Oscillatory phase separation in giant lipid vesicles induced by transmembrane osmotic differentials
Figures
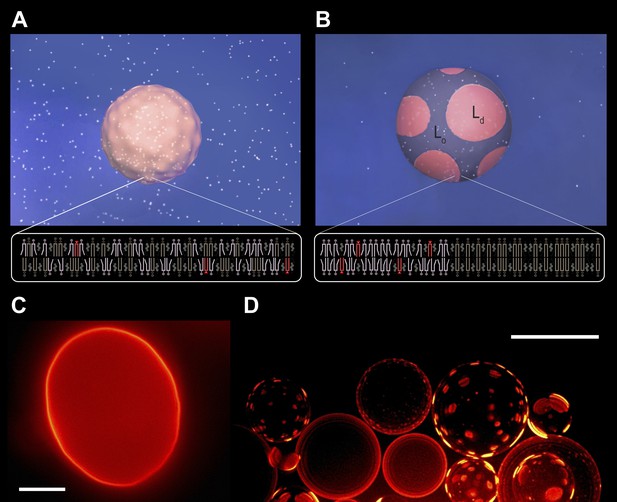
Subjecting giant unilamellar lipid vesicles to an osmotic differential.
(A–B), Schematic of a GUV immersed in an osmotically balanced isotonic bath (A). Dilution of the extra-vesicular dispersion medium by water subjects the GUV to a hypotonic bath producing an osmotic differential (B), which renders initially flaccid vesicles stiff and replaces the initially optically uniform membrane surface by one characterized by a domain pattern of co-existing Ld and Lo phases at microscopic length scales. Solute is rendered as white particles, membrane, pink, and domain pattern in pink and purple. (C–D) The process in (A–B) exemplified by wide-field fluorescence (C) and deconvolved (D) images of a solution of GUVs consisting of POPC:SM:Ch (1:1:1) labeled with 0.5 mol% Rho-DPPE at 25°C containing 200 mM sucrose concentration, osmotically balanced by 200 mM glucose in (C), and under an osmotic differential of ∼200 mM in (D) at 25°C. Scale bars: 15 μm.
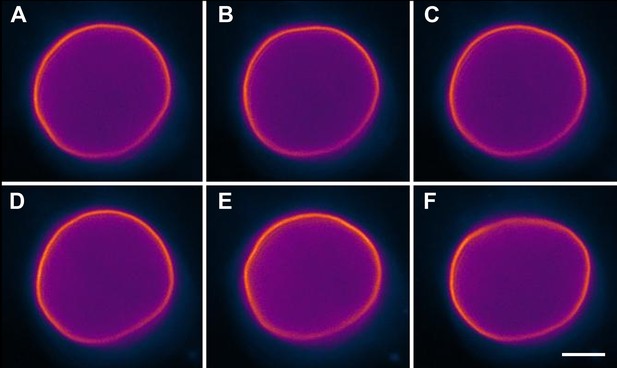
Undulating boundary of isotonic vesicles.
Selected time-lapse fluorescence images from Video 1 revealing out-of-plane membrane fluctuations typical for non-tense GUVs in the absence of osmotic gradient. Panels correspond to (A) 0 s, (B) 3 s, (C) 5 s, (D) 11 s, (E) 16 s, (F) 27 s. The GUV is composed of POPC:SM:Ch (1:1:1), labeled with 0.5% Rho-DPPE (pseudo-colored magenta), and imaged at 25°C. Scale bar: 5 µm.
