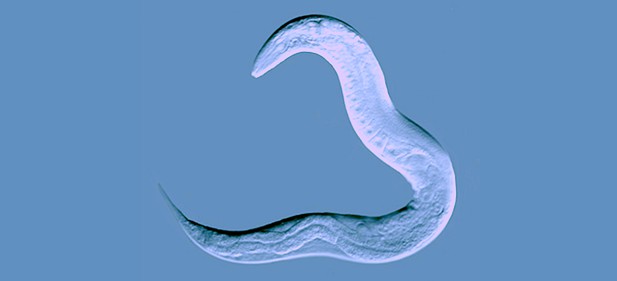
The nematode worm C. elegans is commonly studied in laboratory research. Image credit: Bob Goldstein (CC BY-SA 3.0)
Parasitic worms infect more than a billion people worldwide, using a range of tricks to survive inside the human body. Some species can live for weeks inside the gut, a place with almost no oxygen. Yet exactly how they manage this is remains unclear.
Scientists know that parasitic worms have an unusual way of making chemical energy when oxygen levels drop. Like human cells, worm cells use a series of molecular complexes called the electron transport chain. As electrons pass along the chain, they drive the production of chemical energy. Normally, oxygen sits at the end of the chain to receive the electrons. But, when there is no oxygen, almost all animals stop using the electron transport chain. A few animals can continue to use it by using other molecules to receive the final electrons instead of oxygen. To do that, they need a special electron carrier and, in worms, this electron carrier is rhodoquinone.
Human cells do not use rhodoquinone, making it a prime target for drug design. If a drug could block rhodoquinone production, it might be able to stop worms surviving in the human intestines without harming the patient’s own cells. Yet, even though the scientific community has known about rhodoquinone for more than 50 years, it remains unclear how worms make this molecule.
To find out, Del Borrello et al. examined the laboratory worm Caenorhabditis elegans. This worm is not a parasite, but it does make rhodoquinone. Del Borrello et al. developed a new way to study rhodoquinone production by blocking the normal route of the electron transport chain with cyanide. This causes the worms to switch to using rhodoquinone and is cheaper than raising the worms in low oxygen, making it easier to conduct high-throughput screening. A combination of chemistry and information from other species made it possible to identify candidate genes responsible for the production of rhodoquinone. Worms with faults in these genes revealed the key building blocks of rhodoquinone, and the early steps in its production. Removing any one of the genes made it harder for the worms to survive without oxygen.
Although there are already effective drugs that kill parasitic worms, resistance is growing. A better understanding of rhodoquinone could lead to a new class of drugs to help control this major problem in global health. A drug that blocks any one of the production steps of rhodoquinone might be a future candidate for a new anti-parasitic worm therapy.