory. Volatiles emitted by intact and herbivore-induced D. intortum (n=7 each, Fmodel = 15.597, R2 = 0.132, padj = 0.021) and Z. mays plants (n=7 each, Fmodel = 50.521, R2 = 0.512, padj = 0.021) were significantly different in PERMANOVA and pairwise comparison, but emissions from intact and herbivore-induced M. minutiflora plants (n=7, Fmodel = 1.469, R2 = 0.109, padj = 1) were not. (B) (E)-DMNT emission before and 48 hr following herbivory (n = 8, errir bar represents ± standard error [SE]). The absolute peak areas were divided by the peak area of the internal standard and divided by the sum of monoterpenoids across all laboratory volatile collections for normalization. Treatments with different letters are statistically different (Kruskal–Wallis with Benjamini and Hochberg p value correction, χ2 = 57.315, p = 1.578 × 10−10). (C) Emission of volatile monoterpenoids and sesquiterpenoids from D. intortum and Z. mays before, during, and after S. frugiperda larval feeding (n = 5, error bars represent ± standard error [SE]). Peak areas of each terpenoid were divided by the area of the internal standard and divided by the sum of monoterpenoids or sesquiterpenoids across all laboratory volatile collections. Error bars show the standard error for relative volatile emission of each group. Day 0 – volatile emission before herbivory, Day 1 – 24 hr after herbivory, Day 2 – after 48 hr, and so on. Larvae were removed after 48 hr. Figure 1—figure supplement 1. Heatmap showing relative amounts of headspace volatile compounds emitted from intact, herbivore induced and mechanically damaged Desmodium intortum, Zea mays cv. Delprim, and Melinis minutiflora plants grown in a greenhouse. Figure 1—figure supplement 2. Volatile emission of Desmodium uncinatum and Desmodium intortum compared to Melinis minutiflora and Zea mays cv. Delprim. Figure 1—figure supplement 3. Ordination of volatile samples from intact, herbivore damaged, and mechanically damaged Desmodium intortum, Zea mays cv. Delprim, and Melinis minutiflora plants based on non-metric multidimensional scaling (NMDS).
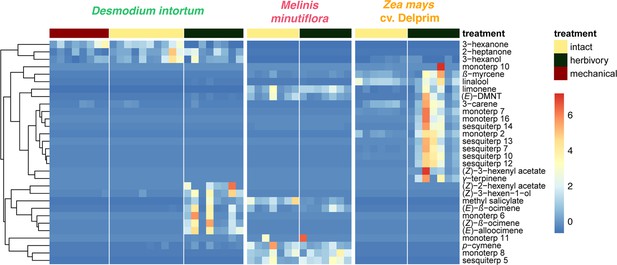
Heatmap showing relative amounts of headspace volatile compounds emitted from intact, herbivore induced and mechanically damaged Desmodium intortum, Zea mays cv. Delprim and Melinis minutiflora plants grown in a greenhouse.
The absolute peak areas were divided by the area of the internal standard peak and z-score was calculated (peak area − mean peak area/standard deviation of peak). The dendrogram of compounds was constructed via hierarchical clustering based on Euclidean distances. The major volatile constituents of intact D. intortum headspace were 2-heptanone and 3-heptanone. Monoterpenoids were only detectable after 48 hr of S. frugiperda feeding, when (E)-4,8-dimethyl-nona-1,3,7-triene ((E)-DMNT), (Z)-β-ocimene, (E)-β-ocimene, and (E)-alloocimene were emitted. The relative (E)-DMNT emission, (E)-β-ocimene emission, and total monoterpenoid emission of intact and herbivore-induced D. intortum were significantly different in pairwise comparisons with Kruskal–Wallis tests and pairwise comparisons with Wilcoxon rank sum test with Benjamini and Hochberg p-correction (n = 7, χ2 = 57.315, p = 0.00012, χ2 = 52.321, p = 8.5 e−05, and χ2 = 52.904, p = 0.00079). Linalool, β-myrcene were present in the headspace of intact maize. In response to 48 hr of larval feeding (E)-DMNT, (Z)-α-bergamotene, β-caryophyllene, (Z)-β-farnesene, humulene, and β-bisabolene were emitted.The relative (E)-DMNT emission and total sesquiterpenoid emission of intact and herbivore-induced Z. mays cv. Delprim was significantly different using the same statistical tests (n = 7, χ2 = 57.315, p = 3.1 e−3 and χ2 = 59.163, p = 8.2 e−4). The volatile headspace of the both intact and herbivore-induced M. minutiflora is composed of a variety of monoterpenoid and sesquiterpenoid compounds, such as (E)-DMNT, limonene, and germacrene D. Neither the relative (E)-DMNT emission nor the total monoterpenoid emission nor the total sesquiterpenoid emission of intact and herbivore-induced M. minutiflora were significantly different in the same statistical tests (n = 7, χ2 = 57.315, p = 0.62, χ2 = 52.904, p = 0.63, and χ2 = 59.163, p = 0.12).
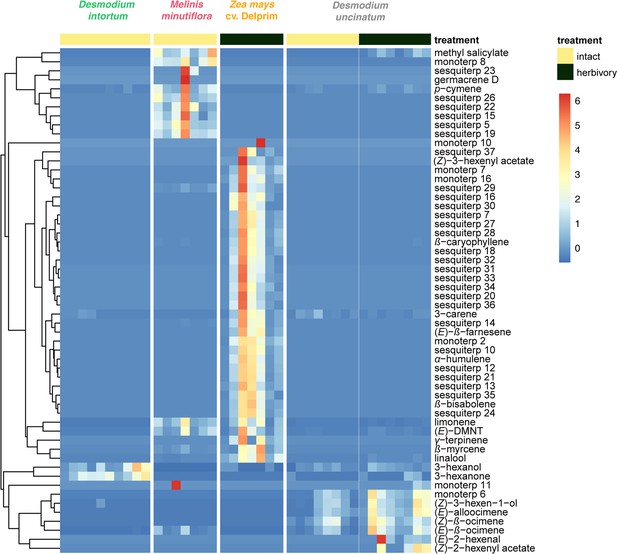
Volatile emission of Desmodium uncinatum and Desmodium intortum compared to Melinis minutiflora and Zea mays cv. Delprim.
