Mouse rods signal through gap junctions with cones
Figures
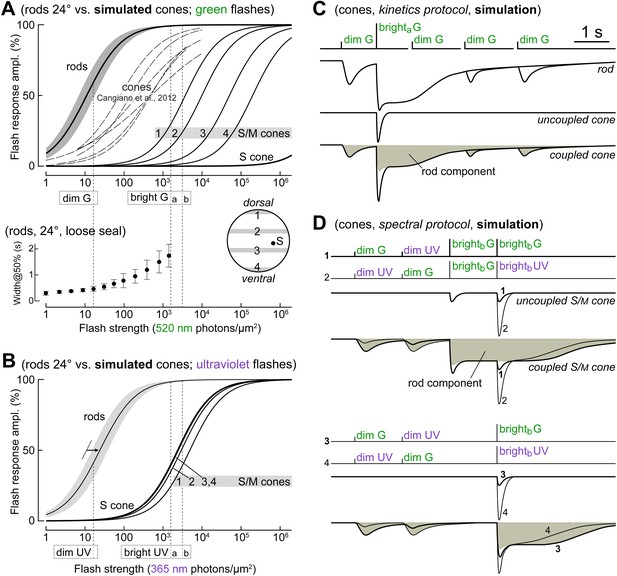
The different flash sensitivities, kinetics, and spectral preferences of rods and cones are exploited to dissect their electrical coupling.
(A upper graph) Comparison of rod and cone responses to green flashes. Continuous lines on the right are simulated flash response profiles of mixed S/M cones at four retinal latitudes (1–4, from dorsalmost to ventralmost; inset in lower graph) and pure S cones, based on recently published models (Daniele et al., 2011; Wang et al., 2011; see ‘Materials and methods’). Thick line and shaded area on the left show the mean ± 1 SD of rod flash response profiles from patch and loose seal recordings in this study (n = 16; 24°C; average of Michaelis–Menten fits in individual rods). Dashed lines in the middle reproduce flash response profiles from spectrally unidentified mouse cones recently recorded with patch (reproduced from Figure 3; Cangiano et al., 2012, 24°C): the high sensitivity of cone photovoltages is suggestive of rod input. (A lower graph) Width at 50% amplitude (mean and SD) of rod flash responses, obtained here with loose seal recordings to avoid kinetics rundown (n = 4, 24°C). (B) Comparison of rod and cone responses to ultraviolet flashes. Rod response profiles in the ultraviolet were obtained by rightward shift of those in the green (panel A) by a factor of 2.2 (arrow; our estimate from two rods in which full flash response profiles were delivered at both wavelengths). Graphs in panels A and B were used to select dim and bright flashes for the protocols used in our experiments (C and D), aimed at dissecting rod input in cones: dim flashes (16.6 photons·µm−2) elicited large responses in rods while being too weak to stimulate cones, while bright flashes (a: 1570, b: 3140 ph·µm−2) were sufficient to saturate rods for >1 s and evoke moderate responses in cones. (C) Kinetics protocol made of sequences of three 520 nm (green, G) flashes, each consisting of dim/brighta/dim flashes, with the third flash occurring at increasing delays. Expected responses in a rod, an uncoupled cone, and a coupled cone. (D) Spectral protocol made of sequences of 4 dim and brightb flashes at 520 nm (G) and 365 nm (UV). Expected responses in an uncoupled and coupled S/M-cone (UV-opsin-dominated cone). When coupled, the cone should prefer dim G to dim UV flashes, while the opposite should occur with bright flashes. The cone’s intrinsic spectral phenotype is unmasked with a rod-saturating pre-flash.