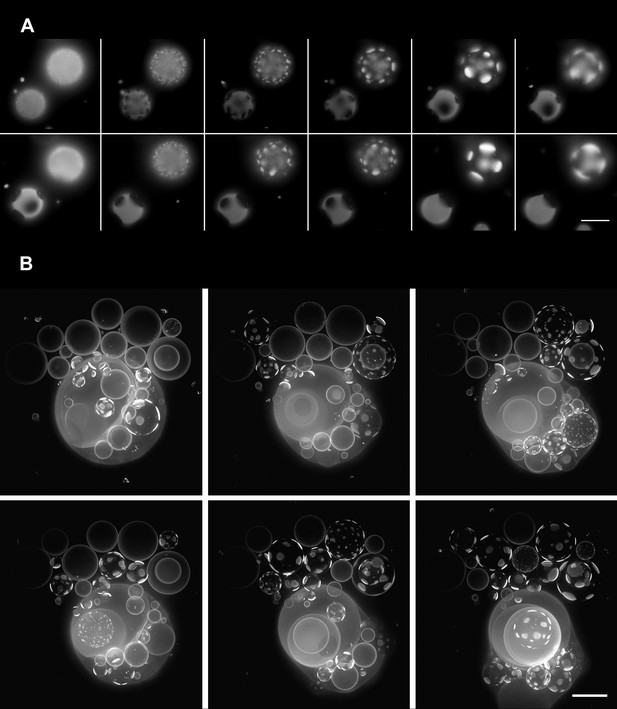
Oscillatory phase separation in hypertonic giant unilamellar vesicles subject to an osmotic imbalance.
(A), Selected frames from a video of time-lapse fluorescence images (Video 2) illustrating stages of domain dynamics during two consecutive cycles of oscillatory phase separation (t = 0 s, 9 s, 12 s, 15 s, 25 s, 27 s, 29 s, 188 s, 191 s, 193 s, 246 s, and 247 s). The GUVs imaged consist of POPC:SM:Ch (1:1:1) labeled with 0.5% Rho-DPPE, encapsulating 1 M sucrose, diluted in deionized water, at room temperature. Scale bar: 10 μm. (B) Selected images from time-lapse fluorescence images (Video 3) showing asynchronous cycling in a population of GUVs (t = 0 s, 98 s, 148 s, 294 s, and 448 s). The images are projections of Z-stacks of the lower hemispheres of GUVs consisting of POPC:SM:Ch (1:1:1) labeled with 0.5 mol% Rho-DPPE, encapsulating 200 mM sucrose, diluted in deionized water at 25°C (n = 5). Scale bar: 15 μm.
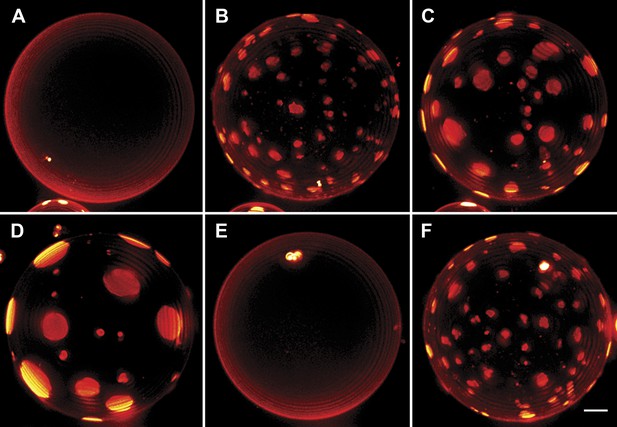
Interrupted imaging of oscillatory phase separation.
Z-stack projections of height-resolved fluorescence images of the lower hemisphere of a GUV consisting of POPC:SM:Ch (1:1:1) labeled with 0.5% Rho-DPPE (pseudo-colored red). The GUV encapsulates 200 mM sucrose, and the external dispersion medium is diluted in MilliQ water. Images are acquired at 25°C at arbitrary time points; (A) 0 s, (B) 99 s, (C) 148 s, (D) 299 s, (E) 550 s, and (F) 692 s. The first image was taken ∼2 hr after imposing the osmotic gradient. Scale bar: 5 µm.
