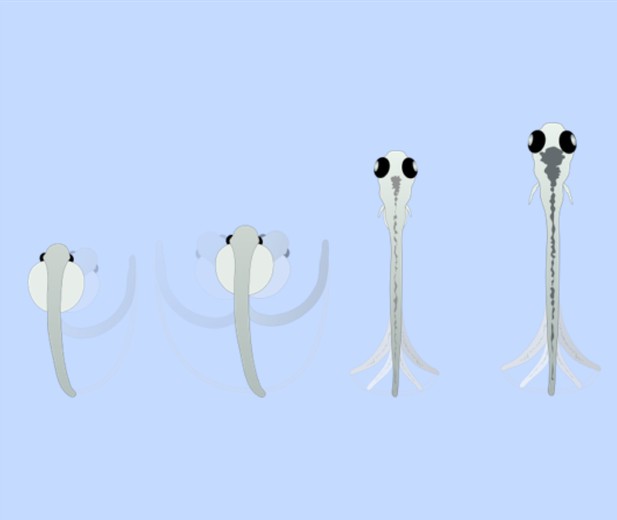
Schematic of a developing zebrafish and the movements it acquires as it grows. Image credit: adapted from Roussel et al. (CC BY 4.0)
The spinal cord is a column of nerve tissue that connects the brain to the rest of the body in vertebrate animals. Nerve cells in the spinal cord, called neurons, help to control and coordinate the body’s movements. As the spinal cord develops, new neurons are born and new connections are made between neurons and muscles, resulting in more coordinated and skillful movements as time goes on.
Zebrafish, for example, display body-bending maneuvers called coils within 24 hours of the egg being fertilized. Next, bursts of swimming movements emerge, which are driven by sporadic tail beats. These tail maneuvers become more consistent as the fish develops, and eventually result in smooth movements called beat-and-glide swimming. The groups of spinal cord neurons that appear at each stage of zebrafish development have been characterized, but it remains unclear how newly formed circuits (groups of neurons recently connected to each other) work together to produce swimming maneuvers.
To answer this question, Roussel et al. simulated changes in the spinal cord that help zebrafish acquire new swimming movements as they grow. The computer models encoded neural circuits based on cell populations identified in experimental studies, and replicated swimming behaviors that emerge during the first few days of zebrafish development. Simulations tested how specific neural circuits generate the characteristic swimming movements that represent key developmental milestones in zebrafish.
The results showed that adding new neurons and more cell-to-cell connections led to increasingly sophisticated swimming maneuvers. As the zebrafish spinal cord matured, the fish were better able to control the pace and duration of their swimming movements. Roussel et al. also identified specific patterns of neural activity linked to particular maneuvers. For example, tail beats switch direction when neurons on one side of the spinal cord excite neurons on the opposite side. This activity, which becomes more rhythmic, also needs to be exquisitely timed to produce and coordinate the right motion.
Roussel et al.’s modelling of developmental milestones in growing zebrafish provides insights into how neural networks control movement. The computer models are among the first to accurately reproduce swimming behaviors in developing zebrafish. More experimental data could be added to the models to capture the full range of early zebrafish movements, and to further investigate how maturing spinal cord circuits control swimming. Since zebrafish and mammals have many spinal neurons in common, further research may aid our understanding of movement disorders in humans.