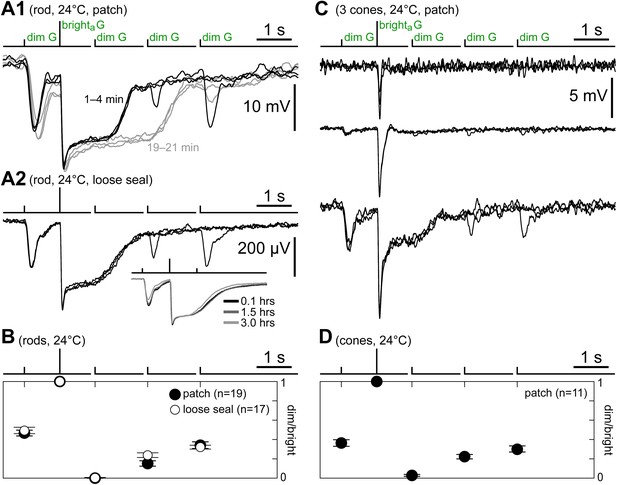
Cones express a rod-like sensitivity to dim flashes and slow recovery after bright flashes.
(A1) Response of a patched rod to the kinetics protocol (Figure 1C) in the first minutes after establishing the seal (black traces). At later times, a previously described rundown of kinetics was observed (gray traces; see Cangiano et al., 2012). (A2) Loose seal recording showing a scaled version of the rod photovoltage in response to the kinetics protocol. The advantage of the loose seal approach is that no kinetics rundown takes place, even in very long recordings (inset). (B) Summary of rod responses to the kinetics protocol in patch (black circles; data from the first 2 min after sealing) and loose seal recordings (white circles). Dim flash responses were normalized to those of the brighta flash (bars are SEM). Rods display a large response to the first dim flash and a progressive recovery after the brighta flash. (C) Responses of three cones to the kinetics protocol, representing the observed spectrum of behaviors. (D) Summary of data from a subset of cones that displayed large dim flash responses. The time course of recovery of the dim flash response after the bright flash is comparable to that of rods. In panels A1, A2, and C, baselines were aligned to each other (max shift 2 mV), and in all records (except C/top), each trace was the average of several sweeps.
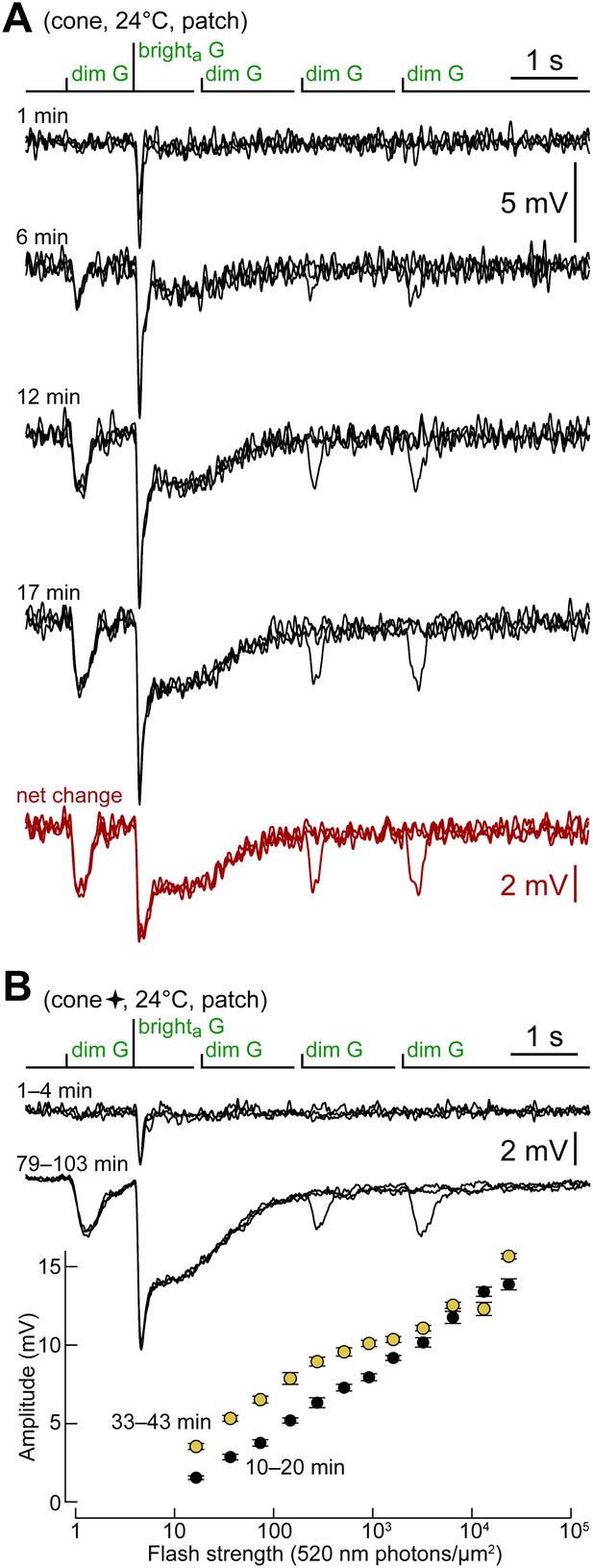
Cones shift toward a rod-like phenotype during recording.
