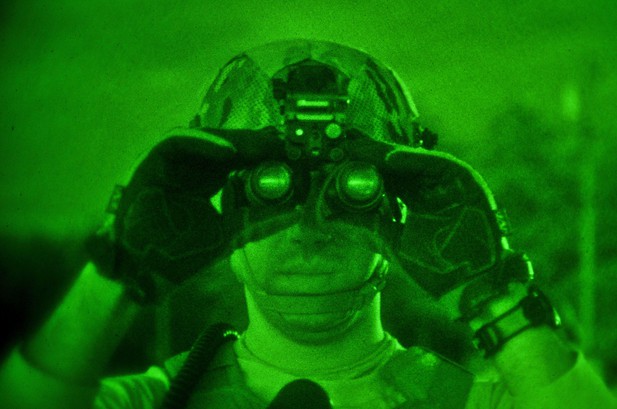
Image credit: Public domain
People can see in dim light because of cells at the back of the eye known as rods. These cells contain two key components: molecules called retinal, which are bound to proteins called rhodopsin. When light hits a rod cell, it kicks off a cascade of reactions beginning with the retinal molecule changing into an activated shape and ending with a nerve impulse travelling to the brain. The activated form of retinal is toxic, and as long as it remains bound to the rhodopsin protein it will not damage the rod or surrounding cells. The toxic retinal also cannot respond to light. It must be released from the protein and converted back to its original shape to restore dim light vision.
As with all proteins, rhodopsin’s structure comprises a chain of building blocks called amino acids. Every land animal with a backbone has the same amino acid at position 122 in its rhodopsin. This amino acid, named E122, helps to stabilize the activated rhodopsin, slowing the release of the toxic retinal. Yet E122 also makes the rod cells less sensitive, resulting in poorer vision in dim light. In contrast, some fish do not have E122 but rather one of several different amino acids takes its place. What remains unclear is why all land animals have stuck with E122, and whether there were other options that evolution could have explored to overcome the trade-off between sensitivity and stability.
By looking at the make-up of rhodopsins from many animals, Castiglione and Chang found other sites in the protein where the amino acid changed whenever position 122 changed. The amino acids at these so-called “coevolving sites” were then swapped into the version of rhodopsin that is found in cows, which had also been engineered to lack E122. These changes fully compensated for the destabilizing loss of E122 on activated rhodopsin but without sacrificing its sensitivity to light. Further experiments then confirmed that unless all amino acids were substituted at once, the activated rhodopsin was very unstable. Indeed, it was almost as unstable as mutated rhodopsins found in some human diseases. These findings suggest that, while there was in principle another solution available to land animals, the routes to it were closed off because they all came with an increased risk of eye disease.
These findings highlight that rhodopsin likely plays a more important role in protecting humans and many other land animals against eye disease than previously assumed. More knowledge about this protective role may lead to new therapies for these conditions. Also, investigating similar evolutionary trade-offs could help to explain how and why different proteins work the way that they do today.