Connecting the dots: Managing species interaction networks to mitigate the impacts of global change
Figures
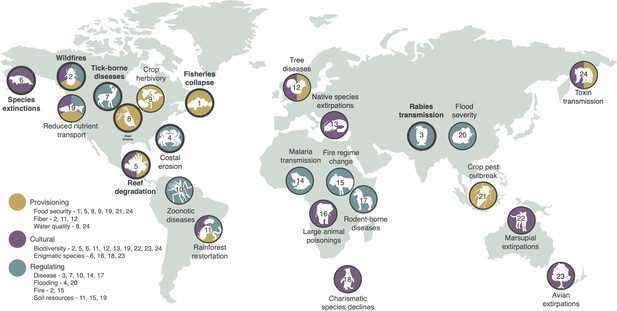
Examples of global change altering networks of species interactions, impacting key species or groups of species that provide ecosystem services.
Four descriptors are provided for each case study: (1) the disrupted ecosystem service is listed (icon text); (2) colors depict the class or classes of ecosystem services (provisioning, cultural, or regulating); (3) an icon depicts the key species (or group of species) that is the ecosystem service (or dis-service) provider driving the effect; and (4) numbers refer to network diagrams showing how altered interaction networks resulted in the disruption of the ecosystem service. Highlighted case studies (bold text and circles) are described in text below with network diagrams provided in Figure 3, representing case studies that provide provisioning services (#1, #2), regulating services (#3, #4), and cultural services (#5, #6), as well as in Figure 4, representing case studies of successful network management (#7, #8). Explanations of other case studies (descriptions, network diagrams) are provided in Appendix 1. Where case studies address multiple classes of ecosystem service, subsequent figures (Figure 3 and Appendix 1) highlight a single class.
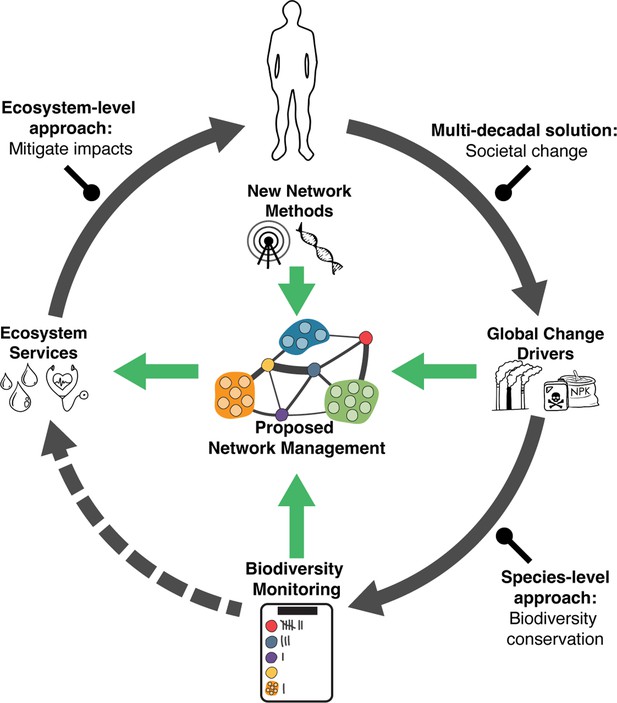
Linkages between global change drivers, societal responses to their impacts, ecosystem services, and human prosperity and wellbeing.
Global change drivers (e.g., CO2, fertilizers, other pollutants) are recognized to affect both biodiversity (e.g., endangered species), and the ecosystem services (e.g., air and water quality) that underlie human wellbeing (solid/curved/gray arrows). Societal responses to these impacts include multi-decadal efforts to transform to sustainable socio-economic systems, biodiversity conservation, or restoration, and attempts to mitigate the impacts of degraded ecosystem services on humans (straight/rounded head/black arrows). While biodiversity monitoring has been an important component of conservation, there has been little connection between such efforts and the restoration of ecosystem services (dashed/curved/gray arrow). We propose the need to develop a third approach, termed network management (green arrows) using information on species interactions and the networks that emerge from them which drive the performance of key ecosystem providers. Such an approach can provide a mechanistic understanding of how global change impacts on species scale up to affect ecosystem services and in turn inform effective management solutions. Figure center: A schematic representation of an interaction network (aka food web). Individual circles represent species, lines represent interactions, and line thickness the strengths of interactions. Networks can be depicted and studied with links between individual species (single circles) or between groups of species (trophic levels or guilds; clusters of circles) that have a similar function within an ecosystem. All species within a network are ultimately linked by some combination of direct and indirect interactions.
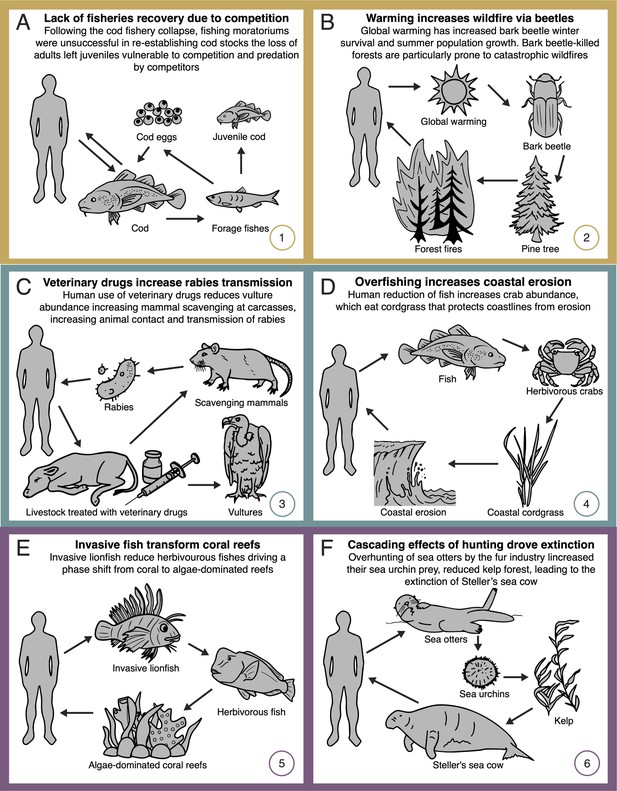
Network diagrams of global change impacts on (A, B) provisioning, (C, D) regulating, and (E, F) cultural ecosystem services through altered species interactions.