(A) Response of a cone to the kinetics protocol delivered at 1, 6, 12 and 17 min after obtaining the seal (records are not averages; Vdark ≈ −44 mV). In this experiment, darkness was maintained between recordings. The net change between the first and the average of the last two records (12 and 17 min) matches the response of rods (Figure 2A). (B) A different cone in which the kinetics protocol was delivered at close intervals for an extended time. Records above compare the average responses of this cone to the kinetics protocol at the beginning of the experiment, with those after >1 hr. The graph below shows the selective increase in sensitivity to dimmer flashes that occurred during the recording (bars are SEM). This cone also appears in Figure 4 (four-pointed star).
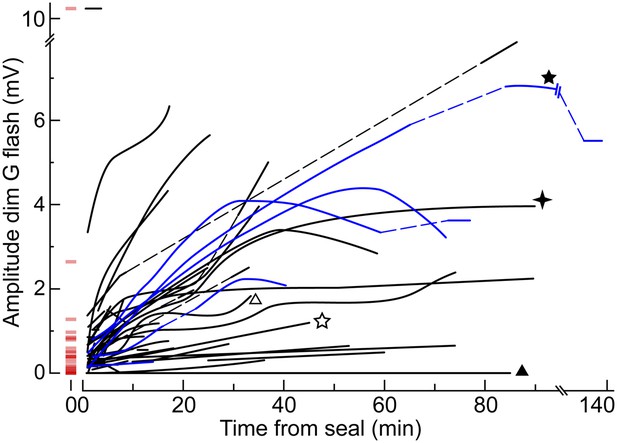
The dim flash sensitivity of cones increases during recording.
Time course of the peak amplitude of the response to the dim G flash in 50 cones. Each curve corresponds to a different cell and is a qualitative fit to the raw data points. In a subset of cones, the unperturbed amplitude of the dim G flash response could be extrapolated with a reasonable degree of confidence (red horizontal segments at time zero). Some cones were recorded with an EGTA zero Ca2+ perforated patch solution in the pipette (blue lines; see ‘Results’). Dashed lines represent gaps in the data resulting from the delivery of other protocols. Some cones also appear in other figures (stars and triangles).
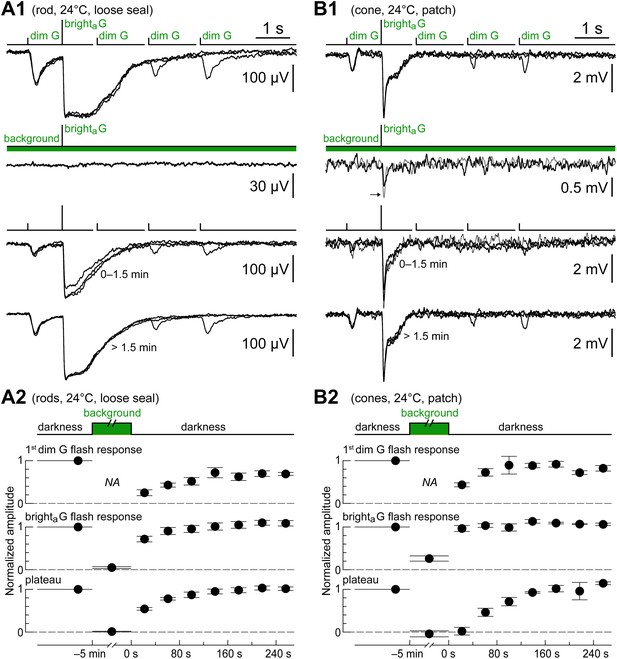
Rods and cones recover from a rod-saturating background at the same rate.
