Molecular assembly of the period-cryptochrome circadian transcriptional repressor complex
Figures
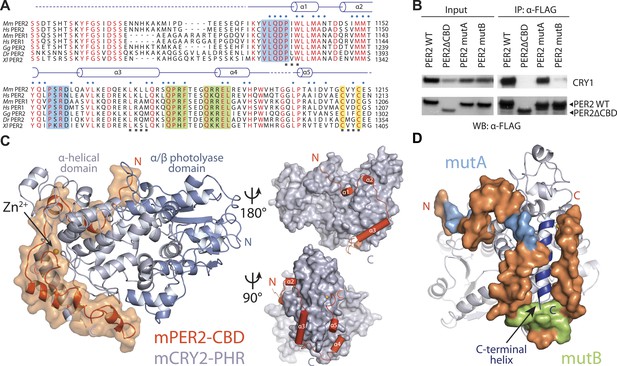
Overall structure of the murine CRY2–PER2 complex at 2.8 Å.
(A) PER2 CBD sequence alignment. 49% of PER2 CBD residues interact with CRY2 (blue dots). The zinc-coordinating residues are conserved throughout vertebrates (highlighted in yellow). Blue and green boxes correspond to the mutA and mutB constructs, respectively, and indicate regions of PER2-CBD that were mutated to alanines. Dashed lines indicate crystallographically disordered regions. Black squares indicate residues mutated under structure guidance. (B) Co-immunoprecipitation of mutant PER2-CBD-FLAG constructs, only mutB was able to abolish CRY1-MYC binding. Western blot of an immunoprecipitaion of COS7 cells transfected with PER2-NLS-FLAG and CRY1-MYC. Proteins were precipitated with α-FLAG and then analyzed by Western blots using α-MYC and α-FLAG. (C) CRY2 PHR (gray) adopts an overall fold identical to its apo and complexed forms (e.g., FAD, FBXL3, and KL001). PER2 CRY-binding domain (CBD) (orange) shows a highly extended binding mode around CRY2. PER2 flanks the CRY2 C-terminal helix and coordinates a zinc ion with CRY2 within a CCCH-type intermolecular zinc finger motif. (D) Crystallographic data identify the location of alanine scanning mutants. Importantly, the mutB construct is centered around the CRY2 C-terminal helix.
-
Figure 1—source data 1
Alanine scanning mutants of PER2 CBD.
Each individual mutation was unable to disrupt PER-CBD-CRY1 binding. Only the combined mutation of two nearby stretches of residues on the C-terminal half of PER2-CBD was able to abolish binding.
- https://doi.org/10.7554/eLife.03674.004
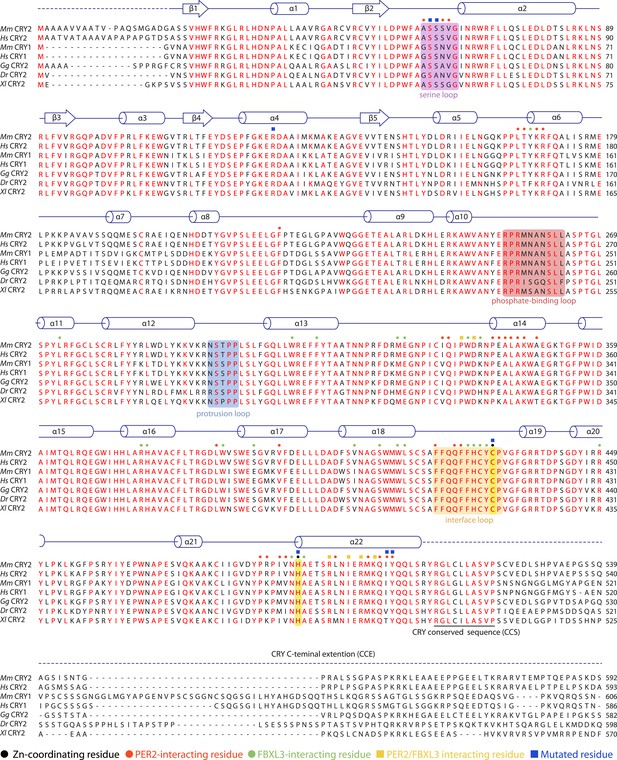
Sequence alignment and structural elements of vertebrate CRY.