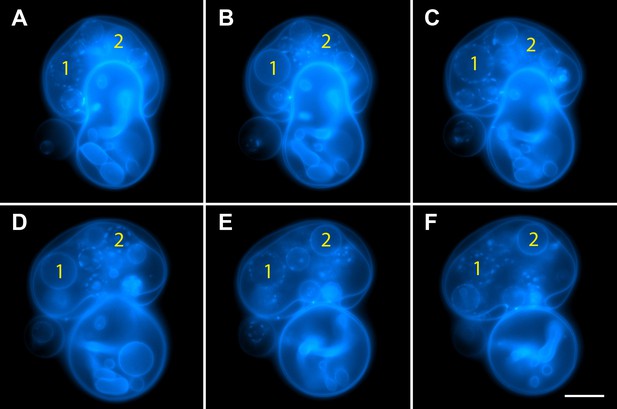
Oscillatory phase separation in complex GUVs prepared by hydration.
Arbitrarily selected micrographs from a time-lapse sequence of fluorescence images from Video 4 for GUVs prepared by ‘gentle hydration’. GUVs consist of POPC:SM:Ch (1:1:1) labeled with 0.5% Rho-DPPE (pseudo-colored blue), encapsulate 200 mM sucrose, and are subject to hypotonic conditions by dilution in MilliQ water at 25°C. Panels correspond to (A) 0 s, (B) 3 s, (C) 6 s, (D) 11 s, (E) 14 s, (F) 22 s, starting about 2 min after establishing the osmotic differential. Daughter vesicles identified as 1 and 2 illustrate clear oscillatory phase separation behavior over the experimental time scale captured by the images displayed. Scale bar: 10 µm.
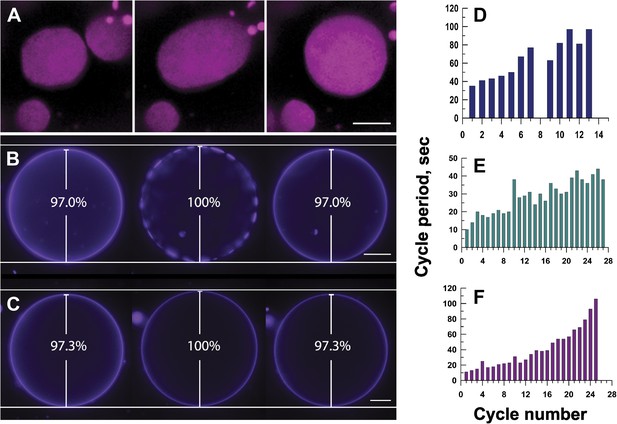
Mechanisms responsible for oscillatory phase separation in GUVs subject to osmotic differentials.
(A) Domain coarsening. Selected frames from Video 4 illustrating collision and coalescence of domains during a swell segment of the GUV oscillations (Ld phase, pseudo-colored magenta). Images are 1 s apart focused on a region of interest located at the bottom of a GUV. Scale bar: 5 μm. (B–C) Relationship between vesicle swelling and phase-separation. Fluorescence images revealing (B) that largest domains are observed under conditions of maximal swelling (t = 0 s, 8 s, and 106 s). Scale bar: 10 μm. (C) Control experiment using single component POPC GUVs, labeled with 0.5% Rho-DPPE, encapsulating 200 mM sucrose, diluted in deionized water at 25°C, confirm that the GUV swelling does not require domain formation and/or reorganization. Scale bar: 10 μm. (D–F) Increase of cycle period during oscillatory domain dynamics. A bar chart showing successively increasing periods of domain growth/dispersion cycles in GUVs (D) 42.0 μm, (E) 26.3 μm, and (F) 10.7 μm in diameter. A cycle period is defined as the time elapsed between two consecutive instances of appearance of uniform fluorescence. Except for control in (C), all data were collected using POPC:SM:Ch (1:1:1) GUVs, labeled with 0.5% Rho-DPPE, encapsulating 200 mM sucrose, diluted in deionized water at 25°C.
