Decoding a neural circuit controlling global animal state in C. elegans
Figures
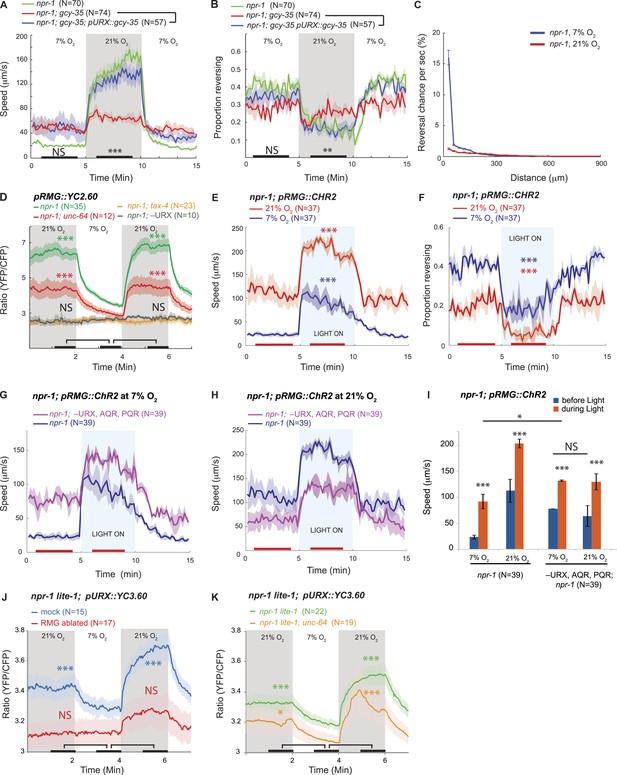
RMG activation induces rapid and persistent forward movement.
(A and B) URX O2 sensors provide an entry point to the circuit controlling response to 21% O2. Selective expression of GCY-35 in URX neurons restores rapid (A) and persistent (B) forward movement at 21% O2 to gcy-35; npr-1 animals on food. Statistics compare rescued (blue) and mutant (red) animals at time points indicated by the black bars. (C) 21% O2 causes npr-1 animals on food to suppress the short, frequent reversals observed at 7% O2. Reversal probability is calculated per 1 s. (D) Ablating URX abolishes the Ca2+ responses evoked in RMG by 21% O2; unc-64 syntaxin loss-of-function mutants show a partial reduction in this response. Here, and in subsequent panels, black bars indicate time intervals for statistical comparison of responses at 21% and 7% O2 using the Mann–Whitney U test. ***p<0.001; **p<0.01; *p<0.05; NS, not significant. (E and F) Stimulating RMG using channelrhodopsin evokes rapid movement (E) and inhibits backward movement (F) in npr-1 animals kept at either 7% or 21% O2 with food. Here and in subsequent panels, red bars indicate time intervals used for statistical comparisons of responses when light is on to when it is off. ***p<0.001, **p<0.01; *p<0.05; NS, not significant. (G–I) Channelrhodopsin stimulation of RMG can induce rapid movement when AQR, PQR, and URX neurons are ablated. Data plotted in (I) are replotted from G and H. (J) URX Ca2+ responses evoked by 21% O2 are strongly attenuated when RMG is ablated. (K) URX neurons retain O2-evoked Ca2+ responses in unc-64 syntaxin mutants, although baseline Ca2+ is reduced. Each line in this panel (and, unless specified, in subsequent panels) represents the mean response of all animals of one genotype or condition. Error bars (lighter shading) in all panels show standard error of the mean. Gray areas indicate periods of higher O2 concentration; blue areas indicate periods with blue light (0.26 mW/mm2) on; orange areas indicate periods with green light (0.64 mW/mm2) on.