(A1) Loose seal recording of a rod showing the complete suppression of its response to the kinetics protocol by a saturating light background (520 nm, 6100 photons·µm−2·s−1 for 5 min; dim G flashes were not delivered during the background), and its recovery upon returning to darkness. (A2) Graphs summarizing the effect of the light background on this and two additional rods. The response amplitudes of the first dim G flash, the brighta flash, and the plateau (0.4–0.6 s post bright flash) were normalized to their control values (bars show the SEM, while their horizontal extent shows the time range of the underlying flashes). (B1) Recording of a cone of high light sensitivity and slow kinetics showing the effect of the same rod-saturating background. In contrast to rods, a fast response component persisted in the cone during the background (arrow; gray trace shows the effect of increasing flash strength by a factor of 3.7). (B2) Graphs summarizing the effect of the light background on this and two additional cones. The time course of recovery in cones matched that of rods. All records in A1 and B1 are averages obtained in the specified time ranges.
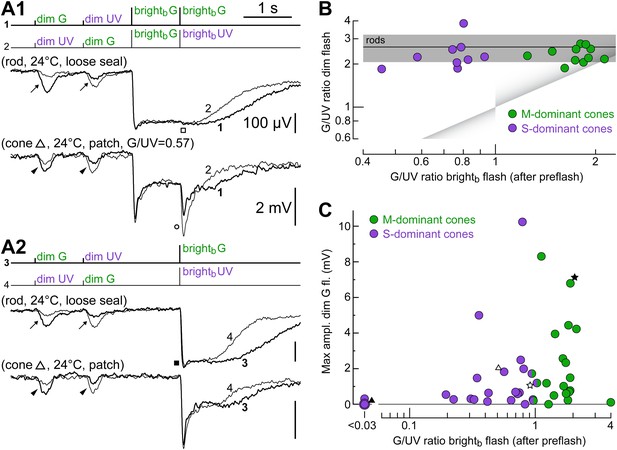
Irrespective of their dominant cone opsin, for dim flashes, cones prefer green light.
(A1 and A2) Responses of a rod and a cone to the spectral protocol (Figure 1D). As expected, the rod was more sensitive to dim G than dim UV flashes (arrows), it saturated with brightb G and brightb UV flashes (filled box) and did not respond after a rod-saturating pre-flash (empty box). Similar to the rod, the cone was more sensitive to dim G flashes (arrowheads). However, its responses after a rod-saturating pre-flash unmasked an intrinsic preference for UV light (empty circle). (B) Cones of widely varying intrinsic spectral preference (corresponding to widely varying M- vs S-opsin expression levels) display a rod-like preference for green light when tested with dim flashes. Spectral preference was quantified as the ratio of G and UV flash response amplitudes. Line and shaded areas show the mean dim flash preference ± 1 SD in rods (four loose seal and four patch recordings). Triangular shades show the expected location in the graph of uncoupled cones. (C) Both M- and S-dominant cones couple to rods, as shown by a plot of the maximum dim G flash response amplitude observed in each cone vs its intrinsic spectral preference.