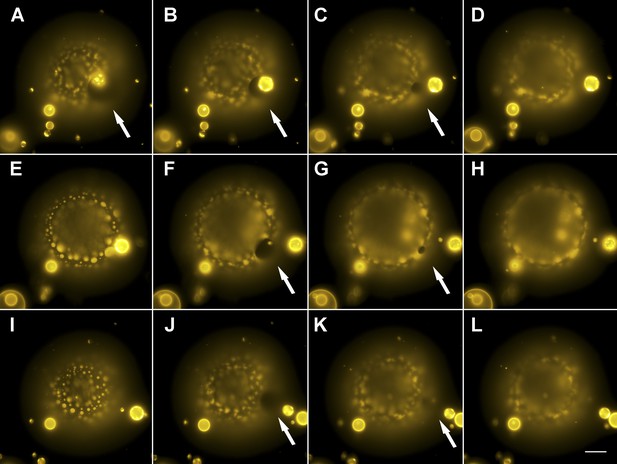
Evidence for the formation of microscopic pores during each individual cycle of oscillatory phase separation.
(A–L) Wide-field fluorescence images of microscopic pore formation (∼5–15 µm in diameter; indicated by arrows) observed in three consecutive swell–burst cycles of a phase-separating GUV. A single pore appears during each phase separation cycle and reseals within 1 s. The POPC:SM:Ch (1:1:1) GUV labeled with 0.5% Rho-DPPE (pseudo-colored yellow), encapsulates 200 mM sucrose, and is immersed in deionized water at 25°C. Images collected 20 min after imposition of the osmotic differential. Height-resolved (increment, 0.5 µm) images shown at arbitrary time intervals following the first frame. (A–L) 0 s, 0.3 s, 0.6 s, 0.9 s, 9.3 s, 9.6 s, 9.9 s, 10.1 s, 15.3 s, 15.6 s, 15.9 s, and 16.1 s. Scale bar: 15 µm. Cascades of pores have been observed more than five times.
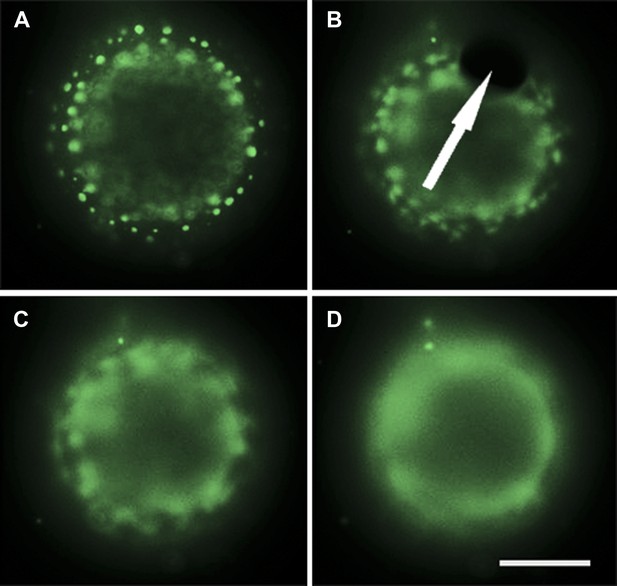
Evidence for pore-formation.
Selected frames from Video 7 showing the equatorial view of a POPC:SM:Ch (1:1:1) GUV labeled with 0.5% Rho-DPPE (pseudo-colored green), encapsulating 50 vol % glycerol, diluted in MilliQ water at 25°C. (A) The GUV is in a tense state exhibiting distinct domains. (B) Pore formation (indicated by the arrow) coincides with the onset of domain dispersion. (C) Further domain dispersion. (D) The GUV is returned to an optically homogenous state. Panels are taken with 0.5 s intervals. Scale bar: 5 μm.
