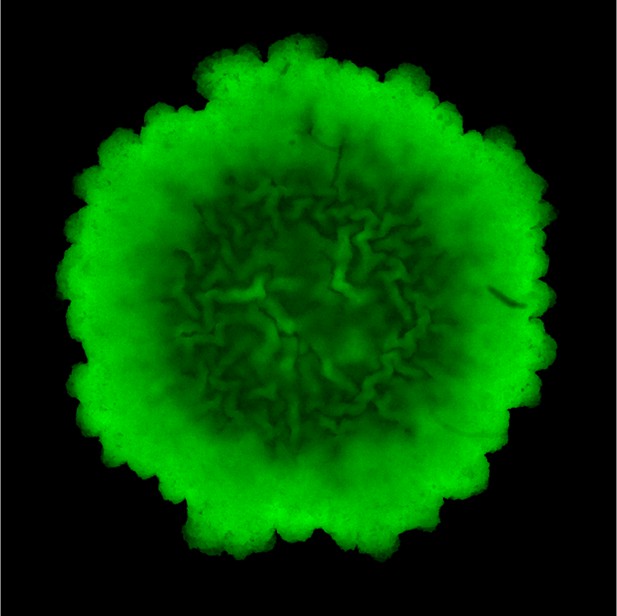
A microscopy image of a biofilm colony of the bacterium Bacillus subtilis. Image credit: Srinivasan et al. 2019 (CC BY 4.0)
Bacteria can grow and thrive in many different environments. Although we usually think of bacteria as single-celled organisms, they are not always solitary; they can also form groups containing large numbers of individuals. These aggregates work together as one super-colony, allowing the bacteria to feed and protect themselves more efficiently than they could as isolated cells.
These colonies move and grow in characteristic patterns as they respond to their environment. They can form swarms, like insects, or biofilms, which are thin, flat structures containing both cells and a film-like substance that the cells secrete. Availability of food and water influences the way colonies spread; however, since movement and growth are accompanied by mechanical forces, physical constraints are also important. These include the ability of the bacteria to change the water balance and their local mechanical environment, and the forces they create as they grow and move.
Previous research has used a variety of experimental and theoretical approaches to explain the dynamics of bacterial swarms and biofilms as separate phenomena. However, while they do differ biologically, they also share many physical characteristics.
Srinivasan et al. wanted to exploit these similarities, and use them to predict the growth and shape of biofilms and bacterial swarms under different conditions. To do this, a unified mathematical model for the growth of both swarms and biofilms was created. The model accounted for various factors, such as the transport of nutrients into the colony, the movement of water between the colony and the surface on which it grew, and mechanical changes in the environment (e.g. swelling/softening). The theoretical results were then compared with results from experimental measurements of different bacterial aggregates grown on a soft, hydrated gel. For both swarms and biofilms, the model correctly predicted how fast the colony expanded overall, as well as the shape and location of actively growing regions.
Biofilms and other bacterial aggregates can cause diseases and increase inflammation in tissues, and also hinder industrial processes by damage to submerged surfaces, such as ships and waterpipes. The results described here may open up new approaches to restrict the spreading of bacterial aggregates by focusing on their physical constraints.