The heatmap shows the relative amounts of volatile compounds emitted from intact D. intortum (greenleaf Desmodium), M. minutiflora, and D. uncinatum (silverleaf Desmodium) as well as herbivore-damaged Z. mays (maize) and D. uncinatum plants. The absolute peak areas were divided by the area of the internal standard peak and z-score was calculated (peak area − mean peak area/standard deviation of peak). The dendrogram of compounds was constructed via hierarchical clustering based on Euclidean distances, (n = 7).
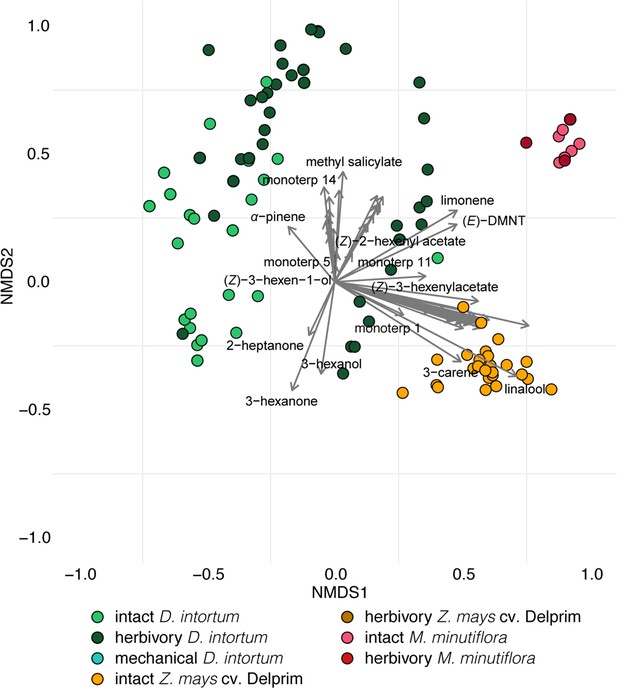
Ordination of volatile samples from intact, herbivore damaged, and mechanically damaged Desmodium intortum, Zea mays cv. Delprim and Melinis minutiflora plants based on non-metric multidimensional scaling (NMDS).
The NMDS plots were based on presence–absence values and calculation of Jaccard dissimilarity indices. The stress value of the plot is 0.138. Vectors represent correlations of volatile features with distribution of plant samples along the NMDS1 and NMDS2 axes.
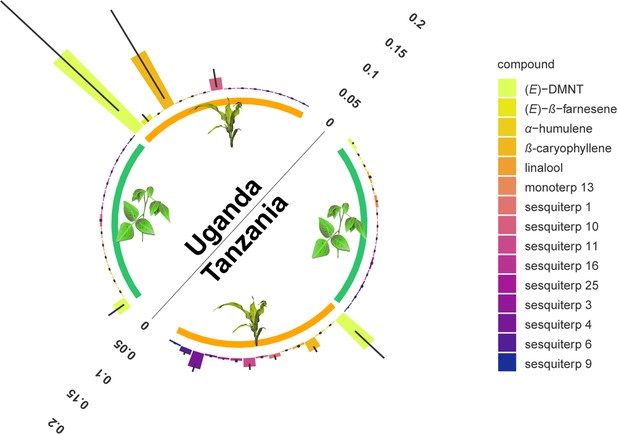
Monoterpenoid and sesquiterpenoid emission by D. intortum and Zea mays plants under field conditions at several locations in Tanzania and Uganda.
The absolute peak area of each peak was divided by the sum of the area of monoterpenoid or sesquiterpenoid emission across all samples from the same location. Error bars represent ± standard error (SE) on the scale of the relative volatile emission (Desmodium: n = 17 and 20, maize = 5 and 15, for locations in Uganda and Tanzania, respectively). Minor terpenoid compounds were not identified to species level as this was not the focus of the study, and was further hindered by the vast diversity of compounds and the lack of synthetic standards. The volatile terpenoids were infrequently observed in the headspace of intact D. intortum plants regardless of soil microbial inoculation. (Figure 2—figure supplement 1) Volatile emission of field grown Desmodium intortum and Zea mays plants from two locations. (Figure 2—figure supplement 2) Volatile emission profile of intact and herbivore-damaged Desmodium intortum and Zea mays grown in soils with different microbial composition. (Figure 2—figure supplement 3) The infrequent observation of volatile terpenoids in the intact Desmodium intortum does not result from poor soil microbiota and insufficient nodulation. (Figure 2—figure supplement 4) The emission profile of Desmodium intortum and Zea mays cv. Delprim was not significantly altered by soil microbial treatments.
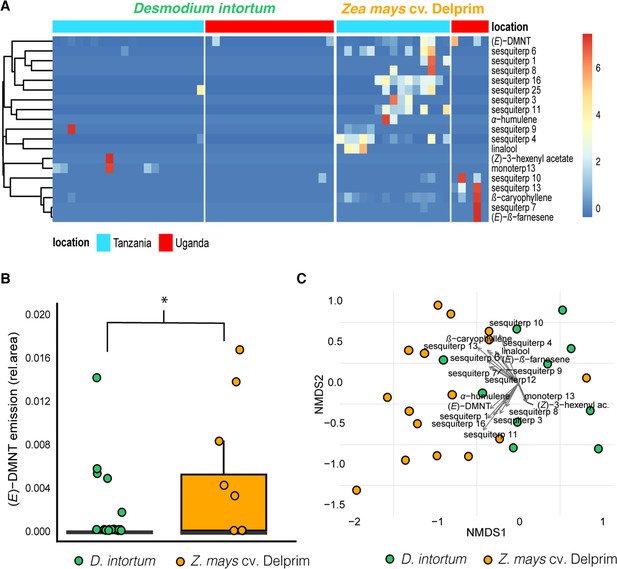
Volatile emission of field grown Desmodium intortum and Zea mays plants from two locations.