Alignment and secondary structure assignments of CRY2 orthologs from Mus musculus (Mm), Homo sapiens (Hs), Gallus gallus (Gg), Danio rerio (Dr), and Xenopus laevis (Xl). Strictly conserved residues are colored in red. Blue and green dots indicate mPER2-CBD-, and hFBXL3-interacting residues, respectively. Yellow squares indicate residues that interact with both PER2 and FBXL3. Black dots indicate residues that are involved in zinc coordination. Colored boxes represent the boundaries of structurally dynamic loops. Dashed line represents the regions outside the PHR.
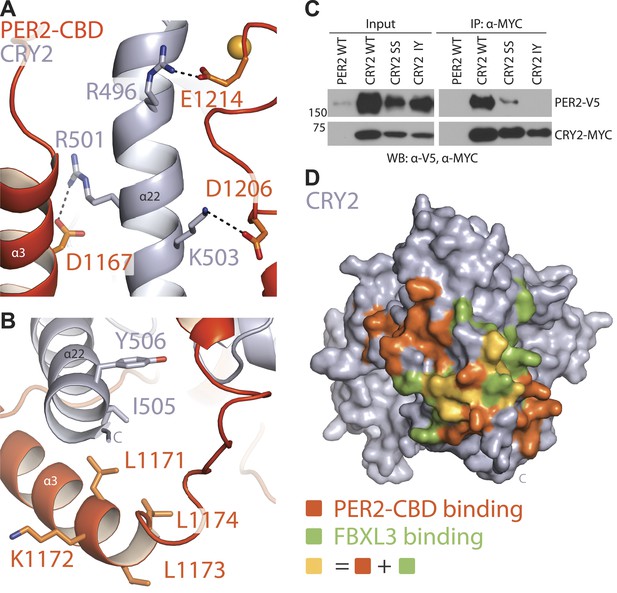
CRY2 C-terminal helix is the central locus of both PER2 and FBXL3 interactions.
(A) PER2 (orange) forms three salt-bridges along CRY2 C-terminus helix (gray) R501 and K503 have been previously reported as critical binding residues. (B) A close-up view of the PER2-CRY2 interface at the end of CRY2 C-terminal helix. While the upper portion of the CRY2 C-terminal helix maintains ionic interactions with PER2, the lower is predominantly mediated by hydrophobic interactions. CRY2 and PER2 residues chose for subsequent mutational analysis are shown in sticks. (C) Concurrent mutations of hydrophobic residues on the CRY C-terminal helix (I505D and Y506D) prevent PER-CRY complex formation. Co-immunoprecipitations were performed with transfected full-length PER2-V5 and MYC-CRY2 in HEK293 cells with α-MYC beads and analyzed by Western blotting using α-V5 and α-MYC. See Figure 2—figure supplement 1A for corresponding PER2 mutants. (D) Surface mapping of FBXL3- and PER2-binding sites on CRY2. Residues that share contacts with PER2 and FBXL3 are colored in yellow and are clustered along the C-terminal helix. Other residues involved in binding PER2 and FBXL3 are colored in orange and green, respectively.
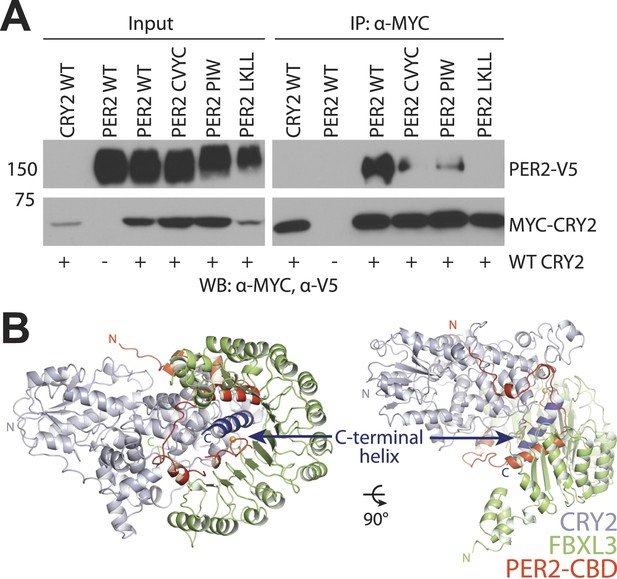
Mutational and structural analysis of the PER2-CRY2 interface.