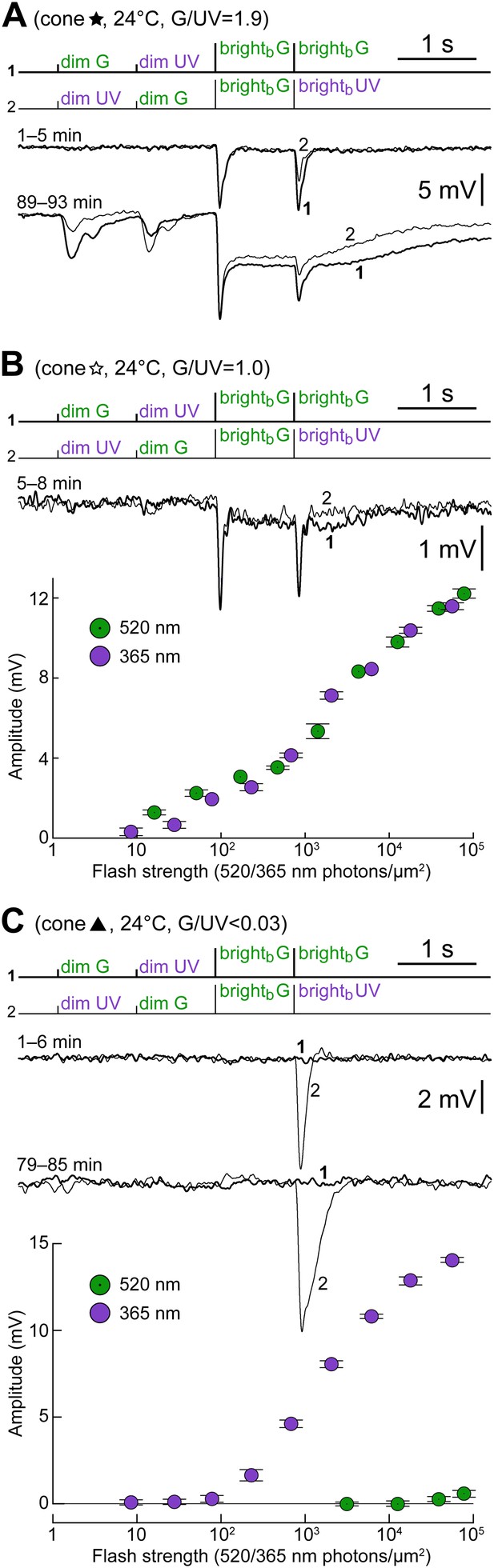
Examples of cones with different S- vs M-opsin expression levels.
(A) Initially uncoupled M-dominant cone, which then develops strong coupling (also shown in Figure 4 and Figure 6C, labeled by a black five-pointed star). (B) Weakly coupled S/M cone with approximately equal sensitivity to G and UV light (white five-pointed star in previous figures). (C) Uncoupled, presumably pure S-cone (black triangle in previous figures). All records are averages obtained in the specified time ranges. Bars are SEM.
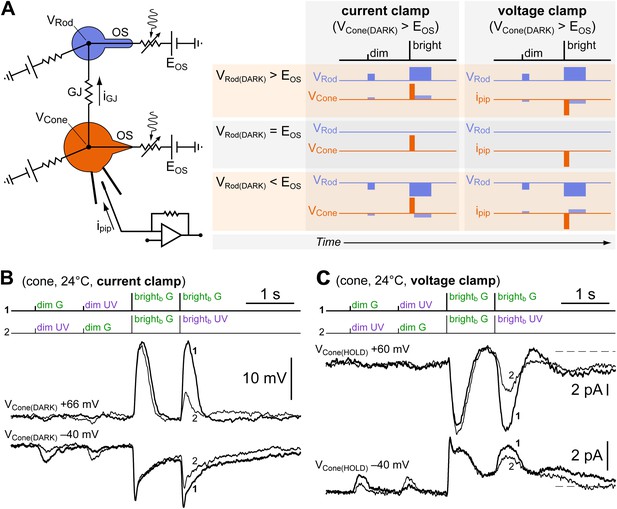
Dim and bright flash responses in cones originate from separate electrotonic compartments.