(A) Heatmap volatile emissions of D. intortum (greenleaf Desmodium) and Z. mays plants at locations in Tanzania and Uganda. The absolute peak areas were divided by the total area of compounds belonging to monoterpenoids, sesquiterpenoids, or green leaf volatiles per location and z-score was calculated (peak area − mean peak area/standard deviation of peak). The dendrogram of compounds was constructed via hierarchical clustering based on Euclidean distances. (B) Similar to greenhouse experiment, the constitutive emission of monoterpenoids, such as (E)-4,8-dimethyl-nona-1,3,7-triene ((E)-DMNT) and (E)-β-ocimene were not detectable in case of D. intortum plants, due to possible underlying biotic and abiotic stressors emission of (E)-DMNT was visible in a small fraction of D. intortum samples. Based on Kruskal–Wallis tests and Wilcoxon rank sum test with Benjamini and Hochberg p-correction the relative (E)-DMNT abundance of Z. mays (n = 20) volatile samples was significantly higher than that of D. intortum (n = 17) volatile samples (χ2 = 15.310, p = 0.002, * symbol shows that the corrected p-value was less than 0.05). (C) Non-metric multidimensional scaling (NMDS) of the volatile profile of Desmodium intortum and Zea mays plants from field locations. The vectors represent the correlation of volatile features with the distribution of plant samples along the NMDS1 and NMDS2 axes. The stress value of the NMDS plot is 0.116. Based on permutational multivariate analysis of variance (PERMANOVA) and pairwise comparison the volatile profile of D. intortum and Z. mays were significantly different (Fmodel = 8.816, R2 = 0.149, padj = 0.001).
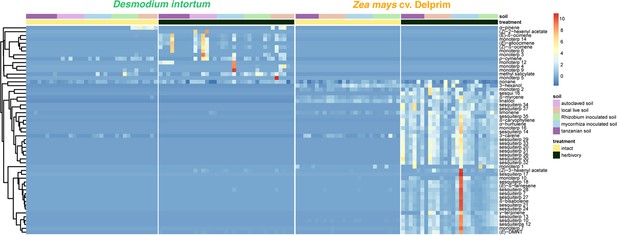
Volatile emission profile of intact and herbivore-damaged Desmodium intortum and Zea mays grown in soils with different microbial composition ( n = 7).
The absolute peak areas were divided by the area of the internal standard peak and z-score was calculated (peak area − mean peak area/standard deviation of peak). The dendrogram of compounds was constructed via hierarchical clustering based on Euclidean distances.
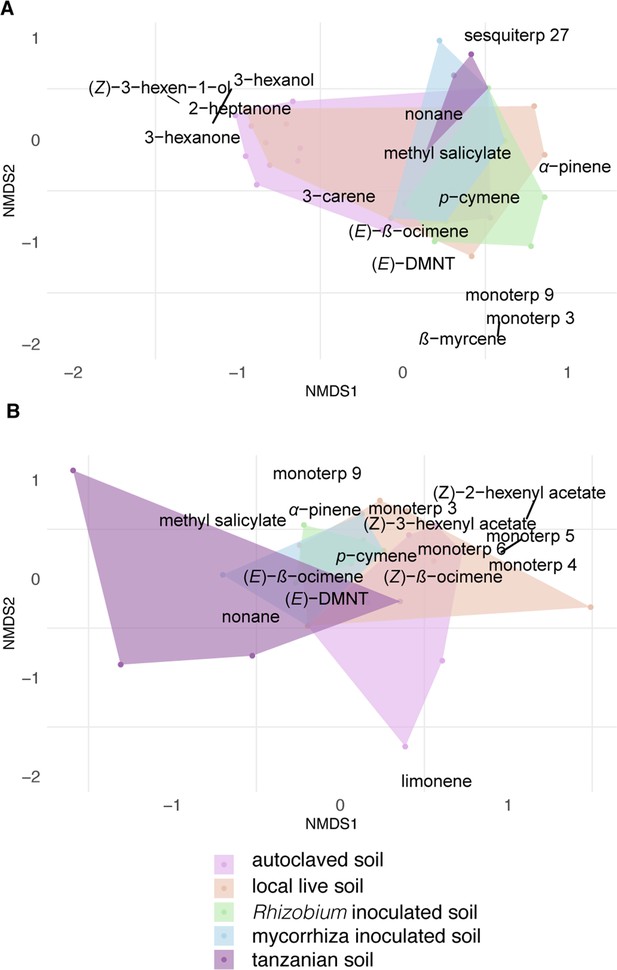
Terpenoids were rarely detected in the volatile samples of intact Desmodium plants and the volatile profile of plants grown in soil inoculated did not differ significantly.
(A) Non-metric multidimensional scaling (NMDS) ordination of volatile profiles from headspace of intact plants, (n = 7). (B) NMDS ordination of herbivore-damaged D. intortum plants grown in different soils in a greenhouse. The stress values of NMDS ordination were 0.146 for intact and 0.120 for herbivore-induced plants. The volatile profile of intact D. intortum on different soil treatments largely overlap while upon herbivory, some differentiation is observed. Scaling is based on Jaccard-distance matrix calculated from centered area values for each compound (n = 7). The stress values are 0.146 and 0.120 for NMDS ordination of intact and herbivore-induced samples. Based on permutational multivariate analysis of variance (PERMANOVA) and pairwise comparison of plants grown in different soil treatments the volatile profile of intact (Fmodel = 3.260, R2 = 0.189, padj = 0.615) and herbivore-induced D. intortum (Fmodel = 7.268, R2 = 0.326, padj = 0.090) did not cluster separately.