(A) Diminished PER2-CRY2 interaction was replicated in a co-immunoprecipitation assay, in which the CXXC motif of the full-length FLAG-tagged PER2 protein was mutated to four alanines. Co-immunoprecipitations were performed with transfected full-length PER2-V5 and MYC-CRY2 in HEK293 cells with α-MYC beads. (B) Superimposition analysis demonstrates the direct competition of PER2-CBD (orange) and FBXL3 (green) binding to CRY (gray), which is centered around the CRY2 C-terminal helix (blue).
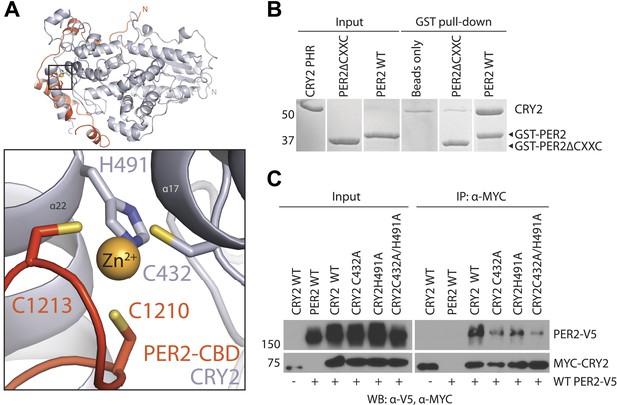
The intermolecular zinc finger is important for PER2–CRY2 complex formation.
(A) Four conserved, contributing residues from PER2 (C1210 and C1213) and CRY2 (C432 and H491) form a CCCH-type zinc finger. (B) GST-pull-down assay with recombinant GST-tagged PER2ΔCXXC CBD and untagged CRY2-PHR protein show compromised CRY binding in the zinc finger mutant compared to WT PER2-CBD. (C) Similarly diminished interaction was replicated in a co-immunoprecipitation assay. Alanine mutations were introduced to CRY2 zinc-coordinating residues, C432 and H491, individually or in combination. Co-immunoprecipitations were performed with transfected full-length PER2 and CRY2 in HEK293 cells with α-MYC beads.
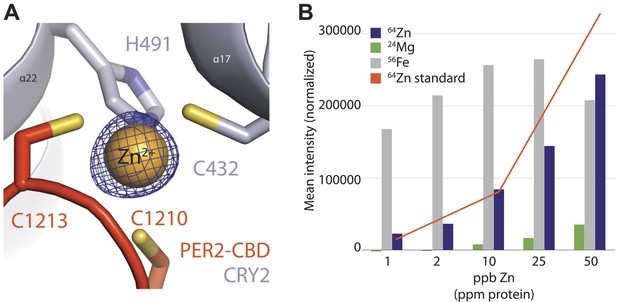
Analysis of the intermolecular zinc finger.
(A) Zinc-coordinating residues of PER2-CBD (orange) and CRY2 (gray) with zinc anomalous signal (λ = 1.284 Å) contoured at 7σ (blue mesh). (B) Inductively coupled plasma mass spectrometric analysis of metal isotopes. Purified PER2-CBD-CRY2 complex was dehydrated, dissolved in concentrated HNO3 overnight, diluted to 1% vol/vol HNO3, and titrated. Zn (purple) isotopes (64Zn, 66Zn, 67Zn, 68Zn, 70Zn) were the only ones that showed a greater than sixfold increase in mean signal intensity above the blank, dose-dependent increase, and approximated the predicted intensity of the standard (orange line). 24Mg (green) and 56Fe (gray) are shown as controls.