(A left) Equivalent circuit of a recorded cone (orange) coupled to neighboring rods (blue) via GJs (OS, outer segment; EOS, reversal potential of the rod/cone light sensitive conductance; GJ, gap junction; iGJ, junctional current; ipip, pipette current; VRod and VCone, rod/cone membrane potential). (A right) When the cone is depolarized, either in current clamp (by constant current injection) such that VCone(DARK) > EOS, or in voltage clamp such that VCone(HOLD) > EOS, a junctional current iGJ will flow into the rods and depolarize them beyond, at, or below EOS. Each of these three possible outcomes is expected to lead to the indicated different combinations of response polarities when delivering dim and bright flashes. (B) A reduced version of the spectral protocol (sequences 1–2 in Figure 1D) was delivered with a cone recorded in current clamp in control conditions (VCone(DARK) = −40 mV) or during depolarization by constant current injection beyond the reversal potential of its light-sensitive conductance (VCone(DARK) = +66 mV). While brightb flash responses reversed polarity, dim flash responses became smaller but did not reverse. This is not compatible with an origin of the dim and bright flash responses in the same electrotonic compartment, and matches one of the predicted outcomes for coupled cones (panel A, VRod(DARK) < EOS). The moderate shift toward G in the spectral preference displayed by this cone (second brightb flash) could be explained by cone–cone coupling and/or by initial recovery from saturation of its coupled rods (see ‘Discussion’). (C) The same experiment as in panel B but performed in voltage clamp in a different cone (VCone(HOLD) = −40 mV and +60 mV). Dim flash responses could not be detected above noise when the cone was depolarized, despite the presence of large inverted brightb flash responses. This matches a different predicted outcome for coupled cones (panel A, VRod(DARK) = EOS). The slight shift toward G in the spectral preference displayed by this cone (second brightb flash) is likely explained by a slow ‘bump’ in the plateau displayed after brightb flashes, present only at −40 mV (not shown, but observed in sequence three of the spectral protocol). The outcome predicted for the case of VRod(DARK) > EOS in panel A was never observed. Records are averages of 3–4 sweeps.
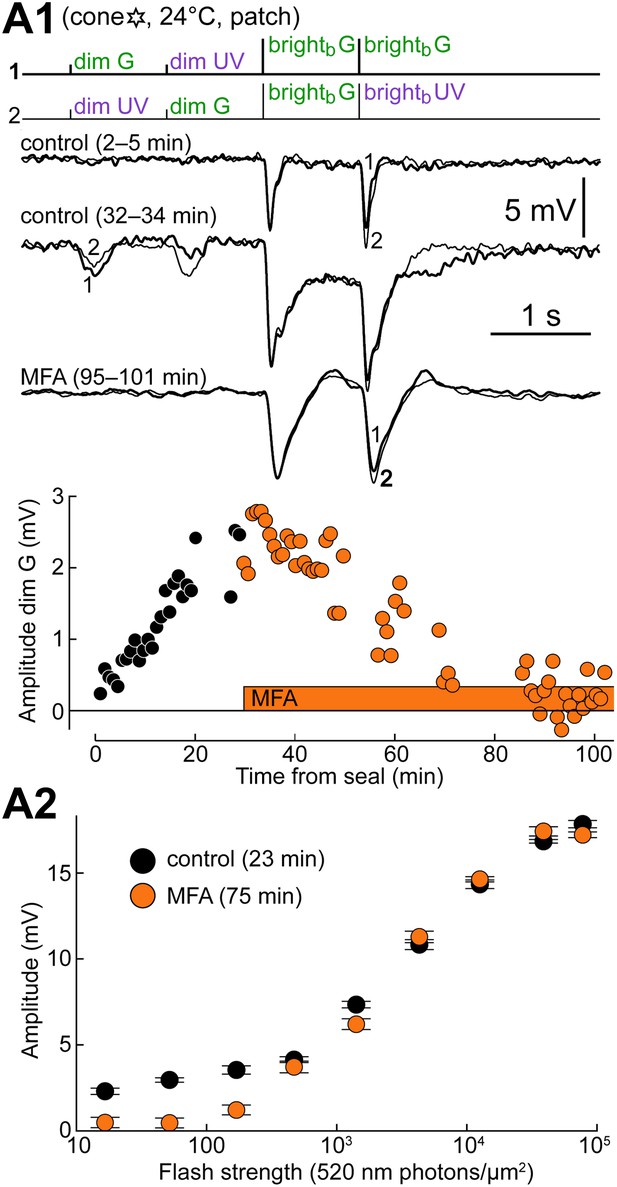
Blocking gap junctions reverts cones to their intrinsic phenotype.
