Criticality and degeneracy in injury-induced changes in primary afferent excitability and the implications for neuropathic pain
Figures
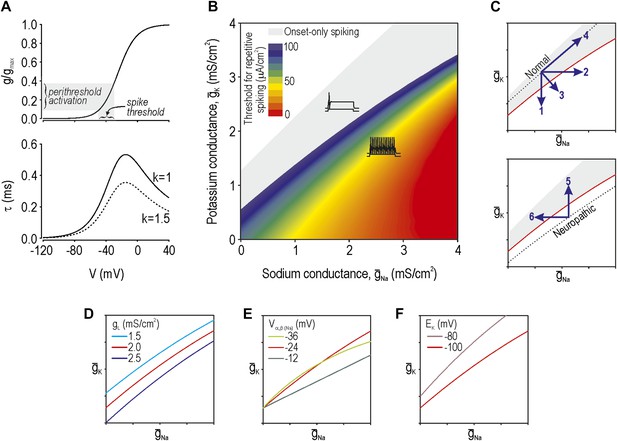
Modeling and theory.
(A) Voltage-dependency and kinetics of subthreshold conductance. In bottom panel, τ = (α+β)−1 where α and β are defined in Equations 3 and 4. Equivalent activation parameters were used to implement either a sodium or potassium current based on reversal potential. (B) Two-parameter bifurcation analysis in which ḡNa and ḡK were co-varied to determine the ḡNa:ḡK ratio for a given threshold for repetitive spiking. Gray shading shows parameter regime associated with onset-only spiking. Changes in excitability are explained by a switch in spike initiation dynamics (Figure 1—figure supplement 1). (C) Predictions (indicated by numbered arrows) of how manipulating ḡNa and/or ḡK may force the system across a tipping point, thus reproducing or reversing neuropathic excitability. Position of the tipping point depends on many other parameters including leak conductance (D), voltage-dependency of ḡNa and ḡK (E), and potassium reversal potential (F).
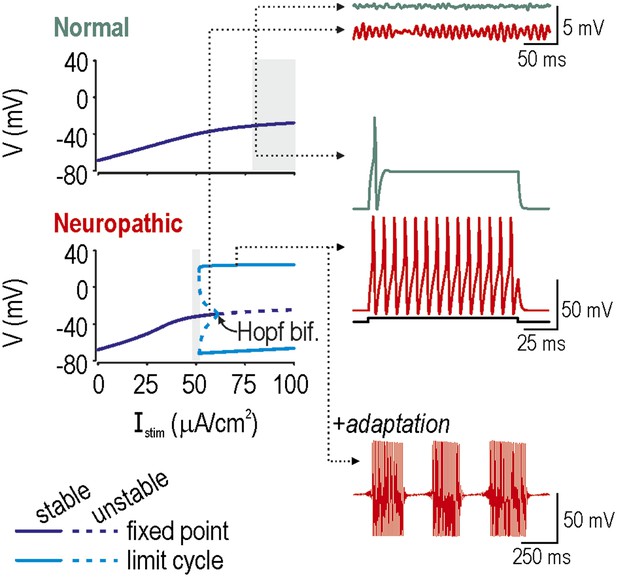
Spike initiation dynamics differ between normal and neuropathic conditions.
In bifurcation analysis, a parameter such as stimulus intensity Istim is systematically varied to determine at what value the system qualitatively changes behavior, which is to say it undergoes a bifurcation. We repeated this analysis in a model with the ḡNa:ḡK ratio set to give normal excitability (ḡNa = 2.0 µS/cm2; ḡK = 2.5 µS/cm2) or neuropathic excitability (ḡNa = 2.5 µS/cm2; ḡK = 2.0 µS/cm2). Bifurcation diagrams (left) illustrate how the system behaves at different Istim: a stable fixed point corresponds to quiescence whereas a stable limit cycle corresponds to repetitive spiking. A subcritical Hopf bifurcation is evident in the bottom (Neuropathic) bifurcation diagram but not in the top (Normal) bifurcation diagram, suggesting that repetitive spiking is only possible in the former condition. In both conditions, the Istim range in which a single spike is produced at stimulus onset is indicated with gray shading. Onset-only spiking is achieved through a quasi-separatrix crossing, independent of any bifurcation. Dotted arrows indicate Istim values for sample traces on the right. Membrane potential oscillations (MPOs) occur when the fixed point is in proximity to a Hopf bifurcation; after being perturbed by noise, the system relaxes towards its nearly-unstable fixed point following a loose spiral trajectory, which corresponds to MPOs on the time series. In the absence of a Hopf bifurcation, the fixed point is more stable and MPOs are negligible. Repetitive spiking occurs when Istim exceeds the intensity required for the Hopf bifurcation. Repetitive spiking can also occur in the Istim range between the Hopf bifurcation and where the stable and unstable limit cycles meet (at a saddle-node bifurcation of limit cycles); that region also contains a stable fixed point, meaning that the system is bistable. The neuron will remain in one or the other stable state unless a slow process like adaptation sweeps the system across the bistable region, in which case the neuron will remain quiescent when sweeping rightward toward the Hopf bifurcation, while spiking repetitively when sweeping leftward toward the saddle-node bifurcation of limit cycles, thereby producing bursts as a consequence of hysteresis. Bistability and adaptation are both required for bursting.