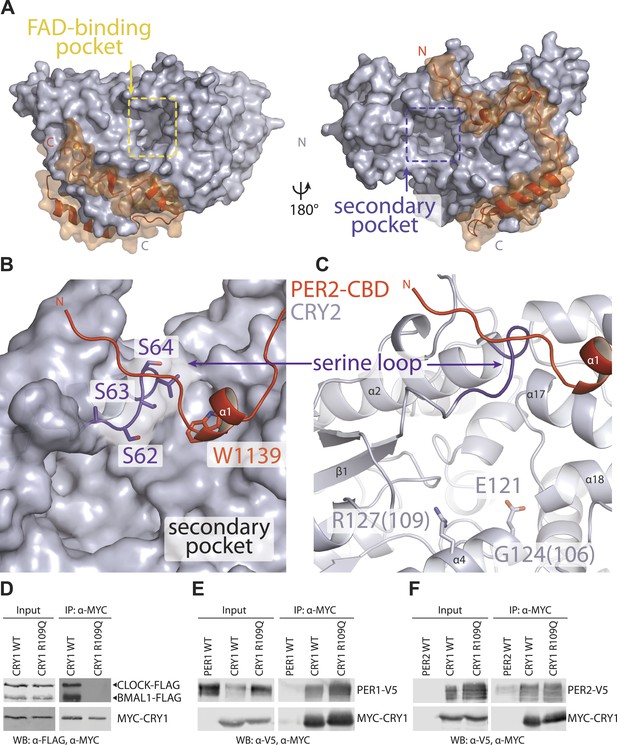
The secondary pocket is involved in CRY-CLOCK-BMAL1 complex assembly and repression.
(A) Relative positions of the two large pockets on CRY2. (B) Surface representation of CRY2 with side chains of the serine loop shown in sticks. PER2 α1 helix inserts into a hydrophobic cleft. Compared to other CRY2 complexed forms, the serine loop flips up and engages PER2. (C) The serine loop lies opposite to the CRY α4 helix, which together frame the secondary pocket, the α4 helix contains three residues (CRY1 G106R and R109Q, CRY2 E121K), whose mutations result in a weak repression phenotype. (D–F) Co-immunoprecipitation assays show that the CRY1 R109Q mutant is unable to bind CLOCK-BMAL1, but retains PER1 and PER2 binding.
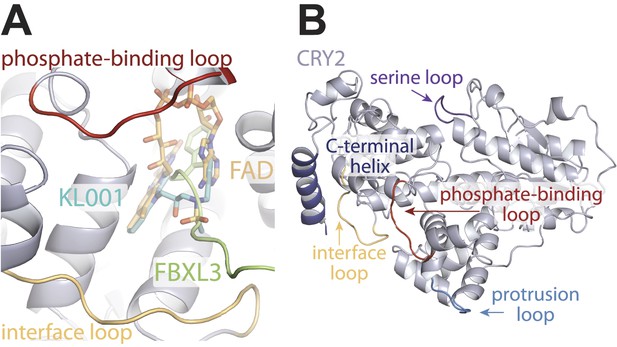
Locations of structurally plastic loops on CRY2.
(A) The phosphate-binding loop and interface loop frame opposite sides of the FAD-binding pocket. Superimposition analysis shows the FAD-binding pocket as a regulatory hotspot, which can bind metabolic cofactor, FAD (yellow), clock-modulating small molecule, KL001 (cyan), and FBXL3 C-terminal tail (green). (B) Locations of CRY2 loops; interface loop (yellow), phosphate-binding loop (red), serine loop (purple), and protrusion loop (light blue), which in light-sensitive CRYs occludes part of the FAD-binding pocket but is pushed outward and maintains an open FAD-binding pocket in vertebrate CRYs.