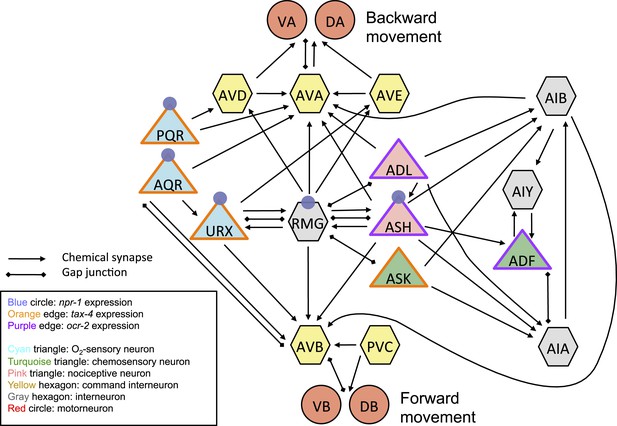
Simplified circuitry associated with the URX, AQR, and PQR neurons dissected in this paper.
https://doi.org/10.7554/eLife.04241.004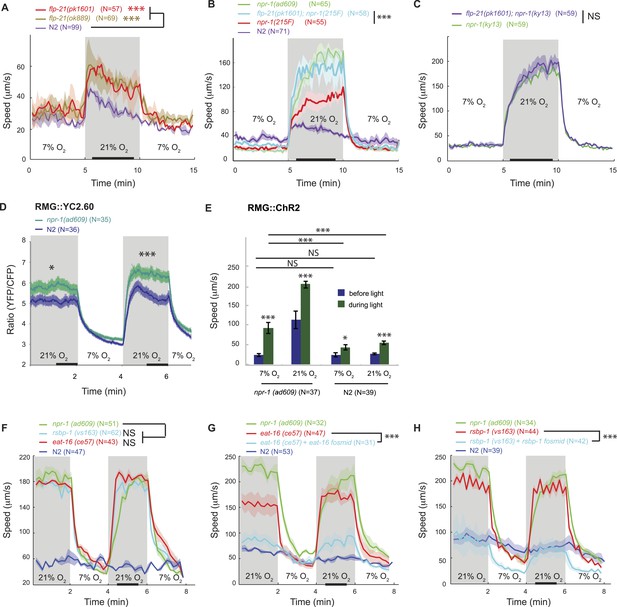
FLP-21/NPR-1 ligand/receptor signaling limits RMG circuit output downstream of RMG cell body Ca2+.
(A–C) Disrupting the flp-21 FMRF-like neuropeptide enables N2 animals to respond to 21% O2 with a persistent increase in locomotory activity (A), and increases the amplitude of such responses in animals expressing the natural npr-1 215F receptor allele (B) but not in npr-1(ky13null) mutants (C). (D) The npr-1 215V allele only slightly reduces the Ca2+ responses evoked by 21% O2 in RMG. (E) RMG stimulation using ChR2 only weakly stimulates movement in N2 animals, both at 7% and 21% O2, contrasting with its effects in npr-1 animals. (F–H) Knocking out eat-16, a member of the RGS7 family that inhibits Gq signaling, or its interacting partner rsbp-1, enables N2 animals to switch to rapid movement at 21% O2.
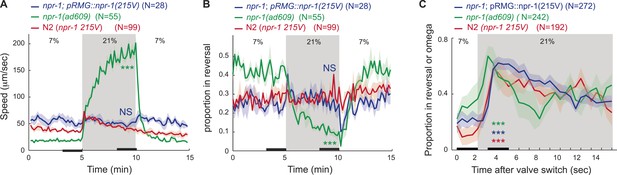
npr-1 GPCR signaling inhibits long-lasting but not transient responses to 21% O2 in feeding animals.
npr-1 215V signallingsignaling in RMG prevents 21% O2 from evoking persistent rapid movement (A), and sustained inhibition of reversals (B), but does not affect the initial bout of reversals evoked by a rise in O2 (C).