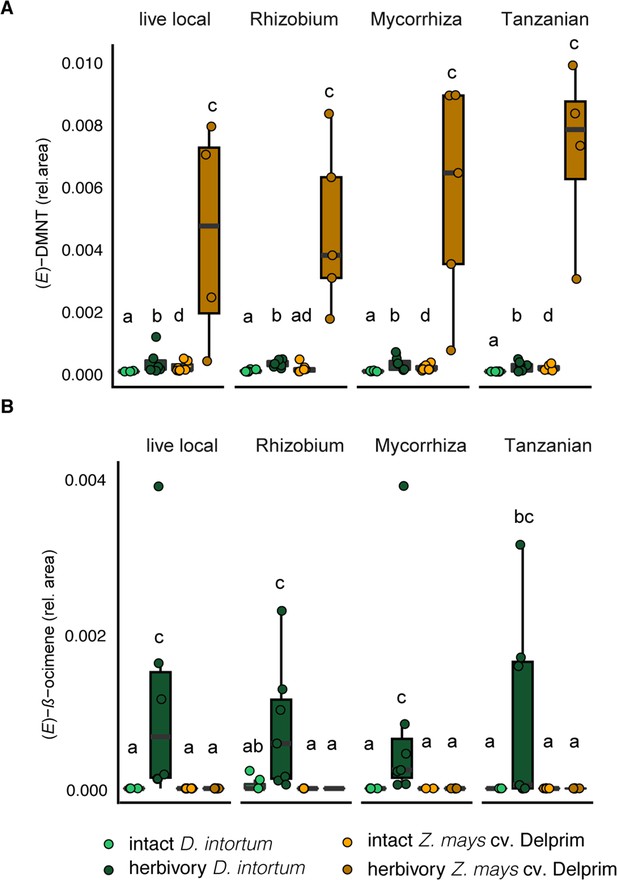
The emission profile of Desmodium intortum and Zea mays cv. Delprim was not significantly altered by soil microbial treatments.
(A) The relative (E)-4,8-dimethyl-nona-1,3,7-triene ((E)-DMNT) emission and (E)-β-ocimene emission of D. intortum and Z. mays cv. Delprim plants grown in soils containing Rhizobium spp., mixture of mycorrhizal fungi and soil of push–pull fields. The absolute peak areas were divided by the area of the internal standard peak to calculate relative values. The error bars show the standard error in relative emission units. Inoculation did not alter significantly the relative (E)-DMNT (n = 7 for each, χ2 = 80.156, p = 0.303). (B) Neither did inoculation affect the (E)-β-ocimene (n = 7 for each, χ2 = 7.688, p = 0.103) emissions of intact D. intortum plants based on pairwise comparisons with Kruskal–Wallis test with Wilcoxon rank sum test with Benjamini and Hochberg p-correction. Herbivore-induced D. intortum plants grown in different soils were also not significantly different from each other in the relative (E)-DMNT (n = 7 for each, χ2 = 5.153, p = 0.272) and (E)-β-ocimene (n = 7 for each, χ2 = 80.395, p = 0.268) emissions. On both plots, bars labelled with the different letters are statistically different.
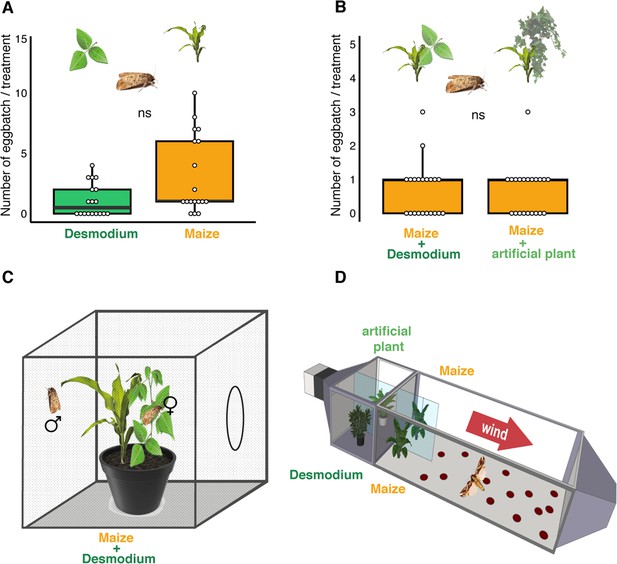
D. intortum does not repel ovipositing S. frugiperda.
(A) The number of egg batches laid on D. intortum or Z. mays plants (n = 25, Wilcoxon signed rank exact test, p = 0.075, ratio of egg batches on other surfaces = 23%) in cage oviposition experiments (setup depicted in C). (B) Number of egg batches on Z. mays plants in a background of either D. intortum plant or a plastic plant mimic did not differ in wind tunnel oviposition assays (n = 21, Wilcoxon signed rank exact test, p = 0.825, ratio of egg batches on walls = 27%). (C) Oviposition experiments were conducted in netted cages and (D) and a modified wind tunnel setup (Figure 3—figure supplement 1). Wind tunnel setup to study the oviposition repellency of Desmodium intortum volatiles (Figure 3—figure supplement 2). The number of eggs laid on D. intortum or Z. mays plants (n = 25, Wilcoxon signed rank exact test, p = 0.105) in cage oviposition experiments (setup depicted in C).
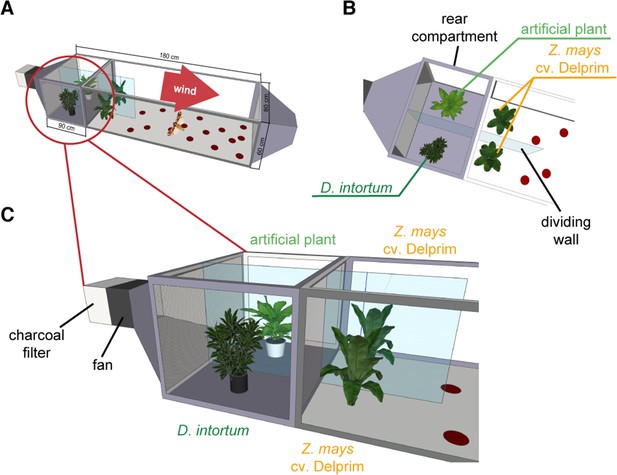
Wind tunnel setup to study the oviposition repellency of Desmodium intortum volatiles.