See text for detailed explanations of each case study. Numbers (bottom right corners) reference the depiction of these case studies in Figure 1. Additional examples of degraded provisioning ecosystem services are provided in Appendix 1. Some case studies address multiple classes of ecosystem service (as shown in Figure 1), but individual classes are highlighted here and in Appendix 1.
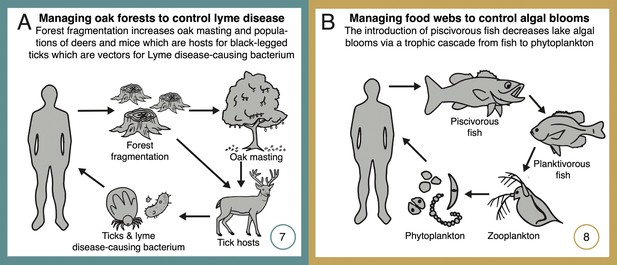
Network diagrams of systems using network management for disease prevention or forecasting (Lyme disease, A), and for trophic regulation of ecosystem productivity for fisheries and recreation (temperate lakes, B).
See text for detailed explanations of each case study. Numbers (bottom right-hand corners) reference the depiction of these case studies in Figure 1. Some case studies address multiple classes of ecosystem service (as shown in Figure 1), but individual classes are highlighted here and in Figure 3 and in Appendix 1.
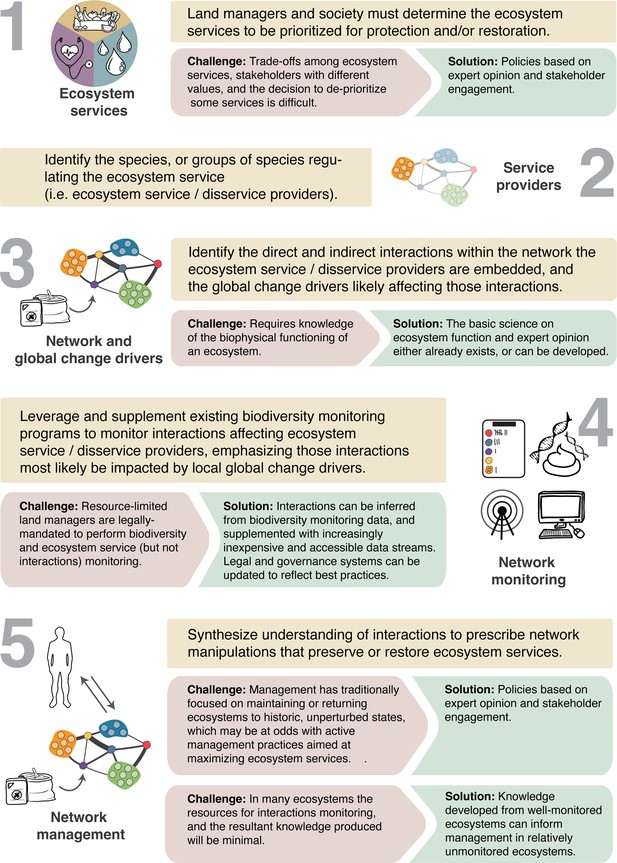
A general framework for developing network management.
We outline the challenges to each of the five fundamental steps for transitioning to network management and suggest solutions in each case.
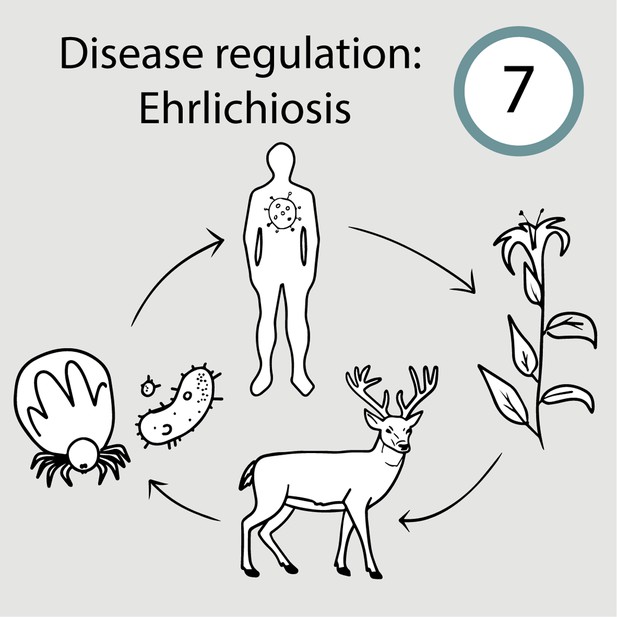
The risk of tick-borne diseases increases when deer congregate in patches of invasive honeysuckle (Case study 7 in manuscript Figure 1).
Honeysuckle is an invasive woody shrub in the Northeastern United States that forms dense understory patches. Deer preferentially use these dense patches for increased shelter, protection from predators, and food (Allan et al., 2010). Deer are also the primary host for the lone star tick (Amblyomma americanum) and are a reservoir of bacterial pathogens. The high density of deer in honeysuckle patches results in a higher proportion of tick blood-meals from deer, and a greater prevalence of ticks infected with Ehrlichia chaffeensis (agents of human ehrlichiosis) (Allan et al., 2010). Therefore, the honeysuckle invasion ultimately increases the risk of human disease via altered interactions between plants, deer, ticks, and bacterial pathogens.