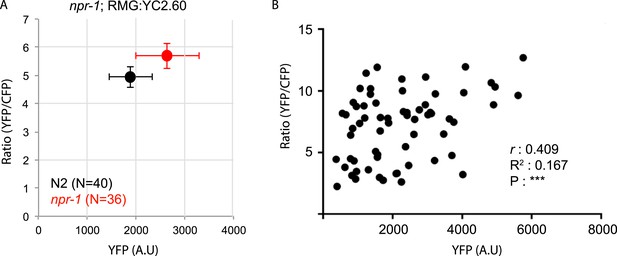
Differences in YC2.60 sensor expression may partially account for differences in YFP/CFP ratios in RMG between N2 and npr-1 animals.
(A) Average YFP/CFP ratios in RMG at 21% O2 in N2 animals and npr-1 mutants plotted against average YFP expression levels. (B) Scatter plot of YFP/CFP ratios in RMG at 21% O2 against YFP expression levels for individual animals shows a positive correlation. ***, p < 0.001. YC2.60 expression is restricted to RMG using a Cre–Lox combination in which sensor expression is driven from the flp-21 promoter (see ‘Materials and methods’).
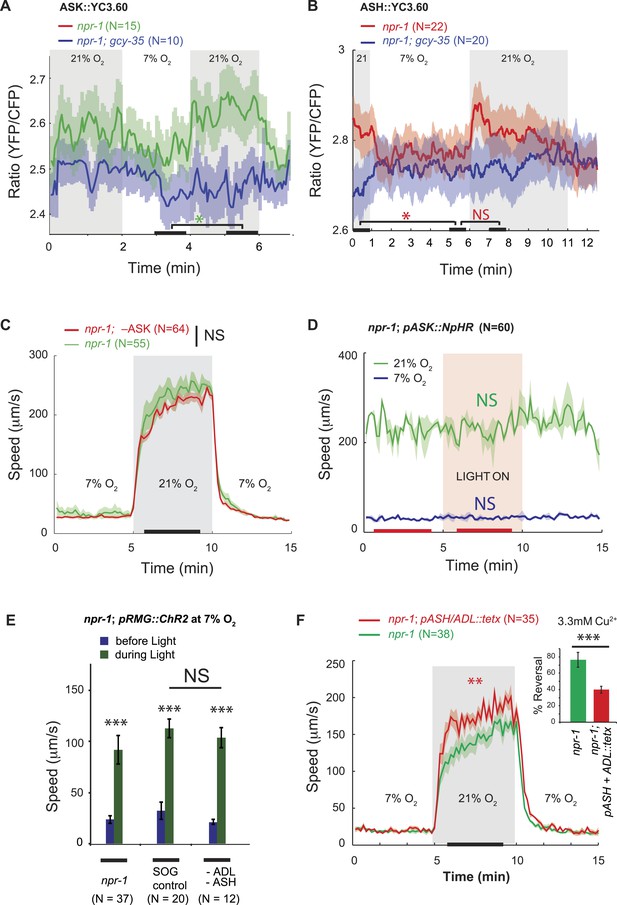
ASK, ASH, and ADL neurons are not necessary for the RMG circuit to stimulate rapid movement at 21% O2.
(A and B) In npr-1 animals a 7% O2 to 21% O2 stimulus evokes a small rise in Ca2+ in ASK (A) and ASH (B) neurons. These responses are abolished in gcy-35; npr-1 animals. (C and D) Ablating ASK (C), or acutely inhibiting its activity using halorhodopsin (D) did not alter the locomotory behavior of npr-1 animals on food at 7% or 21% O2. (E) Stimulating RMG using ChR2 can stimulate locomotion in npr-1 animals kept at 7% O2 in the absence of ASH and ADL neurons. (F) Inhibiting synaptic release from ASH and ADL using tetanus toxin disrupts avoidance of Cu2+ (inset) but does not inhibit the behavioral state switch evoked by changing O2.
