Cell Biology: Make or break for mitochondria
Mitochondria are usually described as being the main source of energy for cells, but they do much more than this. They are, for example, involved in controlling various aspects of the cell cycle and cell growth, and this requires them to undergo repeated cycles of division and fusion. Moreover, mitochondria contain their own DNA, and this needs to be replicated and distributed between the two daughter mitochondria that are produced in the division process.
Mitochondrial division is driven by an enzyme called Dnm1 that assembles into a helix around the mitochondria, and an enzyme called Drp1 performs the same role in mammals. However, the diameter of this helix is much smaller than the diameter of a typical mitochondrion, so the latter must be constricted by something before the helix can form.
It was recently reported that portions of the endoplasmic reticulum (ER) wrap around mitochondria at constriction sites marked by Dnm1/Drp1 helices in yeast/mammalian cells (Friedman et al., 2011). Moreover, depletion of Drp1 does not disrupt the constriction process, which suggests that contact between the ER and mitochondria is a conserved feature of mitochondrial division that precedes the involvement of Dnm1/Drp1.
Contact between the ER and mitochondria enables efficient communication between the two, which is essential for processes such as calcium signaling, lipid biosynthesis and protein import (Rowland and Voeltz, 2012). It is thought that this contact is mediated by one or more protein tethers, but to date only one such tether—a multiprotein complex called the ER-mitochondria encounter structure (ERMES; Kornmann et al., 2009)—has been identified in yeast.
Now, writing in eLife, Jodi Nunnari of the University of California at Davis and colleagues–including Andrew Murley as first author–report evidence that ERMES resides at the contact sites between the ER and mitochondria where constriction takes place, and that another enzyme, Gem1, is needed to break these contacts after the mitochondria have divided (Murley et al., 2013). Gem1, which is already known to regulate other aspects of the interaction between the ER and mitochondria (Kornmann et al., 2011), contains two domains that bind calcium ions and two domains that break down GTP molecules (which are closely related to the ATP molecules used by mitochondria to store chemical energy).
Nunnari and co-workers—who are based at UC Davis, UC San Francisco and the University of Colorado at Boulder—used time-lapse confocal microscopy to study fluorescently-tagged ERMES and Gem1 proteins in living yeast cells. The ERMES subunits were found to localize to the constriction sites in both wild-type yeast and in mutant yeast lacking Gem1 (Figure 1). The mitochondria also went on to divide successfully in both types of yeast, but both daughter mitochondria remained stuck to the ER in the mutant yeast cells (Figure 1). This suggests that Gem1 is required to disengage or mobilize ER–mitochondria contacts, which is important for the proper distribution of mitochondria in cells.
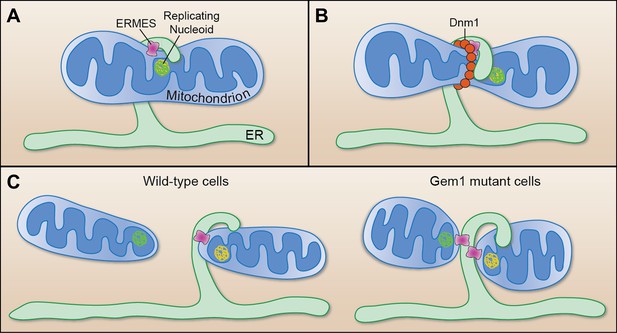
Mitochondrial division.
(A) Murley et al. demonstrate that the ERMES tethering complex (pink) localizes to the region where the endoplasmic reticulum (ER) makes contact with a mitochondrion in yeast, and where the nucleoid that contains the mitochondrial DNA is replicating. (B) The mitochondrion undergoes constriction at this contact site, which allows a helix of Dnm1 (red) to form around it. (C) This helix leads to further constriction and, ultimately, to the division of the mitochondrion and the formation of two daughter mitochondria in wild-type cells (left). Each daughter mitochondrion has its own nucleoid. Significantly, one daughter remains attached to the ER via ERMES, which remains intact through the division process. However, in cells lacking Gem1, both of the daughter mitochondria remain tethered to the same ER segment, and both have somewhat unusual shapes (right).
FIGURE CREDIT: CATHERINE L NEZICH
Murley et al. also added fluorescent tags to nucleoids—the structures that contain the mitochondrial DNA—and confirmed that they were located adjacent to ERMES complexes (Hobbs et al., 2001) at the majority of the constriction/division sites. Before division occurred these nucleoids were seen to exhibit behaviour that is indicative of DNA replication taking place, and after division they were mostly observed at the tips of both of the new daughter mitochondria. The contact sites between the ER and mitochondria thus appear to preferentially form near replicating nucleoids in order to assist with the distribution of DNA between the daughter mitochondria (Figure 1).