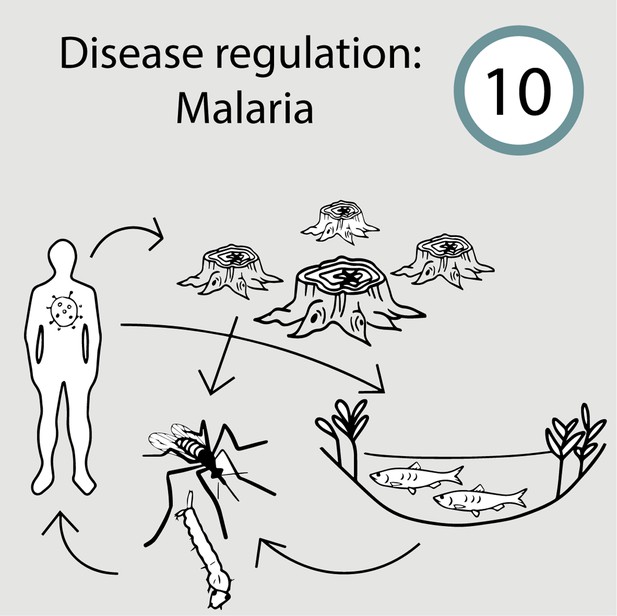
Malaria transmission rates increase as deforestation creates mosquito habitat (Case study 10 in manuscript Figure 1).
Malaria infection has long been a critical health issue in Amazonian Brazil. There were 6 million cases annually in the 1940s, but control efforts were able to reduce cases to <50,000 by the early 1960s. Since then, there has been a resurgence of malaria largely driven by deforestation and land-use changes, altering mosquito–human interactions (MacDonald and Mordecai, 2019). Deforestation can increase infectious disease transmission by expanding the spatial overlap between people and wildlife disease reservoirs (Jones et al., 2008). In this case, not only are frontier settlements encroaching on mosquito habitat, but deforestation itself creates more mosquito breeding habitat near human settlements. The mosquitoes spreading malaria proliferate in larger ponds with more algae, leaf litter, and emergent grasses – conditions characteristic of fish farms created by settlers (Vittor et al., 2009). This case study exemplifies how environmental change affects human health, and how shifts in species interactions can mediate these effects.
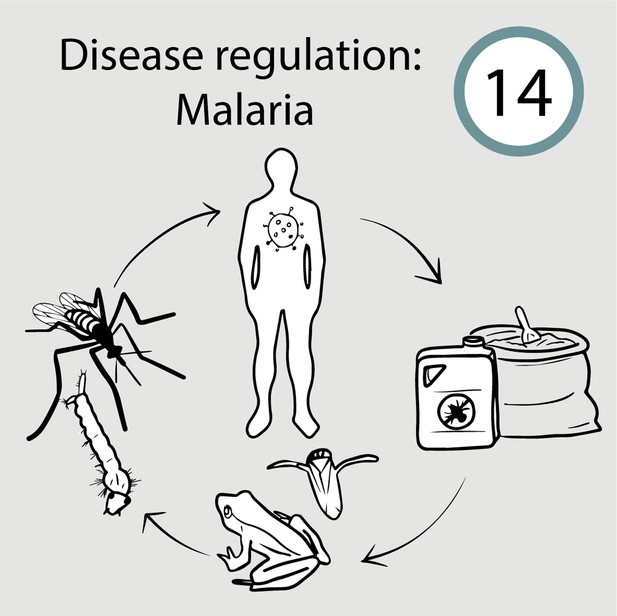
Insecticides can harm aquatic predators, indirectly increasing mosquito abundance and malaria transmission (Case study 14 in manuscript Figure 1).
Efforts to control malaria, a threat to half of the world’s population (World Health Organization, 2020), can backfire when insecticides are used against malaria-transmitting mosquitoes. While these compounds may suppress mosquitoes directly, they also negatively affect fish and predatory insects that would otherwise control mosquito populations, paradoxically leading to mosquito and malaria outbreaks (Roux and Robert, 2019). This example demonstrates that disease regulation requires understanding how disease vectors are embedded in complex interaction networks that, if disrupted, can have large consequences for human health.
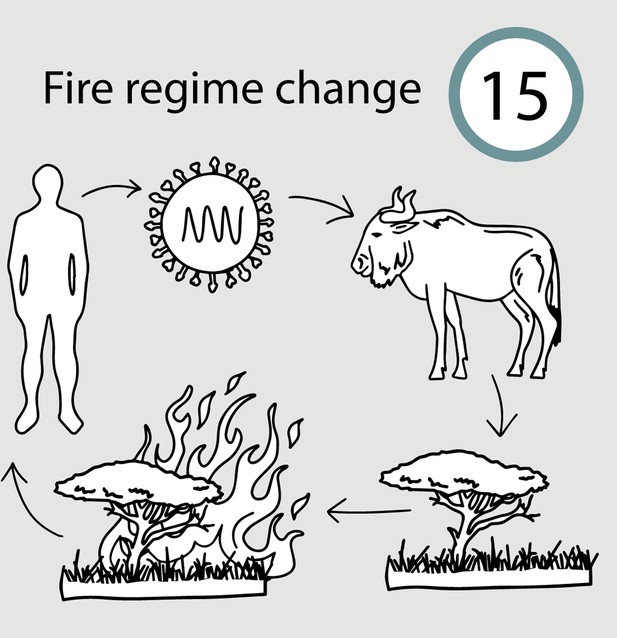
Eradication of rinderpest increased wildebeest abundance and grazing, reducing fire frequency, and increasing C storage (Case study 15 in manuscript Figure 1).
Rinderpest is an infectious viral disease that infects ungulates. The virus arrived in sub-Saharan Africa in 1887 with the import of infected cattle. Following the introduction, rinderpest spread to domesticated and wild ungulates, causing widespread ungulate mortality and famine. In the 1960s, a successful vaccination program eradicated rinderpest. The abundance of many ungulates, particularly wildebeest, rapidly increased without this source of mortality. The increase in wildebeest grazing on the Serengeti reduced fuel loads for fires, decreased fire frequency, and increased tree density. This shift was so significant that the Serengeti switched from a carbon source to a carbon sink (Holdo et al., 2009).