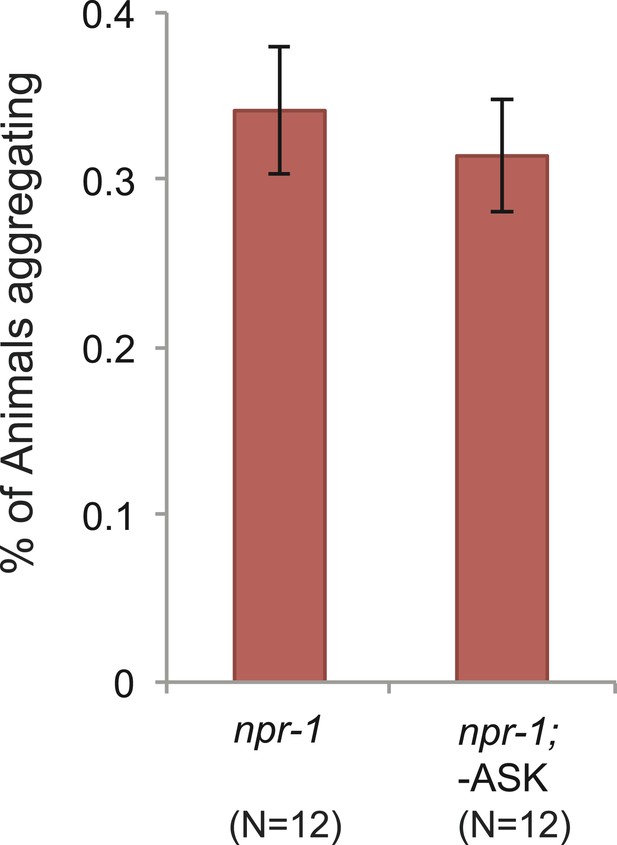
Ablating ASK neurons does not reduce aggregation behavior of npr-1 animals.
https://doi.org/10.7554/eLife.04241.009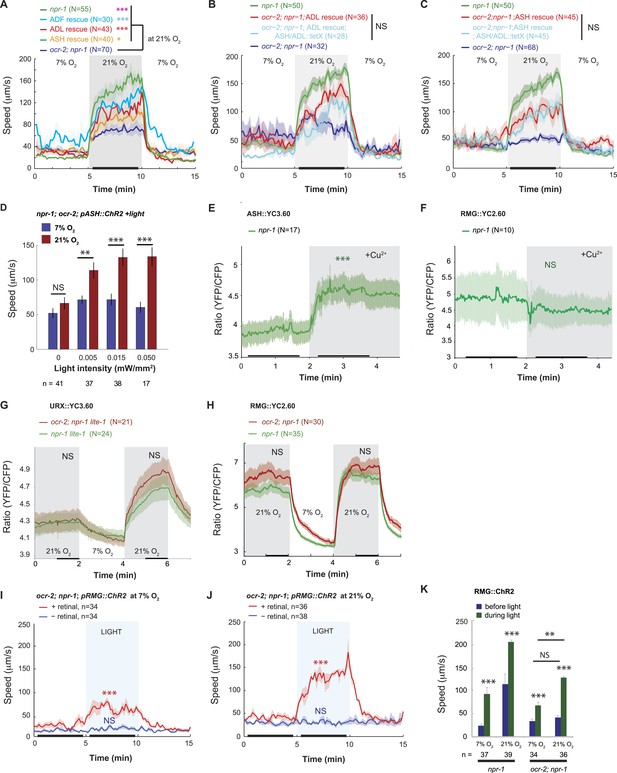
Disrupting a TRPV channel in ASH, ADL, and ADF neurons attenuates locomotory responses to 21% O2.
(A) The switch to rapid movement evoked in npr-1 animals by 21% O2 is attenuated in the absence of the OCR-2 TRPV channel. This defect of ocr-2; npr-1 animals can be rescued to varying extents by expressing ocr-2 cell-specifically in ASH, ADL, or ADF neurons. (B and C) The ability of pADL::ocr-2 (B) or pASH::ocr-2 (C) transgenes to rescue the ocr-2; npr-1 O2 phenotype does not depend on synaptic release. Synaptic release was inhibited by expressing tetanus toxin in ASH and ADL neurons, using the gpa-11 promoter. (D) Channelrhodopsin stimulation of ASH neurons restores modulation of locomotory activity by 21% O2 to ocr-2; npr-1 animals. (E and F) 2 mM Cu2+ elicited strong Ca2+ responses in ASH neurons of N2 and npr-1 animals (E) but did not alter Ca2+ in the RMG neurons (F). (G and H) Disrupting ocr-2 did not significantly reduce O2-evoked Ca2+ responses in the cell bodies of URX (G) or RMG (H) neurons. (I–K) Stimulating RMG in ocr-2; npr-1 animals kept on food using channelrhodopsin can partly restore O2 modulation of locomotory activity.