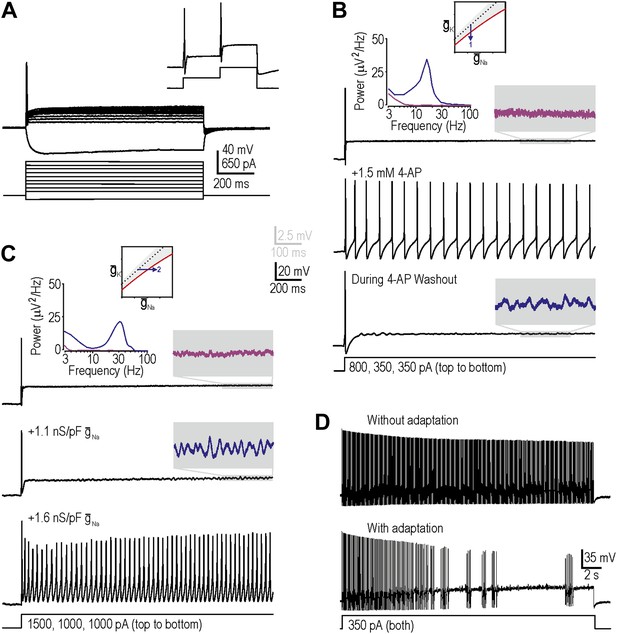
Reproduction of neuropathic excitability in naive cutaneous neurons.
(A) Medium- to large-diameter (putative myelinated) cutaneous afferents from naive rats respond to depolarization with a single spike at stimulus onset. Neurons can respond to increments in stimulus intensity (inset), which demonstrates that sodium channels are not completely inactivated after the initial spike. (B) Prediction 1 was tested by blocking potassium current by application of 1.5–5 mM 4-AP. By repeating stimulation during wash-in and/or wash-out of the drug, we observed repetitive spiking and MPOs (in the order expected based on the change in drug concentration) in response to equivalent stimulation. Gray-shaded windows show enlarged views of voltage traces, with colors corresponding to the power spectra shown at the top. (C) Prediction 2 was tested by adding virtual sodium conductance via dynamic clamp. As predicted, MPOs and repetitive spiking developed as virtual sodium conductance was increased, but bursting was absent. Note that a conductance density of 1 nS/pF corresponds to 1 mS/cm2 assuming a specific membrane capacitance of 1 μF/cm2, which means that the conductance densities added by dynamic clamp are the same order of magnitude as those predicted by modeling in Figure 1B. (D) Bursting was achieved in repetitively spiking neurons by introducing an AHP-type adaptation current via dynamic clamp.
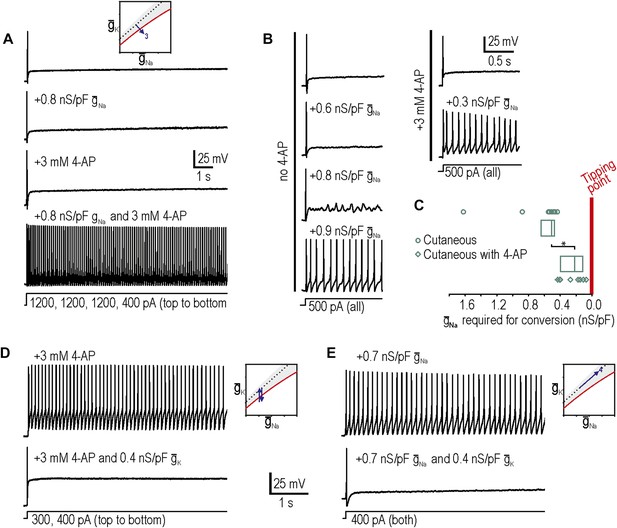
Additivity and subtractivity of conductance changes.