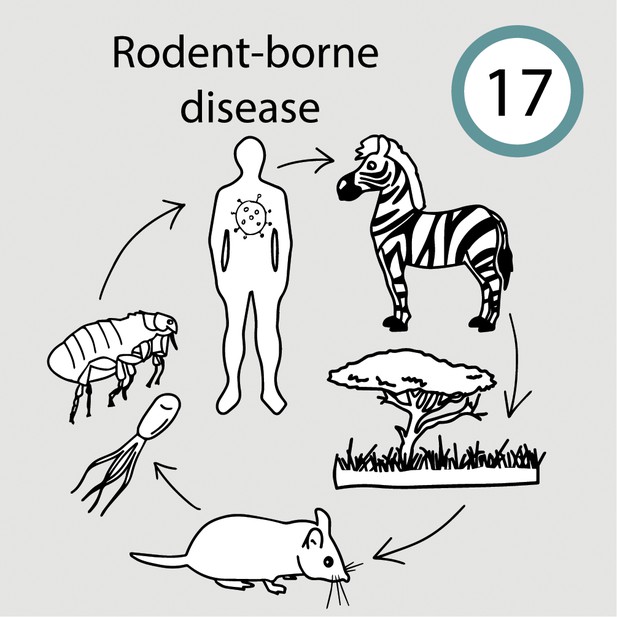
The extirpation of large wildlife alters vegetation, increasing the abundance of rodents and fleas and the risk of bartonellosis (Case study 17 in manuscript Figure 1).
Large-bodied species are at a high risk of extinction, and their loss may have cascading impacts on other trophic levels (Ripple et al., 2017). Large herbivores are declining across African savannas due to land-use change and hunting. The loss of large herbivores has caused an increase in rodent populations through both direct and indirect pathways. Large herbivores directly compete with small mammals for food, so their extirpation reduces competition, allowing rodent populations to proliferate (Keesing, 2000). Simultaneously, their extirpation results in large vegetation structural changes that may also favor small mammals (Young et al., 2013). The increase in rodent abundance results in a higher abundance of their ectoparasites (e.g., fleas) and the pathogens they carry (e.g., Bartonella), ultimately increasing the risk of disease in humans (Young et al., 2014).
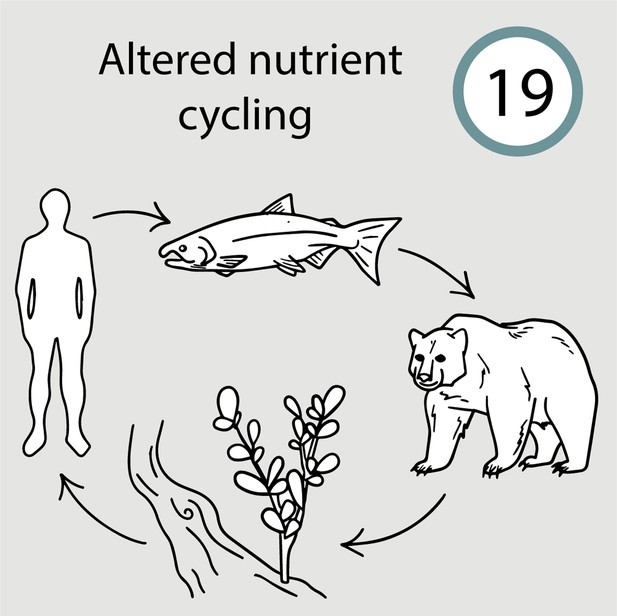
Overfishing salmon reduces soil nutrients due to the reduction of bear-mediated nutrient movement (Case study 19 in manuscript Figure 1).
Climate change and overfishing reduce pacific salmon populations (Oncorhynchus spp.) with unexpected indirect effects on inland ecosystems. Migrating salmon spawn and die in inland rivers and streams, providing large nutrient inputs to adjacent forests from carcasses or feces from bears (Ursus spp.) and other salmon predators, such that declines in salmon populations are causing reductions in soil fertility and shifts in plant communities (Hocking and Reynolds, 2011). By recognizing these indirect effects, management practices are now starting to consider networks affecting ecosystem service or disservice providers that determine soil erosion, fertility, and other associated services.
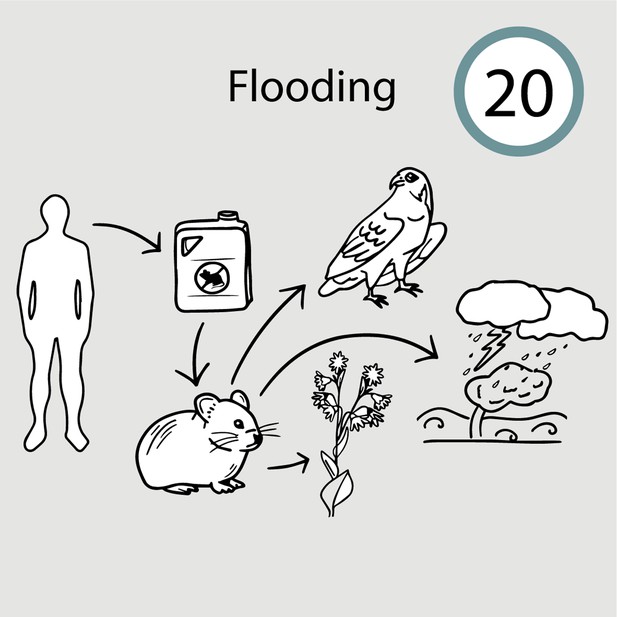
The poisoning of pikas leads to a loss of avian predators, changing soil water retention, and increasing flood severity (Case study 20 in manuscript Figure 1).