(A1) Response of a cone to the spectral protocol during its spontaneous shift toward a rod phenotype (top and middle records) and subsequent superfusion with the GJ blocker meclofenamic acid (MFA, 100 µM; bottom records). MFA abolished both dim flash responses and bright flash plateaus. Records are averages. (A2) Response amplitude vs flash strength for the same cone as in A1 before and during perfusion with MFA. Note the selective reduction in dim flash sensitivity. Bars are SEM.
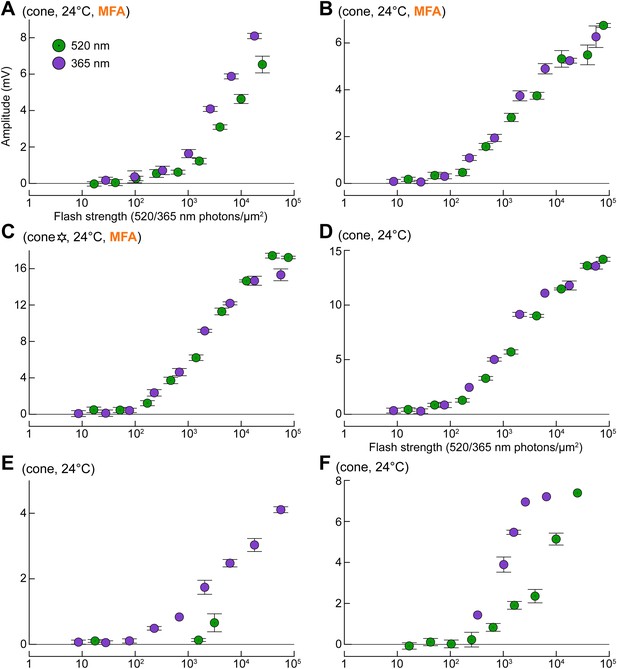
Uncoupled cones display light sensitivities comparable to those predicted from literature (compare with Figure 1A,B, simulated curves).
(A–C) Response profiles of three cones to G and UV flashes, recorded during superfusion with 100 µM MFA. The cone in panel C is also shown in Figure 9 (six-pointed star). (D–F) Response profiles to G and UV flashes in three naturally uncoupled cones. Bars are SEM.
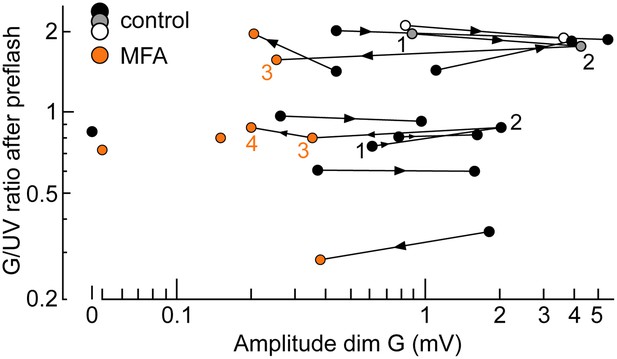
The spontaneous increase in coupling and MFA had a limited impact on our estimates of intrinsic cone spectral preferences.
Graph of the ratio of G over UV flash response amplitudes (brightb flashes delivered after a rod saturating pre-flash; spectral protocol) vs dim G flash response amplitude. Data points from the same cone are connected by lines, with the arrow showing time progression. For two cones, complete data were available spanning both coupling increase and MFA superfusion (1–4). Also shown are two cones sealed upon with MFA already in the bath (isolated orange circles) and a completely uncoupled cone in normal solution (isolated black circle).