(A) Example in which neither virtual sodium conductance nor potassium channel blockade reproduced neuropathic excitability, whereas the combination of manipulations did, thus confirming prediction 3, that manipulations can be additive. (B) Example in which the minimum virtual sodium conductance required to reproduce neuropathic excitability was reduced when combined with potassium channel blockade. (C) Even when 4-AP application did not, on its own, produce repetitive spiking, it nonetheless moved the neuron significantly closer to criticality as evidenced by comparing the minimal virtual sodium conductance needed to produce repetitive spiking without vs with 4-AP (*p<0.001; Mann–Whitney U test). Points represent data from individual neurons and boxes represent median and 25–75 percentile range. Repetitive spiking caused by 4-AP application (D) or by virtual sodium conductance (E) was reversed by insertion of virtual potassium conductance. Panel E confirms prediction 4, that manipulations can be subtractive.
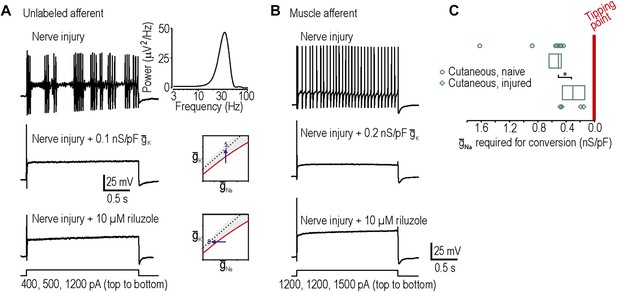
Reversal of neuropathic excitability in neurons from nerve-inured rats.
(A) Typical response of an injured unlabeled primary afferent (top). Repetitive spiking and MPOs were abolished by insertion of a potassium conductance (middle) or by blockade of sodium channels using 10 μM riluzole (bottom), thus confirming predictions 5 and 6, respectively. (B) Typical response of an injured muscle afferent whose neuropathic excitability was similarly reversed by increased potassium conductance and reduced sodium conductance. (C) Although only 1 of 9 injured cutaneous afferents exhibited repetitive spiking and MPOs, those afferents were nonetheless closer to criticality insofar as they required significantly less virtual sodium conductance than uninjured cutaneous afferents to reach criticality (*p<0.05; Mann–Whitney U test). Points represent data from individual neurons and boxes represent median and 25–75 percentile range.
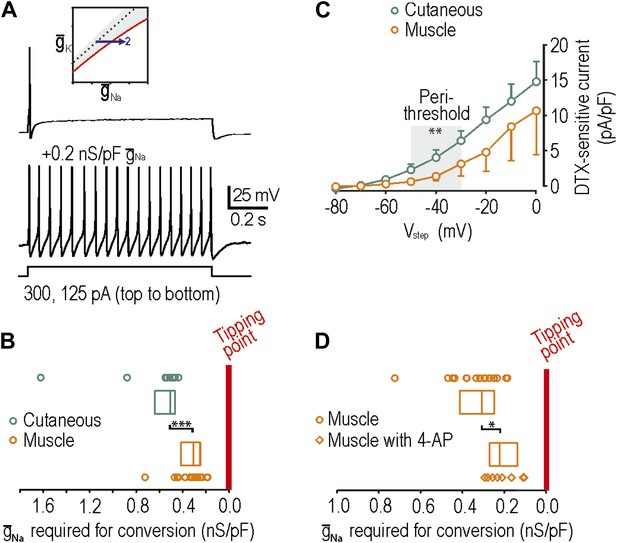
Reproduction of neuropathic excitability in naive muscle afferents.
(A) As predicted, repetitive spiking developed as virtual sodium conductance was increased. (B) Uninjured muscle afferents operate significantly closer to criticality than uninjured cutaneous afferents based on the minimum virtual sodium conductance needed to produce repetitive spiking (***p<0.001; Mann–Whitney U test). In B and D, points represent data from individual neurons and boxes represent median and 25–75 percentile range. These data suggest that muscle afferents are more prone to develop neuropathic excitability after nerve injury because they start off closer to their tipping point, and not necessarily because injury induces a larger change in membrane conductances. (C) Based on the observation that 4-AP and dendrotoxin (DTX) produced repetitive spiking in only 1 of 12 muscle afferents, we measured the DTX-sensitive current. The persistent outward current activated at perithreshold voltages (−50 to −30 mV) and blocked by 10 nM DTX was significantly smaller in muscle afferents than in cutaneous afferents (**p<0.01; two-way ANOVA and post-hoc Student-Newman-Keuls test). (D) Although 4-AP produced repetitive spiking in only 1 of 12 muscle afferents, it nonetheless moved neurons closer to criticality as evidenced by the minimal virtual sodium conductance needed to cause hyperexcitability after 4AP application (*p<0.05; Mann–Whitney U test).