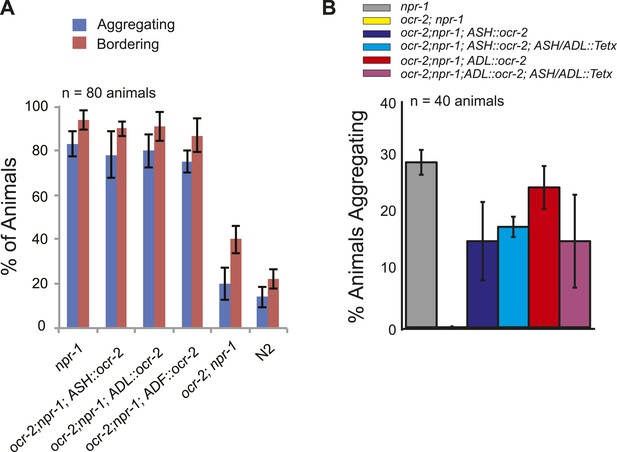
OCR-2 expression in ASH or ADL can restore aggregation behavior to ocr-2; npr-1 animals (A) even when synaptic transmission in these neurons is inhibited by tetanus toxin expression (B).
https://doi.org/10.7554/eLife.04241.011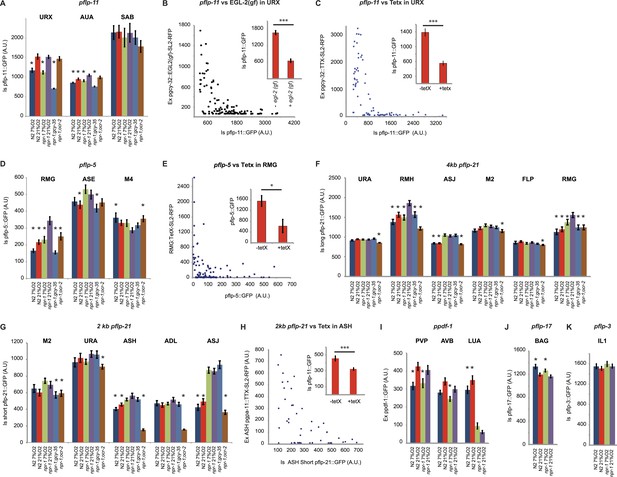
Neuropeptide gene expression levels report neurosecretory activity.
(A) Expression of a pflp-11 reporter in URX, AUA, and SAB neurons in different genotypes and at different O2 tensions. In this and subsequent panels, AU = arbitrary units. (B) Expression of pflp-11::GFP in URX is inversely related to expression of a gain-of-function K+ channel, EGL-2(GF), in the same neuron. Expression of the K+ channel can be tracked due to co-expression of RFP in an operon. (C) Blocking exocytosis from URX by targeted expression of tetanus toxin strongly reduces expression of pflp-11::GFP in URX. Expression of tetanus toxin can be tracked due to co-expression of RFP in an operon. (D) Expression of a pflp-5 reporter in RMG, ASE, and M4 in different genotypes and at different O2 tensions. (E) Blocking exocytosis from RMG by targeted expression of a tetanus toxin strongly reduces expression of pflp-5::GFP in RMG. Expression of tetanus toxin can be tracked due to co-expression of RFP in an operon. (F) Expression of a pflp-21 reporter that includes 4 kb of upstream sequences in URA (probably), RMH, ASJ, M2, FLP, and RMG neurons in different genotypes and at different O2 tensions. (G) Expression of a pflp-21 reporter that includes 2 kb of upstream sequences in M2, URA (probably), ASH, ADL, and ASJ neurons in different genotypes and at different O2 tensions. (H) Blocking exocytosis from ASH by targeted expression of a tetanus toxin reduces expression of pflp-21 (2 kb)::GFP in ASH. Expression of tetanus toxin can be tracked due to co-expression of RFP in an operon. (I–K) Expression of ppdf-1::gfp (I), pflp-17::gfp (J) or pflp-3::gfp (K) reporters in different neurons in different genotypes at different O2 concentrations. For (A, D, F, G, I, J, and K), asterisks indicate comparisons to npr-1 animals kept at 21% O2, *p<0.05; ***p<0.001.