Two Zea mays cv. Delprim plants were placed in laminar filtered air flow with D. intortum (greenleaf Desmodium) or a plastic mimic plant directly upwind from the flight chamber containing two maize plants. Part (A) shows overview of wind tunnel setup, (B) shows arrangement of plants from above and (C) from the side. A gravid Spodoptera frugiperda female was released in the wind tunnel. The number of egg batches laid on both maize plants was counted and the position of mimic plants and D. intortum plants was randomized.
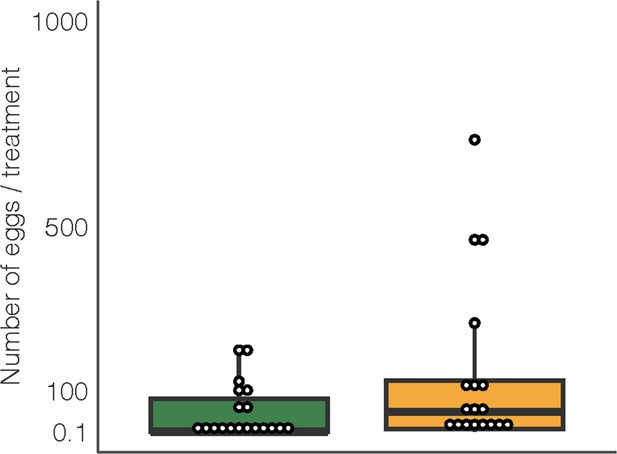
The number of eggs laid on D. intortum or Z. mays plants (n = 25, Wilcoxon signed rank exact test, p = 0.105) in cage oviposition experiments (setup depicted in Figure 2C).
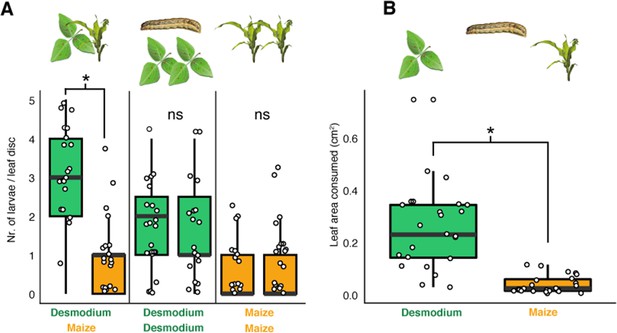
D. intortum is preferred by neonate S. frugiperda larvae.
(A) First instar S. frugiperda larvae preferred D. intortum against Z. mays in two-choice leaf disc bioassays (n = 25, Wilcoxon signed rank exact test, p = 2.73 × 10−3). (B) First instar S. frugiperda larvae consumed more D. intortum than Z. mays (20 hr, two-choice leaf disc bioassays, n = 25, Wilcoxon signed rank exact test, p = 3.338 × 10−6, the ratio of non-settled larvae = 74.2%). The symbol * shows comparisions where p-values were lower than 0.05.
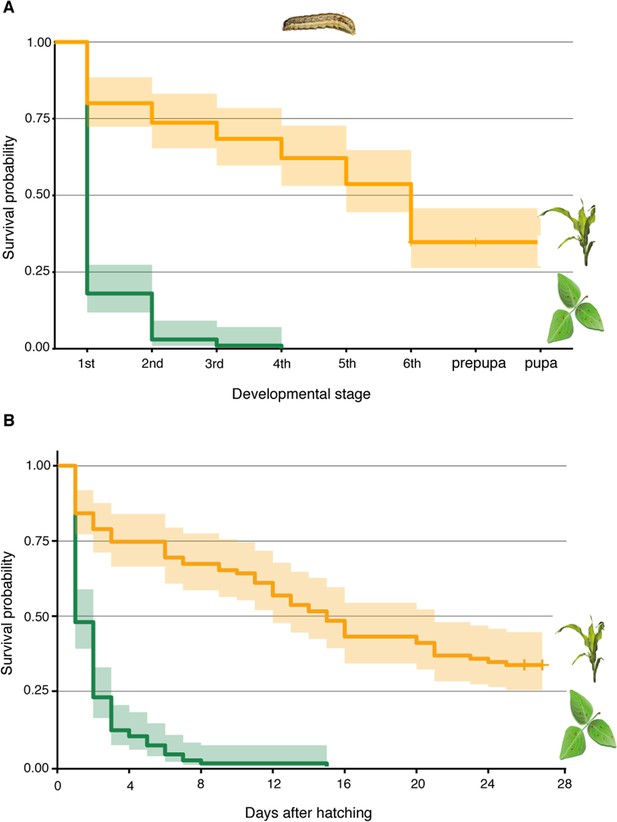
D. intortum is not a suitable host plant for S. frugiperda.
(A) Survival probability of S. frugiperda on diets consisting of D. intortum (greenleaf Desmodium) was lower than on Z. mays in every developmental stage (n = 100, Kaplan–Meier survival analysis, p = 2.000 × 10−16). (B) Larvae on D. intortum diet had significantly higher mortality throughout the experiment than larvae on Z. mays diet (n = 100, Kaplan–Meier survival analysis, p = 2.000 × 10−16). The D. intortum diet resulted in a total mortality by the fourth instar larval stage. Envelope indicates the standard error.
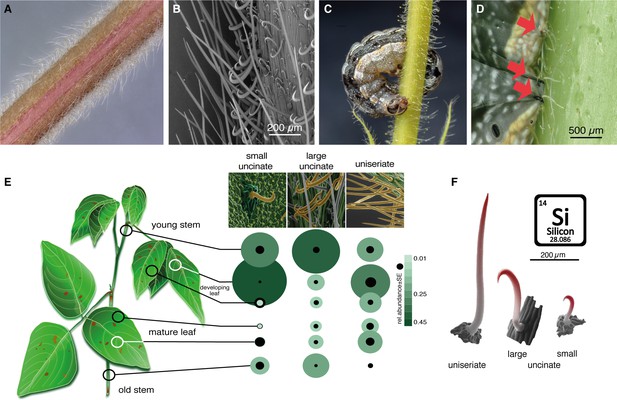
Non-glandular trichomes on Desmodium intortum act as a physical barrier for Spodoptera larvae.
