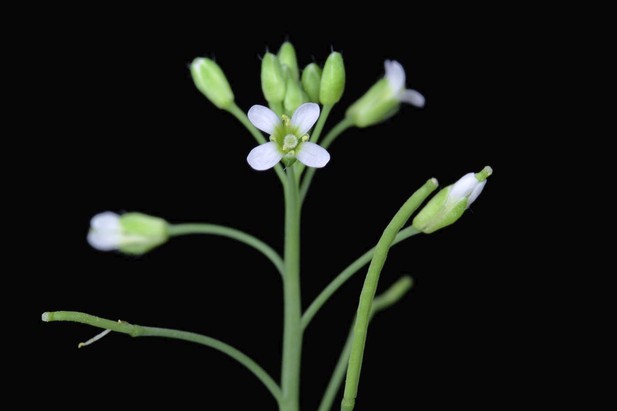
Flower of Arabidopsis thaliana. Image credit: Stewart Malecki (CC BY 4.0)
All the information necessary to build the proteins that perform the biological processes required for life is encoded in the DNA of an organism. Making these proteins requires the DNA sequence of a gene to be transcribed into a ‘messenger RNA’ (mRNA), which is then processed into a final, mature form. This blueprint is then translated to assemble the corresponding protein.
When an mRNA is processed, segments of the sequence that do not code for protein are removed and the remaining coding sequences are joined together in the right order. An intricate molecular machine known as the spliceosome controls this mechanism by recognising the ‘splice sites’ where coding and non-coding sequences meet. Depending on external conditions, the spliceosome can ‘pick-and-mix’ the coding sequences to create different processed mRNAs (and therefore proteins) from a single gene. This alternative splicing mechanism is often used to regulate when certain biological processes take place based on environmental cues; for example, the splicing of genes which control the timing of plant flowering is sensitive to ambient temperatures.
To investigate this mechanism, Parker et al. focused on Arabidopsis thaliana, a plant that blooms later when temperatures are low. This precise timing partly relies on a gene whose mRNA is efficiently spliced in the cold, resulting in an active form of its protein that blocks blooming. Parker et al. grew and screened many A. thaliana plants to find individuals that could flower early in the cold, in which splicing of this gene was disrupted.
A mutant fitting these criteria was identified and subjected to further investigation, which revealed that it could not produce FIONA1. In non-mutant plants, this enzyme chemically modifies one of the components of the spliceosome, a small nuclear RNA known as U6. Parker et al found that there are two types of splice site – one more likely to interact with U6 and another that preferentially interacts with another small nuclear RNA, U5. When FIONA1 is inactive (such as in the mutant identified by Parker et al.), splice sites that tend to strongly interact with U5 are selected. However, when the enzyme is active, splice sites that tend to bind with the chemically modified U6 are used instead.
Further work by Parker et al. showed that these two types of splice sites (‘preferring’ either U5 or U6) are found in equal proportions in the genomes of many species, including humans. This suggests that Parker et al. have uncovered an essential feature of how genomes are organised and splicing is controlled.