The plateau pika (Ochotona curzoniae) is a keystone species and ecosystem engineer in the Qinghai–Tibetan Plateau region (Smith et al., 2019). The plateau pikas are important prey for avian and mammalian predators and dig burrows that nesting birds use. Burrowing also increases local plant diversity and soil water retention. Because pikas compete for food with local livestock, there have been on-going pika poisoning programs since the 1960s, poisoning pikas across hundreds of thousands of square kilometers at the cost of over $100 million USD. The loss of pika has had cascading impacts on other species and the ecosystem – their predators have been extirpated, plant diversity reduced, and soil water infiltration decreased. The eradication of the perceived pests has ultimately degraded cultural (biodiversity) and regulating (flood control) ecosystem services.
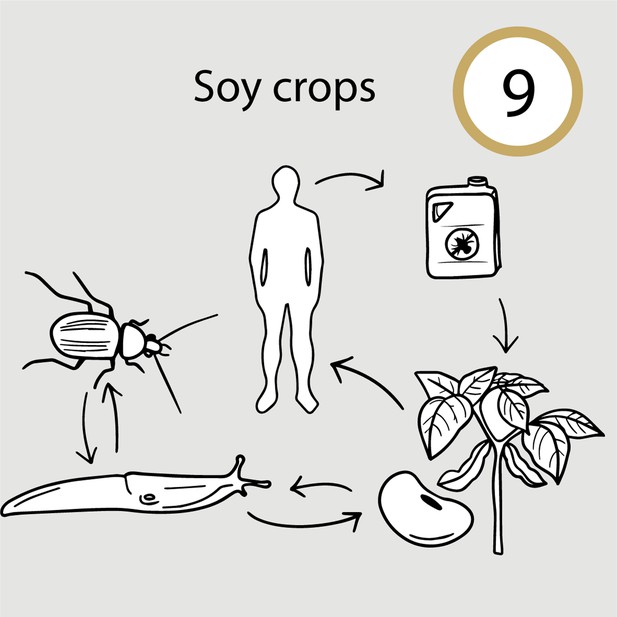
Insecticides applied to soybeans concentrate in slugs killing their predators ultimately increasing slug herbivory (Case study 9 in manuscript Figure 1).
Agricultural practices, such as using insecticides, can alter species interactions affecting the services provided by agroecosystems. Neonicotinoids are the most common insecticide used across the globe to reduce pests in agricultural landscapes and increase crop yield. These insecticides are often coated onto seeds, protecting seeds and early stages of the plant as they are absorbed into tissues as the plant germinates and grows. Soybeans are one of the crops that routinely receive a neonicotinoid seed coating in North American agriculture. Although the goal of the insecticide application is to decrease herbivory, it has the opposite effect because of a series of species interactions. First, slugs eat neonicotinoids. They are not affected by the insecticide, and instead, the toxin is concentrated in their tissues. Next, slug predators are killed by the insecticide when they eat slugs, reducing the abundance of slug predators. The insecticides, therefore, increase herbivory by weakening pest biocontrol, ultimately reducing crop yields (Douglas et al., 2015).
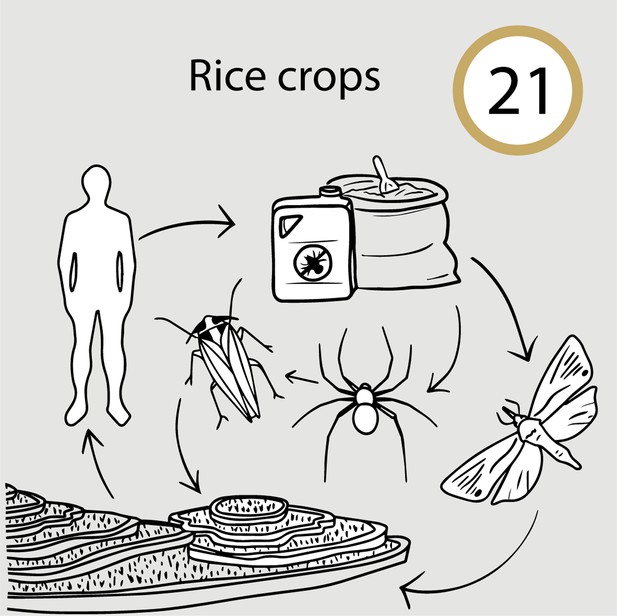
Pesticides applied to rice reduced competition and predation on a herbivore pest, increasing herbivore damage of rice (Case study 21 in manuscript Figure 1).
In Indonesia, the use of pesticides against an economically important insect herbivore of rice, the yellow stem borer (Scirpophaga incertulas), unexpectedly created an even greater pest problem. Paradoxically, the suppression of the stem borer boosted populations of a secondary pest, the brown planthopper (Nilaparvata lugens), which is less susceptible to pesticides. Insecticides benefited the brown plant hopper by reducing competition with the stem borer, and by killing predatory arthropods that had previously held it in check (Heinrichs and Mochida, 1984; Settle et al., 1996). Such unintended impacts of insecticides are leading to agricultural practices that reduce pesticide use in favor of practices that seek to conserve networks of interactions that sustain these ecosystem service providers.
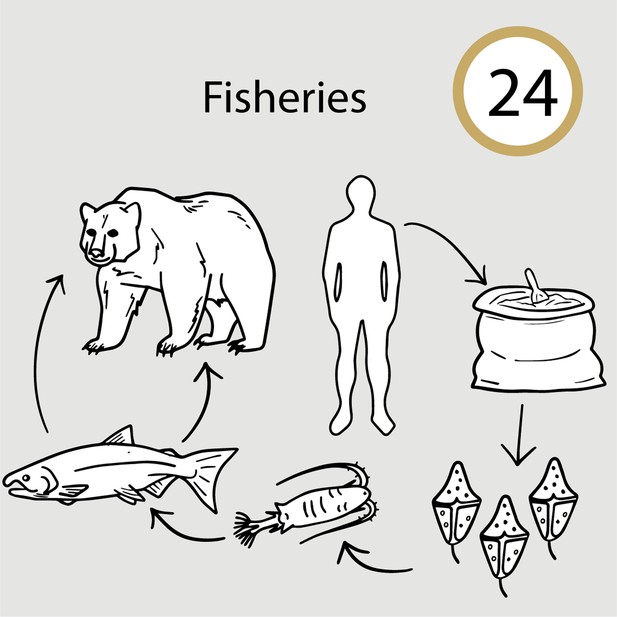
Terrestrial, marine mammals, and fish die when they consume prey containing toxins produced by fertilizer-fueled algal blooms (Case study 24 in manuscript Figure 1).