(A) Light microscopy image of a section of a young D. intortum stem densely covered with trichomes. (B) Scanning electron microscopy (SEM) image of a young D. intortum stem. Straight uniseriate hairs (up to 2 mm long) extended beyond the large (0.2–0.4 mm) and small (0.05–0.2 mm) hooked uncinate trichomes (scale bar: 200 µm). (C) A fifth instar S. frugiperda larva impaled and immobilized on a stem of D. intortum by both large and small uncinate trichomes. (D) Fourth instar S. frugiperda larva pierced by uncinate trichomes (red arrows). Trichomes either immobilized larvae or broke off from the basal cell with the tip remaining in the larval body causing severe wounds. (E) Distribution of non-glandular trichomes on different parts of the D. intortum plant. The relative abundance was calculated as the mean of trichome count divided by the sum of trichomes per trichome type across samples. Black circles indicate the standard error of relative trichome abundance (n = 5). (F) SEM images combining energy-dispersive X-ray spectroscopy (EDX) element topography images indicate relative surface silica (Si) distribution (red) of uniseriate, large, and small uncinate trichomes (n = 5) (Figure 1). Spodoptera littoralis larvae and adult Spodoptera frugiperda immobilized on Desmodium intortum and Desmodium uncinatum stems.
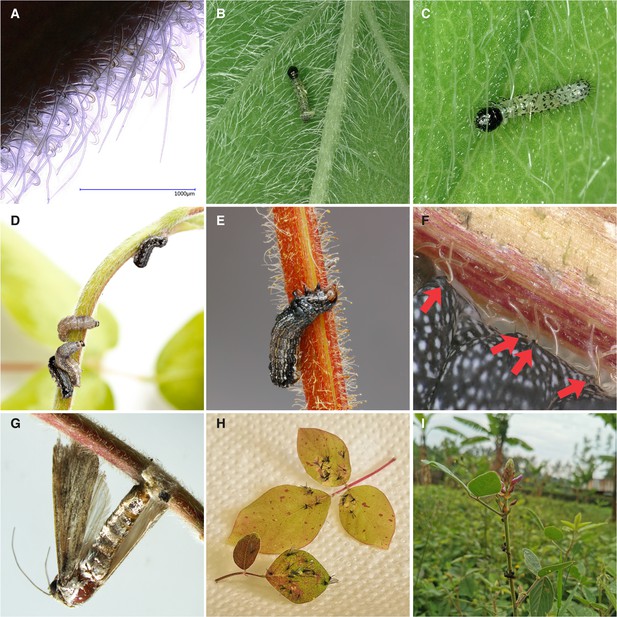
Spodoptera littoralis larvae and adult Spodoptera frugiperda immobilized on Desmodium intortum and Desmodium uncinatum stems.
(A) Light microscopic picture of trichomes on the stem of D. intortum. (B, C) Despite the dense network of sharp, straight, and hooked trichomes, neonate larvae of Spodoptera spp. are able to graze and easily navigate through the leaf surfaces of D. intortum. (D, E) Immobilized S. littoralis larvae on stems of D. uncinatum and on D. intortum stems. (F) The cuticle of an S. littoralis larva pierced by uncinate trichomes, the red arrows indicate puncture sites. (G) Ovipositing S. frugiperda female immobilized on D. intortum. (H) Bradysia sp. immobilized on D. intortum leaves. (I) Hymenopteran insects immobilized on D. intortum stems at a volatile collection site in Mwanza, Tanzania.
Tables
Volatile collection sites and environmental conditions.
Sampling site | Practice | GPS coordinates | Relative humidity (%) | Temperature (°C) |
---|---|---|---|---|
Kitagasembe village, Gwitiriyo ward, Tarime district, Mara region, Tanzania | D. intortum monoculture, Maize with common beans as intercrop (Phaseolus vulgaris) | −1.3, 34.4792 | 70 | 20–22 |
Gwitiriyo, Gwitiriyo ward, Tarime district, Mara region | D. intortum monoculture | −1.266661, 34.488133 | 68 | 20–22 |
Kyoruba village, Pemba Ward, Tarime District | D. intortum monoculture | −1.318, 34.520 | 75 | 20–22 |
Vi Agroforestry center, Lubango Ward, Musoma District, Mara region | Push–pull farming with D. intortum intercropping | −1.53054, 33.857955 | 81 | 20–22 |
RUCID centre, Mityana district, Uganda | D. intortum monoculture and maize monoculture | 0.437941, 32.042500 | 68 | 26 |
Identification of volatile components from field and laboratory volatile collection.
Compounds were tentatively identified by matching their mass spectra with those found in MS Libraries (NIST11 and Wiley). The identification was verified by synthetic standards (Compound (standard)) and matching Kovats retention indices (Compound (RI)) found in literature for DB-WAX and HP-5 capillary columns.
