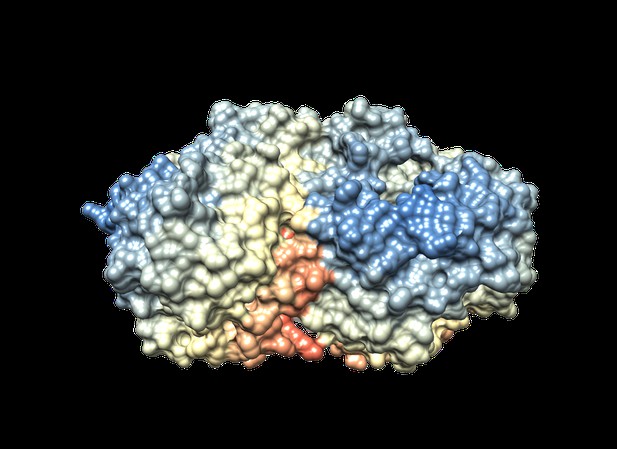
Neuraminidase is an enzyme present at the surface of the influenza virus. Changes in certain amino acids (blue), in particular those closer to the enzymatic site, are more likely to affect how the protein can escape immune recognition compared to modifications in other amino acids (red). Image credit: Catani et al. (CCBY 4.0)
Two proteins, the hemagglutinin and the neuraminidase, protrude from the surface of the influenza virus. Their detection by the immune system allows the host organism to mount defences against the viral threat. The virus evolves in response to this pressure, which manifests as changes in the appearance of its hemagglutinin and neuraminidase. This process, known as antigenic drift, leads to the proteins evading detection. It is also why flu vaccines require frequent updates, as they rely on ‘training’ the immune system to recognise the most important strains in circulation – primarily by exposing it to appropriate versions of hemagglutinin. While the antigenic drift of hemagglutinin has been extensively studied, much less is known about how the neuraminidase accumulates mutations, and how these affect the immune response.
To investigate this question, Catani et al. selected 43 genetically distant neuraminidases from human viral samples isolated between 2009 and 2017. Statistical analyses were applied to define their relatedness, revealing that a group of closely related neuraminidases predominated from 2009 to 2015, before they were being taken over by a second group. A third group, which was identified in viruses isolated in 2013, was remarkably close to the neuraminidase of strains that circulated in the late 1990s. The fourth and final group of neuraminidases was derived from influenza viruses that normally circulate in pigs but can also occasionally infect humans.
Next, Catani et al. examined the immune response that these 43 neuraminidases could elicit in mice, as well as in ferrets – the animal most traditionally used in influenza research. This allowed them to pinpoint which changes in the neuraminidase sequences were important to escape recognition by the host. Data obtained from the two model species were comparable, suggesting that these experiments could be conducted on mice going forward, which are easier to work with than ferrets. Finally, Catani et al. used machine learning to build a computational model that could predict how strongly the immune system would respond to a specific neuraminidase variant.
These findings could help guide the development of new vaccines that include neuraminidases tailored to best prime and train the immune system against a larger variety of strains. This may aid the development of ‘supra-seasonal’ vaccines that protect against a broad range of influenza viruses, reducing the need for yearly updates.