Humans have dramatically accelerated nitrogen (Galloway et al., 2008) and phosphorus (Van Vuuren et al., 2010) cycles. Nutrients are transported by runoff water from terrestrial systems into lakes and rivers (Carpenter et al., 1998), eventually making their way to coastal ecosystems and oceans (Howarth et al., 1996). Upon reaching waterways, nutrients promote excessive plant growth and algal blooms (i.e., cultural eutrophication) which can impact aquatic ecosystems in various ways. Nutrient inputs increase the prevalence of harmful algal blooms that produce high levels of phycotoxins that affect organisms at higher trophic levels (Anderson et al., 2012). Toxic algae are consumed by zooplankton which are then fed upon by higher trophic level organisms such as fish. Fish may be directly killed by phycotoxins, or they may survive and in turn kill mammals and birds that prey on these fish (Landsberg, 2002). These findings teach us that to mitigate eutrophication effects on ecosystem function, we need to understand how its effects are transmitted through food webs via species interactions. Doing so will provide the scientific basis for protecting aquatic food webs, conserving and managing species of economic importance, and improving human wellbeing.
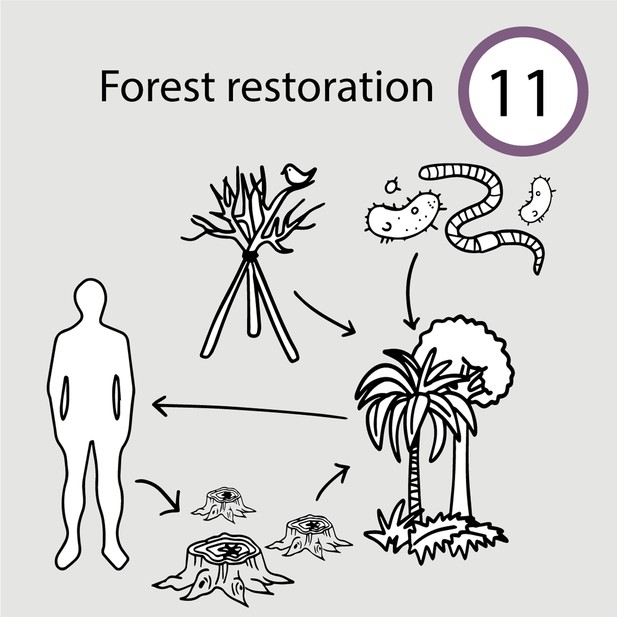
Rainforest restoration benefits from perches for birds and bats, shelters for reptiles and small mammals, and topsoil containing microbes, earthworms, and fungi (Case study 11 in manuscript Figure 1).
Tropical rainforests have experienced deforestation for decades. Conservation efforts are increasingly focused on restoration efforts, turning deforested areas back into diverse and functioning ecosystems that provide many ecosystem services (e.g., biodiversity, species used in medicine). The most common restoration technique is creating tree plantations. Plantations vary in how successful they are at restoring fully functional ecosystems (Guariguata and Ostertag, 2001). Instead, techniques that consider species interactions and use a network approach have been more predictably successful (Bechara et al., 2016). One such approach is nucleation, where animals that directly and indirectly interact with plants are added in addition to planting trees. Successful additions include (1) Soil relocation from natural remnants to reestablish edaphic communities; (2) Seed banks from natural forest remnants; (3) Artificial perches for birds to use; (4) Artificial shelters for reptiles and small mammals. By nucleating the recovery of species that interact with rainforest plants, the ecosystem is able to restore ecosystem services more frequently and quickly than those that ignore species interactions (Bechara et al., 2016).
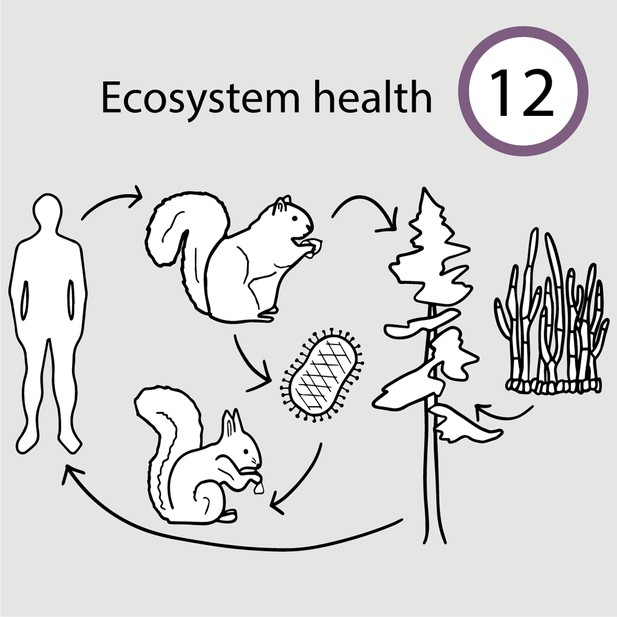
Invasive gray squirrels out-compete and infect native red squirrels and increase fungal infections on timber trees through bark peeling (Case study 12 in manuscript Figure 1).