Chemical group | Compound(RI) | CAS | DB-WAX RI(calc) | DB-WAXRI(lib) |
---|---|---|---|---|
Reference compound | nonane | 111-84-2 | 900 | 900 |
Acetate ester | isobutyl acetate | 110-19-0 | 995 | 1002 |
Acetate ester | isoamyl acetate | 123-92-2 | 1114 | 1126 |
Acetate ester | (Z)-3-hexenyl acetate | 3681-71-8 | 1316 | 1320 |
Acetate ester | (Z)-2-hexenyl acetate | 56922-75-9 | 1332 | 1319 |
Primary alcohol | 1-propanol | 71-23-8 | 1026 | 1035 |
Primary alcohol | 1-butanol | 71-36-3 | 1122 | 1136 |
Primary alcohol | (Z)-3-hexen-1-ol | 928-96-1 | 1382 | 1387 |
Secondary alcohol | 3-hexanol | 623-37-0 | 1189 | 1189 |
Secondary alcohol | 3-octanol | 589-98-0 | 1388 | 1396 |
Dialkyl ketone | 3-hexanone | 589-38-8 | 1044 | 1042 |
Dialkyl ketone | 2-heptanone | 110-43-0 | 1185 | 1184 |
Dialkyl ketone | 3-octanone | 106-68-3 | 1262 | 1248 |
Methyl ketone | 6-methyl-5-heptene-2-one | 110-93-0 | 1332 | 1341 |
Aliphatic aldehyde | (E)-2-hexenal | 6728-26-3 | 1224 | 1218 |
Aliphatic aldehyde | ctanal | 124-13-0 | 1280 | 1287 |
Saturated fatty aldehyde | nonanal | 124-19-6 | 1398 | 1396 |
Saturated fatty aldehyde | decanal | 112-31-2 | 1490 | 1498 |
Monocarboxylic acid | acetic acid | 64-19-7 | 1410 | 1410 |
Monocarboxylic acid | butanoic acid | 107-92-6 | 1614 | 1612 |
Monocarboxylic acid | pivalic acid | 75-98-9 | 1566 | 1579 |
Aaromatic hydrocarbon | toluene | 108-88-3 | 1027 | 1037 |
Aromatic hydrocarbon | styrene | 100-42-5 | 1254 | 1254 |
Benzaldehyde | benzaldehyde | 100-52-7 | 1537 | 1528 |
Benzoate ester | methyl salicylate | 119-36-8 | 1783 | 1778 |
Monoterpene | (E)-alloocimene | 14947-20-7 | 1404 | 1396 |
Monoterpene | 3-carene | 13466-78-9 | 1148 | 1142 |
Monoterpene | p-cymene | 99-87-6 | 1277 | 1265 |
Monoterpene | (E)-4,8-dimethylnona-1,3,7-triene | 19945-61-0 | 1303 | 1302 |
Monoterpene | limonene | 138-86-3 | 1211 | 1200 |
Monoterpenoid | linalool | 78-70-6 | 1531 | 1540 |
Monoterpene | β-myrcene | 123-35-3 | 1166 | 1165 |
Monoterpene | (E)-β-ocimene | 3779-61-1 | 1251 | 1250 |
Monoterpene | (Z)-β-ocimene | 3338-55-4 | 1233 | 1234 |
Monoterpene | α-pinene | 80-56-8 | 1015 | 1015 |
Monoterpene | γ-terpinene | 99-85-4 | 1242 | 1250 |
Monoterpene | β-pinene | 127-91-3 | 1161 | 1136 |
Monoterpenoid | monoterp2 | - | 1164 | - |
Monoterpenoid | monoterp3 | - | 1230 | - |
Monoterpenoid | monoterp4 | - | 1252 | - |
Monoterpenoid | (Z)-4,8-dimethylnona-1,3,7-triene | - | 1262 | 1274 |
Monoterpenoid | monoterp6 | - | 1272 | - |
Monoterpenoid | monoterp7 | - | 1277 | - |
Monoterpenoid | monoterp8 | - | 1297 | |
Monoterpenoid | monoterp9 | - | 1305 | - |
Monoterpenoid | monoterp10 | - | 1306 | - |
Monoterpenoid | monoterp11 | - | 1308 | - |
Monoterpenoid | monoterp12 | - | 1315 | - |
Monoterpenoid | monoterp13 | - | 1371 | - |
Monoterpenoid | monoterp14 | - | 1376 | - |
Monoterpenoid | monoterp15 | - | 1399 | - |
Monoterpenoid | monoterp16 | - | 1405 | - |
Sesquiterpene | β-bisabolene | 495-61-4 | 1740 | 1727 |
Sesquiterpene | β-caryophyllene | 87-44-5 | 1619 | 1604 |
Sesquiterpene | (E)-β-farnesene | 18794-84-8 | 1668 | 1665 |
Sesquiterpene | germacrene D | 23986-74-5 | 1744 | 1746 |
Sesquiterpene | α-humulene | 6753-98-6 | 1699 | 1690 |
Sesquiterpenoid | sesquiterp1 | - | 1493 | - |
Sesquiterpenoid | sesquiterp2 | - | 1498 | - |
Sesquiterpenoid | cyclosativene | 22469-52-9 | 1500 | 1490 |
Sesquiterpenoid | α-copaene | 3856-25-5 | 1503 | 1497 |
Sesquiterpenoid | ylangene | 14912-44-8 | 1523 | 1499 |
Sesquiterpenoid | sesquiterp6 | - | 1533 | - |
Sesquiterpenoid | sesquiterp7 ((Z)-α-bergamotene) | 18252-46-5 | 1547 | 1555 |
Sesquiterpenoid | α-cedrene | 469-61-4 | 1552 | 1565 |
Sesquiterpenoid | sesquiterp9 | - | 1561 | - |
Sesquiterpenoid | sesquiterp10 | - | 1566 | - |
Sesquiterpenoid | sesquiterp11 | - | 1588 | - |
Sesquiterpenoid | α-santalene | 512-61-8 | 1591 | 1597 |
Sesquiterpenoid | sesquiterp13 | - | 1594 | - |
Sesquiterpenoid | sesquiterp14 | - | 1607 | - |
Sesquiterpenoid | sesquiterp15 | - | 1648 | - |
Sesquiterpenoid | (Z)-β-farnesene | 28973-97-9 | 1653 | 1652 |
Sesquiterpenoid | α-himachalene | 3853-83-6 | 1657 | 1649 |
Sesquiterpenoid | sesquiterp18 | - | 1658 | - |
Sesquiterpenoid | sesquiterp19 | - | 1665 | - |