But how does Gem1 function? Murley et al. demonstrate that the GTPase activity of this protein, but not its calcium-binding activity, is required for the final release of ER–mitochondria contacts, which is consistent with the results of mitochondrial inheritance studies (Koshiba et al., 2011). It has been suggested that ERMES components link the nucleoids to the cellular cytoskeleton (Boldogh et al., 2003). This notion is compatible with the results of experiments which found that the enzymes Miro1 and 2—which are similar to Gem1 in many ways—mobilize mitochondria in mammalian cells by interacting with components of the cytoskeleton (Glater et al., 2006). Gem1 could thus be involved in the recruitment of protein motility factors that promote the spatial separation of the daughter mitochondria following the division process. Alternatively, Gem1 might act directly on ERMES to regulate the physical link between the ER and mitochondria. Consistent with this idea, Miro1 localizes to ER-mitochondria contact sites in mammalian cells, and Gem1 associates with ERMES subunits and regulates the number and size of ERMES foci in yeast (Kornmann et al., 2011).
The discovery of a novel role for ERMES and Gem1 proteins by Murley et al. raises new questions about mitochondrial division. First, does the ER facilitate constriction and, if so, how? One possibility is that an exchange of lipids at the ER–mitochondria interface alters the content of the mitochondrial membrane in this specific region, and that this leads to the constriction required for Dnm1 helix formation. Alternatively, ERMES (or its as yet unidentified mammalian equivalent) may facilitate the generation of a constricting force via actin polymerization (Korobova et al., 2013). And how is Dnm1 recruited to the constriction? Possibilities include via the ERMES complex itself or via some component of the ER that we don’t yet know about. Or maybe the curvature of the membrane activates the proteins that help to recruit the Dnm1/Drp1 enzymes that form the helix around the constriction.
While the present results leave these and many other questions to be resolved, future research delving into these unknowns should lead to a better understanding of the many neurodegenerative diseases that result from errors in the process of mitochondrial division.
References
-
The conserved GTPase Gem1 regulates endoplasmic reticulum-mitochondria connectionProc Natl Acad Sci USA 108:14151–14156.https://doi.org/10.1073/pnas.1111314108
-
Endoplasmic reticulum-mitochondria contacts: function of the junctionNat Rev Mol Cell Biol 13:607–625.https://doi.org/10.1038/nrm3440
Article and author information
Author details
Publication history
Copyright
© 2013, Nezich and Youle
This article is distributed under the terms of the Creative Commons Attribution License, which permits unrestricted use and redistribution provided that the original author and source are credited.
Metrics
-
- 1,808
- views
-
- 159
- downloads
-
- 5
- citations
Views, downloads and citations are aggregated across all versions of this paper published by eLife.
Download links
Downloads (link to download the article as PDF)
Open citations (links to open the citations from this article in various online reference manager services)
Cite this article (links to download the citations from this article in formats compatible with various reference manager tools)
Further reading
-
- Cell Biology
- Neuroscience
The claustrum complex is viewed as fundamental for higher-order cognition; however, the circuit organization and function of its neuroanatomical subregions are not well understood. We demonstrated that some of the key roles of the CLA complex can be attributed to the connectivity and function of a small group of neurons in its ventral subregion, the endopiriform (EN). We identified a subpopulation of EN neurons by their projection to the ventral CA1 (ENvCA1-proj. neurons), embedded in recurrent circuits with other EN neurons and the piriform cortex. Although the ENvCA1-proj. neuron activity was biased toward novelty across stimulus categories, their chemogenetic inhibition selectively disrupted the memory-guided but not innate responses of mice to novelty. Based on our functional connectivity analysis, we suggest that ENvCA1-proj. neurons serve as an essential node for recognition memory through recurrent circuits mediating sustained attention to novelty, and through feed-forward inhibition of distal vCA1 neurons shifting memory-guided behavior from familiarity to novelty.
-
- Cell Biology
- Computational and Systems Biology
Induced pluripotent stem cell (iPSC) technology is revolutionizing cell biology. However, the variability between individual iPSC lines and the lack of efficient technology to comprehensively characterize iPSC-derived cell types hinder its adoption in routine preclinical screening settings. To facilitate the validation of iPSC-derived cell culture composition, we have implemented an imaging assay based on cell painting and convolutional neural networks to recognize cell types in dense and mixed cultures with high fidelity. We have benchmarked our approach using pure and mixed cultures of neuroblastoma and astrocytoma cell lines and attained a classification accuracy above 96%. Through iterative data erosion, we found that inputs containing the nuclear region of interest and its close environment, allow achieving equally high classification accuracy as inputs containing the whole cell for semi-confluent cultures and preserved prediction accuracy even in very dense cultures. We then applied this regionally restricted cell profiling approach to evaluate the differentiation status of iPSC-derived neural cultures, by determining the ratio of postmitotic neurons and neural progenitors. We found that the cell-based prediction significantly outperformed an approach in which the population-level time in culture was used as a classification criterion (96% vs 86%, respectively). In mixed iPSC-derived neuronal cultures, microglia could be unequivocally discriminated from neurons, regardless of their reactivity state, and a tiered strategy allowed for further distinguishing activated from non-activated cell states, albeit with lower accuracy. Thus, morphological single-cell profiling provides a means to quantify cell composition in complex mixed neural cultures and holds promise for use in the quality control of iPSC-derived cell culture models.