Eastern gray squirrels are invasive across Europe, having been introduced from the Americas. Eastern gray squirrels thrive in oak and beech forest and urban environments and have been outcompeting the native red squirrel for habitat and food. Eastern gray squirrels may also be the source of a disease caused by parapoxvirus that kills native squirrels. The introduction of gray squirrels not only threatens the survival of native red squirrels but is a problem for commercial hardwood timber production (Bruemmer et al., 2000). Eastern gray squirrels pull the bark off trees resulting in wounds that severely decrease the value of the wood. The wound sites are also susceptible to fungal infections which can kill the trees (Bruemmer et al., 2000). Therefore, the invasive Eastern gray squirrel affects ecosystem services via altered species interactions, decreasing native biota (cultural ecosystem service), and negatively affecting the timber industry (provisioning service).
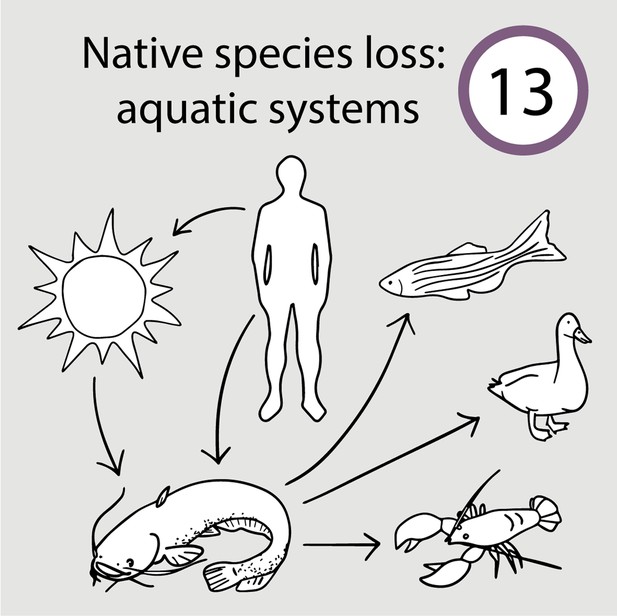
Alien fish species in Southern European rivers reduce native waterbirds, fish, and invertebrates, and are spreading as the climate warms (Case study 13 in manuscript Figure 1).
The European catfish (Silurus glanis) is the largest-bodied freshwater fish of Europe and has a broad diet based mainly on other smaller fishes. It has been introduced to many areas in Europe outside its native range, mainly for recreational purposes (fishing) and is now posing a threat to local stream and river ecosystems across many countries and the services they provide (Copp et al., 2009). By feeding on native fishes, it drives changes in the structure of aquatic food webs, including changes in species composition and declines in abundance of both native vertebrate and invertebrate species (Carol et al., 2009). Impacts also include negative direct (and potentially also indirect) effects on waterbirds and other terrestrial species associated with riparian systems (Carol et al., 2009). Further, because this species is more prone to invade and successfully establish under warmer conditions, climate change-driven warming favors its invasion into southern regions such as the Mediterranean (Britton et al., 2010), amplifying the local strength and spatial extent of its ecological impacts.
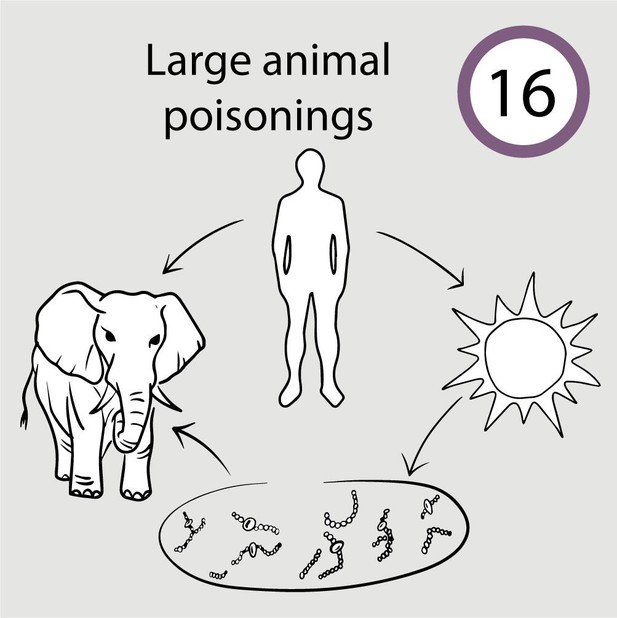
Poisonous microalgal blooms caused by warming can kill large animals at watering holes (Case study 16 in manuscript Figure 1).
Botswana is home to one of the largest populations of elephants and is considered one of the last strongholds for this declining species. Elephant abundances have rapidly declined from an estimated 10 million in the 1930s to <500 thousand today due to poaching and land-use change. Close to 400 elephants were found dead between March and June 2020 near watering holes (Veerman et al., 2022). Toxins produced by cyanobacterial blooms were the culprit, with synchronous blooms across the Okavango Delta region in Botswana for many months beginning in 2019 (Veerman et al., 2022). Such blooms are promoted by low precipitation (longer water residence times), and high temperatures – both of which occurred in 2019/2020 in this region. This is not the first time cyanobacterial blooms have killed large charismatic and endangered species at watering holes (Oberholster et al., 2009), and it is unlikely to be the last, as climate change continues to alter precipitation and increase temperatures promoting cyanobacterial dominance in freshwaters worldwide (Paerl and Huisman, 2008).
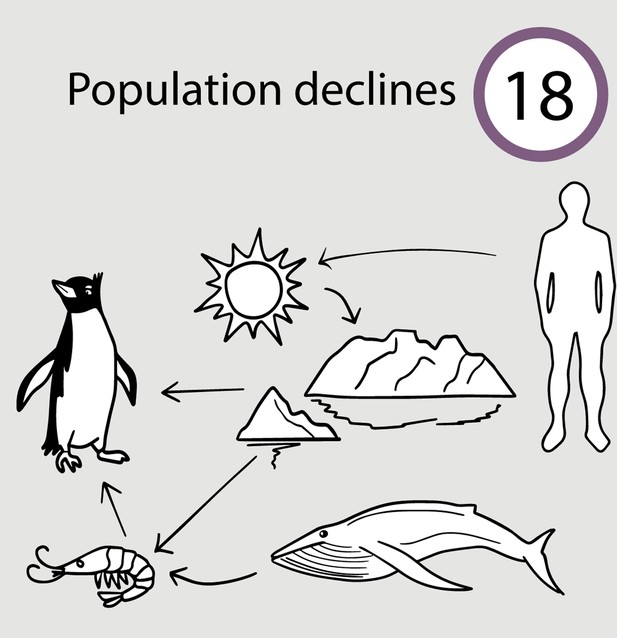
Climate warming has reduced penguin population abundance via shifts in prey biomass (Case study 18 in manuscript Figure 1).