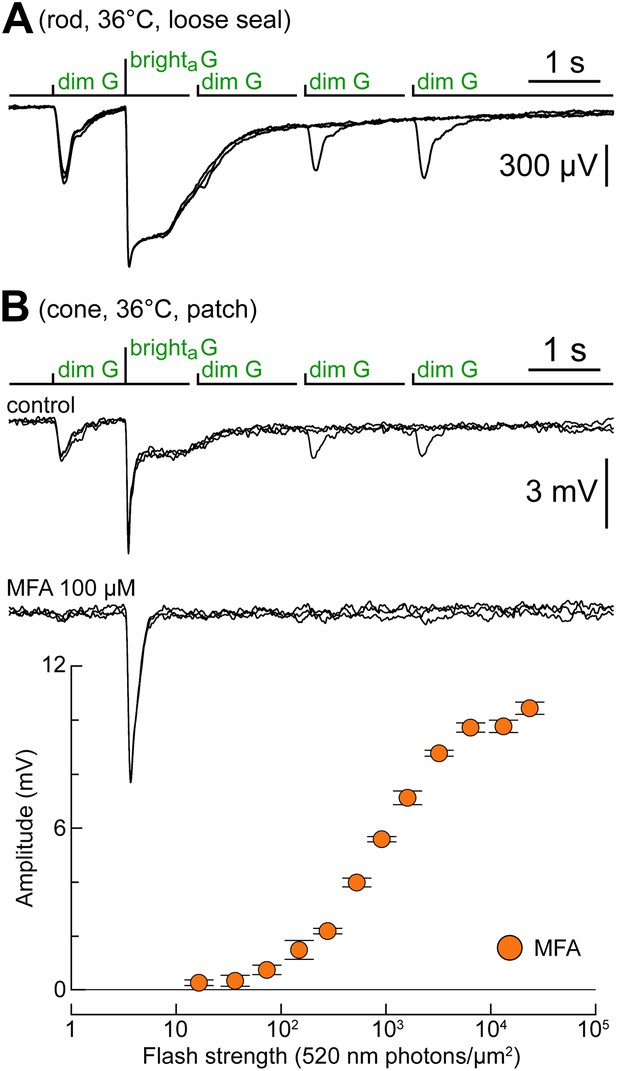
Rod–cone coupling is also expressed near body temperature.
(A) Loose seal recordings at 36°C in a rod, showing its response to the kinetics protocol. As expected, rod recovery from the brighta flashes was faster compared to near room temperature. (B) Cone recorded at 36°C displaying a rod-like phenotype in response to the kinetics protocol (upper records). MFA abolished dim flash responses and slow plateaus after the bright flash (lower records). The graph shows the G flash response profile of the cone during superfusion of MFA.
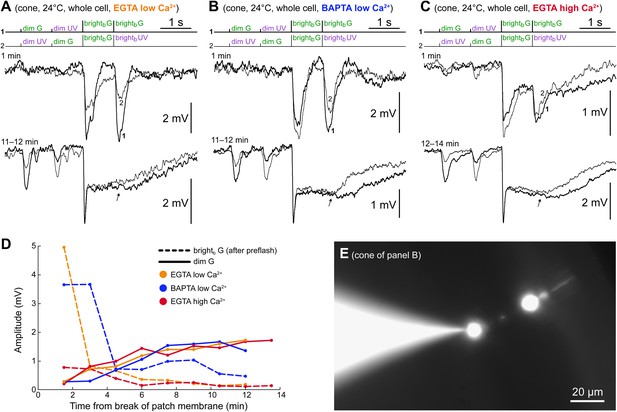
The spontaneous increase in coupling does not require Ca2+ changes in the cone.
(A–C) Three cones recorded in whole cell patch clamp using either low Ca2+ intracellular solutions (50 nM free [Ca2+]) buffered with EGTA (panel A) or BAPTA (panel B), or a high Ca2+ intracellular solution (approx. 5 µM free [Ca2+]) buffered with EGTA. All three cones expressed the same time-dependent increase in coupling to rods observed in perforated patch recordings: increased dim flash response and bright flash plateau amplitudes. Note that, in contrast to the perforated patch, the component of the light response originating in the cones themselves ran down rapidly (second brightb flashes, arrows). Records are averages of 1–3 sweeps. (D) Time course of rod (continuous lines) and cone (dashed lines) response components in the experiments shown in panels A–C. (E) Pedicles (cone synaptic terminals) were targeted in all of these whole cell recordings to ensure a rapid and effective calcium clamp at the GJs, which are located on the adjacent telodendria. The image shows a Lucifer Yellow stain of the cone in panel B with the pipette sealed on the pedicle (final image obtained by blending two photographs acquired on slightly different focal planes; pedicle and cell body appear larger than their actual size due to an intentional overexposure during acquisition, implemented to highlight the dim outer segment).