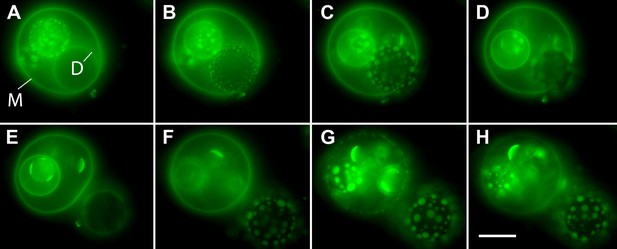
Osmotic gradients sensed by the membrane and visualized by oscillatory phase separation in nested vesosomes.
Selected frames from Video 7 showing hierarchical membrane structures of POPC:SM:Ch (2:2:1) GUVs labeled with 0.5% SM-Atto647N (pseudo-colored green), encapsulating 1 M sucrose, submerged in MilliQ water at 25°C. In panel (A), we define the entrapping mother vesicle as M and daughter vesicle of interest as D. Both M and D initially exhibit homogenous fluorescence from their membranes, but store different amounts of tension (M is flaccid, while D appears tense). (B) The homogeneous fluorescence from D is replaced by the appearance of optically resolved domains. In the meantime, M becomes more spherical. (C) The domains of D have increased in size, and M has now reached an almost spherical shape. (D) Expulsion of the tense D vesicle. This image acquired during a transient pore formation suggests that the intravesicular pressure and/or crowding is reduced via preferential expulsion of daughter. This event, we surmise, also delays the onset of domain formation by reducing the swelling of the M vesicle. (E) M is returned to a flaccid state, remaining homogenously fluorescent, consistent with the reduction in swelling and a reduction of osmotic pressure. At the same time, D experiencing a new hypotonic medium gets engaged in swell–burst cycles. (F) Further inflation of GUVs leads to M adopting a tense spherical configuration, while yet retaining homogenously fluorescent state, while D's domain sizes continue to grow. (G) The continued swelling of M finally leads to phase separation. (H) Domains in M disappear producing homogeneous state, consistent with the oscillatory phase separation under osmotically generated tension. Panels correspond to (A–H) 0 s, 6 s, 14 s, 18 s, 20 s, 62 s, 103 s, and 118 s. Scale bar: 10 µm.
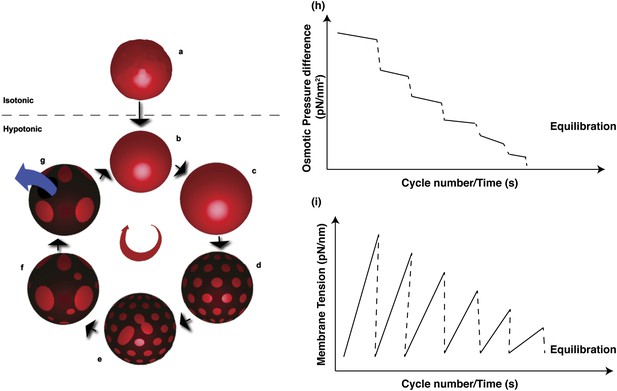
Schematic representations of physical mechanisms and changes in membrane properties during vesicular osmoregulation.
(Left panel) (A) GUV in isotonic medium exhibiting a flaccid morphology. (B–C) Immersion in a hypotonic bath initiates an osmotically triggered influx of water rendering the GUV tense. (D–F) The optically uniform vesicular surface breaks up into a pattern of microscopic domains, which grow by collision and coalescence. (G) Transient appearance of a microscopic pore (∼0.3–0.5 s lifetime), enabling solute efflux and tension relaxation, which drives pore closure, producing closed GUVs with a reduced osmotic differential and homogenous surface. Steps (B–G) repeat until the sub-lytic solute concentration differential is reached and the Laplace tension in the membrane is able to compensate for the residual osmotic pressure. (Right panel) Temporal cascades of osmotic pressure (H) and oscillations in membrane tension (I) during osmotic relaxation of giant vesicles subject to hypotonic bath. Note that the relative rates implied in the schematic are only best-guess estimates.
Videos
Thermally excited undulations of isotonic GUVs.