Penguin (Pygoscelis adeliae, P. antarctica) populations in Antarctica have suffered up to 50% reductions due to the indirect effects of climate warming. Krill (small crustaceans), the main food source for penguins (Trivelpiece et al., 2011), feed upon the algae that dwell under ice sheets, which also provides the krill physical shelter. Increasing ocean temperatures have reduced ice sheet coverage, reducing krill abundance and thus affecting penguins, as well as other species such as whales, seals, and other sea birds (Piñones and Fedorov, 2016; Trivelpiece et al., 2011).
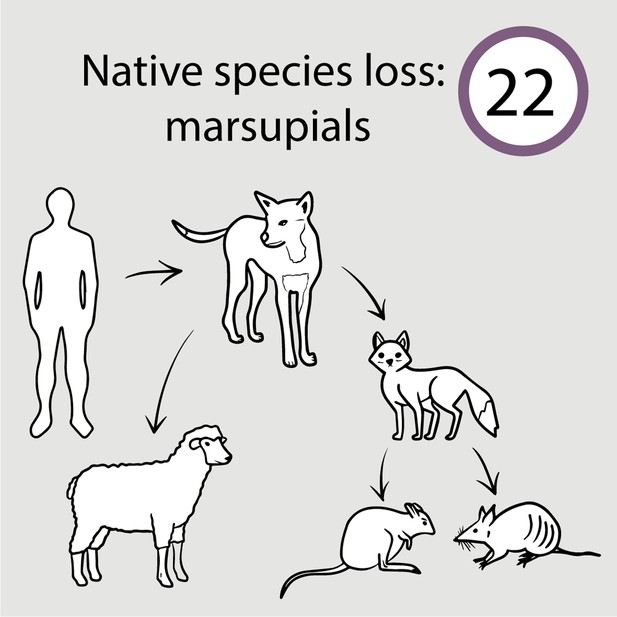
Australia’s marsupials were extirpated when dingo removal increased the abundance of invasive mesopredators (Case study 22 in manuscript Figure 1).
Concomitant with the hominid invasion, Australia lost all of its native, large-bodied (>5 kg), terrestrial predators, resulting in one of the world’s most depleted predator guilds (Johnson et al., 2007), including its largest native predator, the dingo (Canis lupus dingo). Habitat loss and hunting as a preventive measure to protect sheep herds (without prior evidence that dingoes posed a significant risk to herds), drove the eventual elimination of the dingo and unexpected consequences due to altered species interactions. The loss of the dingo led to the rise of its former prey – non-native mid-sized predators such as the red fox (Vulpes vulpes) and feral cats (Felis catus). Mid-sized predators, left unchecked, unleashed drastic population declines and extinctions of their native marsupial prey throughout Australia. This wave of marsupial extinctions was symptomatic of underlying changes in species interactions due to top-predator extirpations (Letnic et al., 2012).
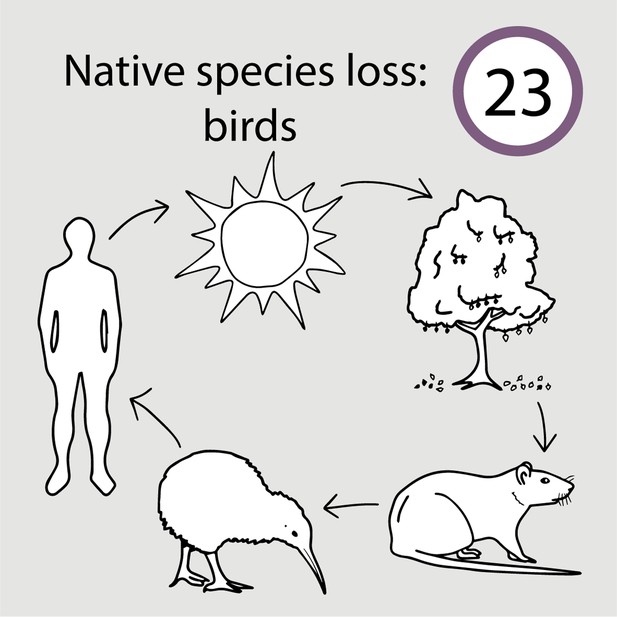
The proliferation of mice and rats following beech tree masting increases invasive stoat predation on native New Zealand birds (Case study 23 in manuscript Figure 1).
Half of New Zealand’s endemic bird species have gone extinct since human colonization of the island, and many are currently threatened (Holdaway, 1999). The introduction of bird predators such as stoats has been a major driver of bird decline (Dilks et al., 2014). A cascade of species interactions magnifies the impact of invasive predators on native bird populations. First, beech trees reproduce through masting, simultaneously releasing flowers and seeds at irregular intervals (3–5 years, Schauber et al., 2002). Second, this large pulse of resources increases the population sizes of mice and rats and the population size of their predators – the invasive stoats (Dilks et al., 2014). The increased abundance of stoats is one of the most important factors driving forest birds' decline in New Zealand (Dilks et al., 2014; Wilson et al., 1998). As temperatures rise, seed production during masting is likely to increase (Schauber et al., 2002), with the greatest impact on predator abundance, not plant fitness (Bogdziewicz et al., 2020), exacerbating the reduction of native bird populations.