Sesquiterpenoid | sesquiterp20 | - | 1678 | - |
Sesquiterpenoid | γ-curcumene | 28976-68-3 | 1704 | 1695 |
Sesquiterpenoid | sesquiterp22 | - | 1705 | - |
Sesquiterpenoid | sesquiterp23 | - | 1717 | - |
Sesquiterpenoid | β-curcumene | 28976-67-2 | 1753 | 1744 |
Sesquiterpenoid | sesquiterp25 | - | 1768 | - |
Sesquiterpenoid | α-curcumene | 644-30-4 | 1784 | 1773 |
Sesquiterpenoid | sesquiterp27 | - | - | - |
Sesquiterpenoid | (E,E)-4,8,12-trimethyltrideca-1,3,7,11-tetraene | 62235-06-7 | 1809 | - |
Sesquiterpenoid | cadine-1,4-diene | 16728-99-7 | 1816 | 1802 |
Sesquiterpenoid | sesquiterp30 | - | 1972 | - |
Sesquiterpenoid | sesquiterp31 | - | 2020 | - |
Sesquiterpenoid | sesquiterp32 (β-caryophyllene oxide) | 1139-30-6 | 2023 | 2013 |
Sesquiterpenoid | sesquiterp33 | - | 2036 | - |
Sesquiterpenoid | sesquiterp34 | - | 2075 | - |
Sesquiterpenoid | sesquiterp35 | - | 2139 | - |
Sesquiterpenoid | sesquiterp36 | - | 2175 | - |
Sesquiterpenoid | sesquiterp37 | - | 2269 | - |
Unknown | butyl acetate | 123-86-4 | 1054 | 1059 |
Unknown | comp2 | - | 1114 | - |
Unknown | comp3 | - | 1163 | - |
Unknown | comp4 | - | 1183 | - |
Unknown | butyl butanoate | 109-21-7 | 1213 | 1221 |
Unknown | 5-hepten-2-one | 6714-00-7 | 1253 | 1249 |
Unknown | 2-heptanol | 543-49-7 | 1316 | 1315 |
Unknown | trimethyl-cyclohexanone | 2408-37-9 | 1333 | 1335 |
Unknown | anisole | 100-66-3 | 1344 | 1340 |
Unknown | comp10 | - | 1380 | - |
Unknown | comp11 | - | 1393 | - |
Unknown | comp12 | - | 1399 | - |
Unknown | comp13 | - | 1414 | - |
Uknown | comp14 | - | 1442 | - |
Unknown | comp15 | - | 1450 | - |
Unknown | comp16 | - | 1569 | - |
Suppliers and purity of synthetic standard compounds.
The synthetic standards were injected in DB-WAX and HP-5 columns to verify identification of headspace volatile components.
Compound | CAS | Supplier | Purity |
---|---|---|---|
(E)-alloocimene | 673-84-7 | Sigma-Aldrich | 80% |
β-bisabolene | 495-61-4 | preparative GC | 1 µg/µl |
camphene | 79-92-5 | Sigma-Aldrich | 95% |
3-carene | 13466-78-9 | Sigma-Aldrich | 90% |
β-caryophyllene | 87-44-5 | Sigma-Aldrich | ≥98.0% |
β-caryophyllene oxide | 1139-30-6 | Sigma-Aldrich | 95% |
α-cedrene | 11028-42-5 | Sigma-Aldrich | 95% |
α-cubebene | 17699-14-8 | preparative GC | 1 µg/µl |
m-cymene | 535-77-3 | Sigma-Aldrich | 99% |
p-cymene | 99-87-6 | Sigma-Aldrich | 99% |
α-farnesene | 502-61-4 | Sigma-Aldrich | 95% |
(Z)-farnesol | 106-28-5 | Sigma-Aldrich | 95% |
(Z)-β-farnesene | 28973-97-9 | Sigma-Aldrich | 99% |
(E)-β-farnesene | 18794-84-8 | preparative GC | 1 µg/µl |
germacrene D | 23986-74-5 | preparative GC | 1 µg/µl |
isobutyl acetate | 110-19-0 | Sigma-Aldrich | ≥98.0% |
isoamyl acetate | 123-92-2 | Sigma-Aldrich | 99% |
3-hexanone | 589-38-8 | Sigma-Aldrich | ≥97% |
1-hexanol | 111-27-3 | Sigma-Aldrich | 99% |
2-heptanone | 110-43-0 | Sigma-Aldrich | 99% |
(E)-2-hexenal | 6728-26-3 | Fluka | 99% |
(Z)-3-hexen-1-yl acetate | 928-96-1 | Sigma-Aldrich | 98% |
β-humulene | 116-04-1 | preparative GC | 1 µg/µl |
γ-humulene | 6753-98-6 | Sigma-Aldrich | 85% |
limonene | 5989-27-5 | Sigma-Aldrich | 97% |
linalool | 78-70-6 | Sigma-Aldrich | 97% |
methyl jasmonate | 1211-29-6 | Sigma-Aldrich | 98% |
methyl salicylate | 119-36-8 | Sigma-Aldrich | 99% |
β-myrcene | 123-35-3 | Sigma-Aldrich | ≥90.0% |
nonane | 111-84-2 | Fluka | 99% |
nonanal | 124-19-6 | Sigma-Aldrich | 95% |
β-(E)-ocimene | 13877-91-3 | Sigma-Aldrich | 70% |
1-octen-3-ol | 3391-86-4 | Fluka | 98% |
3-octanone | 106-68-3 | Sigma-Aldrich | 99% |
3-octanol | 589-98-0 | Sigma-Aldrich | 99% |
α-pinene | 86-56-8 | Sigma-Aldrich | 97% |
β-pinene | 18172-67-3 | Sigma-Aldrich | 99% |
α-phellandrene | 99-83-2 | Sigma-Aldrich | 85% |
α-terpinene | 99-86-5 | Sigma-Aldrich | 95% |
γ-terpinene | 99-85-4 | Sigma-Aldrich | 97% |
experiment | specimen | Sobhy et al. | manuscript |
---|---|---|---|
volatile collection | Desmodium | no information | 6- 8 weeks |
wind tunnel oviposition | Desmodium | 11 weeks | |
wind tunnel oviposition | Maize | 4-5 weeks | |
cage oviposition | Desmodium | 4-5 weeks | 8 weeks |
cage oviposition | Maize | 2-3 weeks | 3-4 weeks |
wind/cage | S. frugiperda | 2 days old mated | 6 days old mated |