A video assembled from time-lapse fluorescence images revealing out-of-plane membrane fluctuations typical for non-tense GUVs in the absence of an osmotic gradient (50 vol% glycerol inside and outside). The osmotically balanced GUV consists of POPC:SM:Ch (1:1:1) labeled with 0.5 mol% Rho-DPPE (pseudo-colored magenta) and is imaged at 25°C.
Oscillatory domain dynamics in GUVs immersed in hypotonic bath.
Time-lapse images of a bottom view of GUVs consisting of POPC:SM:Ch (1:1:1) labeled with 0.5% Rho-DPPE (pseudo-colored magenta) under a net osmotic differential. The GUVs encapsulate 1 M sucrose in their interior, and the external dispersion medium is MilliQ water. A striking temporal pattern of oscillatory phase separation revealing appearance, coalescence, and dispersion of optically resolved domains is evident (see manuscript for details).
Domain dynamics of GUVs in hypotonic bath.
Video assembled from time-lapse images of Z-stack projections of the bottom hemispheres of GUVs, consisting of POPC:SM:Ch (1:1:1) labeled with 0.5 mol% Rho-DPPE (white). The vesicles encapsulated 200 mM sucrose and were diluted in MilliQ water at 25°C.
GUVs prepared by gentle hydration reproduce the oscillatory domain dynamics.
GUVs prepared by ‘gentle hydration’ consisting of POPC:SM:Ch (1:1:1) labeled with 0.5 mol% Rho-DPPE (pseudo-colored blue), encapsulating 200 mM sucrose. Hypotonic conditions are established by dilution in deionized water at 25°C. Vesicles exhibit osmotic swelling and oscillatory domain dynamics comparable to that seen for electroformed GUVs under the same conditions.
Evidence for domain merger by collision and coalescence.
Time-lapse wide-field fluorescence images of the lower hemisphere of POPC:SM:Ch (1:1:1) GUVs labeled with 0.5 mol% Rho-DPPE (pseudo-colored yellow), encapsulating 200 mM sucrose, diluted in MilliQ water at 23°C. Domain–domain coalescence, followed by line-tension driven shape transformations, drives domain growth. Frames are collected at 1 s intervals.
Oscillatory domain dynamics in mixed ternary system known to exist in single liquid state in the absence of net osmotic differential.
Time-lapse wide-field fluorescence images of DOPC:DPPC:Ch (5:2:3) GUVs labeled with 0.5 mol% Rho-DPPE (pseudo-colored yellow), encapsulating 200 mM sucrose, diluted in MilliQ water at 23°C. Frames are collected at 1 s intervals.
Evidence for pore formation.
Equatorial view of a POPC:SM:Ch (1:1:1) labeled with 0.5 mol% Rho-DPPE (pseudo-colored green), encapsulating 50vol % glycerol, diluted in MilliQ water at 25°C. Pore formation can be clearly seen at 1.1 s, just prior to the disappearance of domains and size shrinkage of the GUV.
Oscillatory phase separation during expulsion of daughter GUVs.
A video assembled from time-lapse fluorescence images of phase-separating GUVs containing internal ‘organelle’ vesicles. GUVs consisting of POPC:SM:Ch (2:2:1) labeled with 0.5 mol% Sphingomyelin-Atto647N (SM-647N) (pseudo-colored green), encapsulate 1 M sucrose, and diluted in MilliQ water at 25°C. The video reveals shifting patterns of osmotic pressure and tension during expulsion of the internal vesicles after an osmotic differential had been established. Key steps include (A) a homogeneous, flaccid mother vesicle encapsulating tense daughter vesicles, at a time point prior to vesicle expulsion; (B) just after expulsion, the daughter GUV remains tense exhibiting oscillatory phase separation, while the mother GUV is left deflated and homogenous due to the sudden loss of volume; (C) The mother GUV subsequently becomes inflated by influx of water; and (D) the mother GUV begins to exhibit oscillatory phase separation.