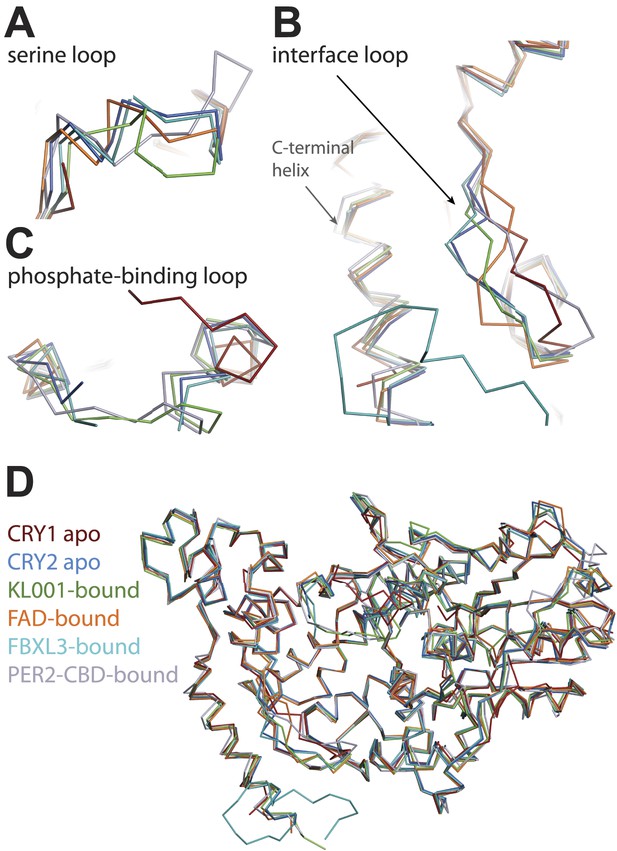
CRY-PHR superposition: including CRY1 apo (red), CRY2 apo (light blue), KL001-bound (green), FAD-bound (orange), FBXL3-bound (cyan), and PER2-CBD-bound (gray) CRY.
(A) Serine loop undergoes a large conformational change after PER2-CBD binding. (B and C) The interface loop and phosphate-binding loop are also sites of high structural plasticity. (D) Overall CRY-PHR showing the global structure adopts a common fold.
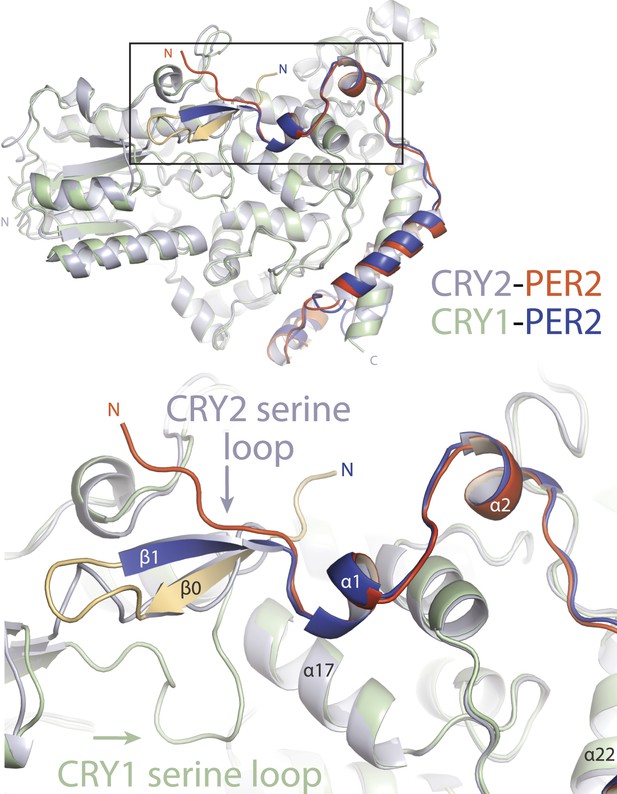
Major differences between CRY1-PER2-CBD and CRY2-PER2-CBD complex structures.
Superposition of the two structures reveals major structural dissimilarities between the two paralogs at the CRY secondary pocket and a residual fusion-protein sequence (yellow) in CRY1-bound PER2-CBD. The PER2-CBD (dark blue) N-terminus together with the artifactual sequence (AGLEVLFQGPDSM) forms a β-hairpin and induces an inward conformation of the CRY1 (light green) serine loop.