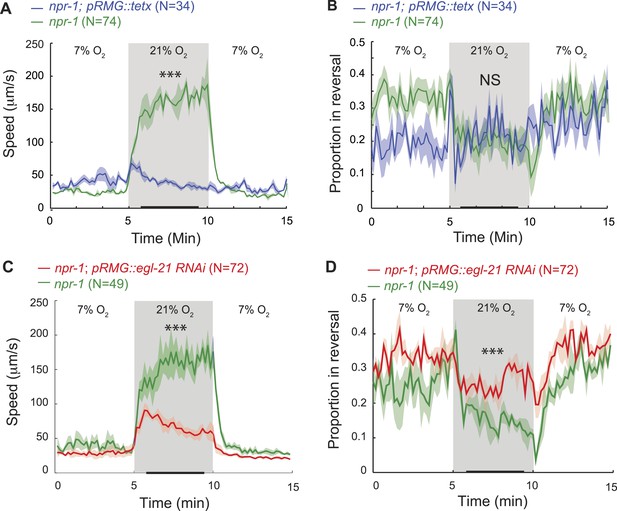
RMG neuropeptide secretion drives rapid movement at 21% O2.
(A–D) Selective expression of tetanus toxin (A and B) or RNAi knockdown of EGL-21 carboxypeptidase E (C and D) in RMG inhibits the O2-evoked switch in locomotory state. NS, not significant; ***p<0.001.
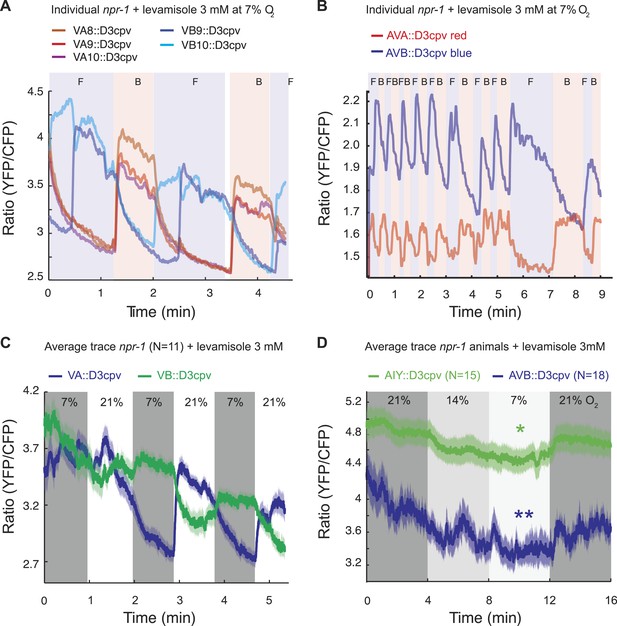
Spontaneous and O2-evoked Ca2+ responses in interneurons and motoneurons in immobilized animals.
(A and B) Spontaneous bi-stable Ca2+ responses observed in VA and VB neurons (A) and in AVA and AVB neurons (B) in individual npr-1 animals immobilized with 3 mM levamisole and kept at 7% O2. As expected, Ca2+ responses in VAs versus VBs (A) and AVA versus AVB (B) neurons were anti-correlated. Different colored shading and F and B indicate fictive forward (F) or backward (B) movement. (C) On average, an upstep from 7% to 21% O2 evoked a Ca2+ response in the VAs neurons of npr-1 animals immobilized with 3 mM levamisole. (D) Despite stochastic, high amplitude changes in Ca2+ levels of AIY and AVB neurons, on average, higher [O2] correlated with higher Ca2+ levels in AIY and AVB in animals immobilized with 3 mM levamisole.