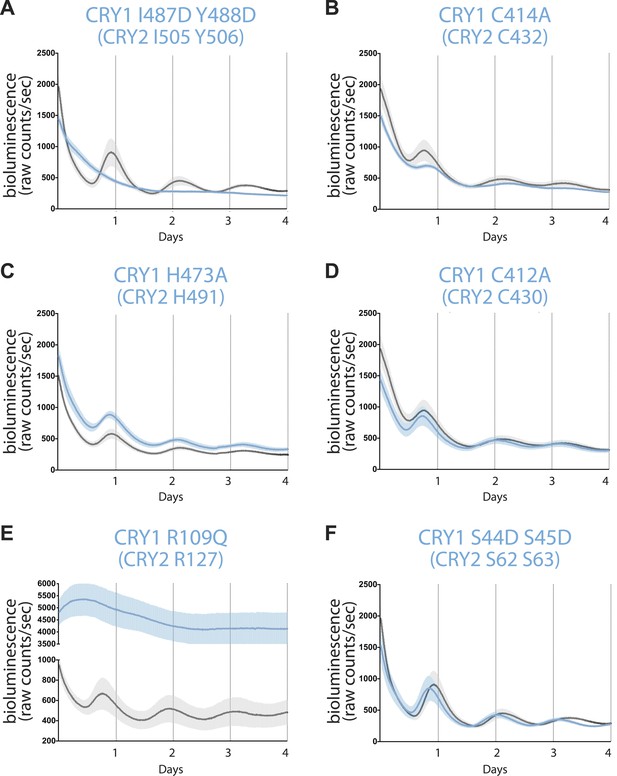
Real-time circadian rescue assays.
Cry1−/−/Cry2−/− MEFs were transfected 24 hr after plating with dLuc reporter plasmid and mCry1 expression or mutant vector. 72 hr after transfection, the cells were synchronized with dexamethasone. Bioluminescence (raw counts/s) monitoring was performed continuously for 70 s every 10 min using a photomultiplier tube at 37°C. Traces are shown as mean ± SEM and are representative of triplicate samples. Mutants are shown in blue and WT control in black. Only CRY1, not CRY2 is able to reconstitute robust circadian rhythmicity. (A) CRY1 I487D Y488D (CRY2 I505 Y506) ‘IY’ mutant abolishes rhythmicity but maintains repression compared to WT, suggesting that PER is not required for transcriptional repression. (B and C) Zinc-coordinating residues on CRY1 C414 and H473 (CRY2 C432 and H491) show blunted rhythm amplitude. (D) A nearby cysteine residue, C412 (CRY2 430), when mutated to alanine, does not show a significantly different phenotype from the WT control. (E) A critical residue on the secondary pocket, CRY1 R109 (CRY2 R127) shows a severely weakened repression phenotype when mutated to a glutamine. Traces are shown as mean ± SEM and are representative of duplicate samples. (F) Mutations of two serine residues in the serine loop, CRY1 S44D S45D (CRY2 S62 S63), show near WT rhythmicity and repression but with a 1-hr shorter period. For all mutants, corresponding CRY2 residues are in parenthesis.
Tables
Data collection and refinement statistics
CRY2-PER2 | |
---|---|
Data collection | |
Space group | P41 |
Cell dimensions | |
a, b, c (Å) | 97.67, 97.67, 163.21 |
α, β, γ (°) | 90, 90, 90 |
Resolution (Å) | 2.9 (2.8) |
Rmeas | 0.06 (0.8) |
I/σI | 18.8 (2.1) |
Completeness (%) | 99.6 (98.2) |
Redundancy | 4.2 (4.2) |
Refinement | |
Resolution (Å) | 42.7–2.8 |
No. reflections | 37541 (3671) |
Rwork/Rfree | 20.5/27.7 |
No. atoms | 9342 |
Protein | 9292 |
Ligand/ion | 2 |
Water | 48 |
B-factors | 97.3 |
Protein | 97.5 |
Ligand/ion | 114.1 |
Water | 66.3 |
R.m.s. deviations | |
Bond lengths (Å) | 0.009 |
Bond angles (°) | 1.3 |