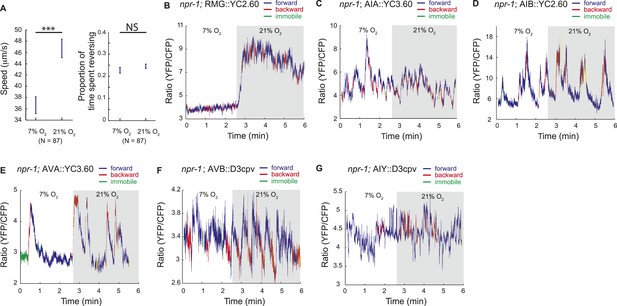
Correlation of neural activity and behavior at 7% and 21% O2.
(A) Under our Ca2+ imaging conditions, freely moving npr-1 animals increase their speed but do not suppress reversals at 21% O2. (B–G) RMG neurons respond to 21% O2 with a strong persistent increase in Ca2+ regardless of direction of travel (B). By contrast, freely moving npr-1 animals display frequent brief Ca2+ changes in AIA (C), AIB (D), AVA (E), AVB (F), and AIY neurons (G), at both 7% and 21% O2. Most Ca2+ changes are associated with reversal events.
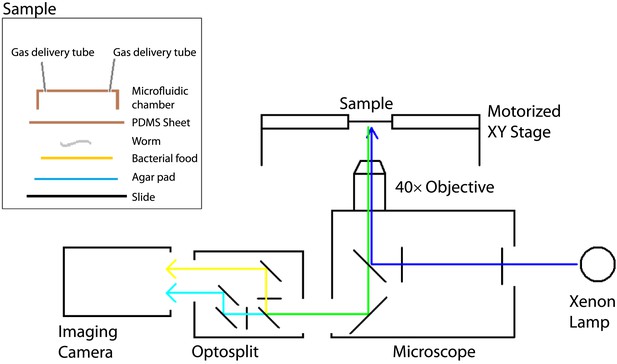
The experimental setup used to image freely moving animals.
https://doi.org/10.7554/eLife.04241.016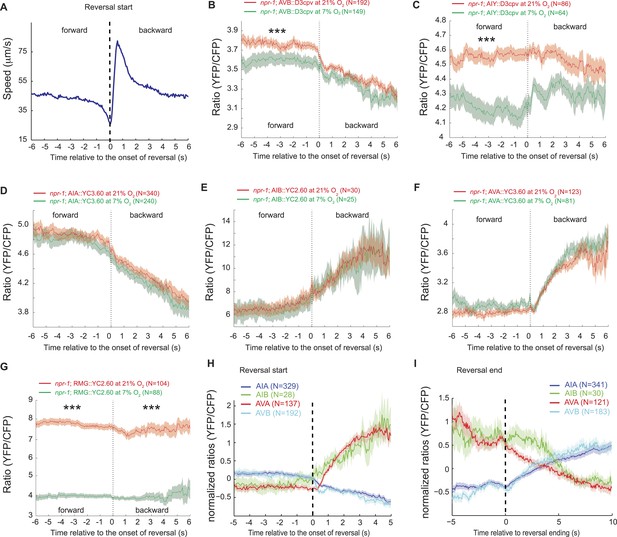
AVB and AIY interneurons integrate information about O2 levels with other input.
(A) Animals showed a characteristic pattern of speed changes when traces were aligned according to the time of reversal initiations. (B–G) AVB (B), AIY (C), and RMG neurons (G) show increased Ca2+ at 21% O2 compared to 7% O2 during forward movement. By contrast, average Ca2+ in AIA (D), AIB (E), and AVA (F) was not significantly modulated by O2. (H and I) Normalized Ca2+ traces in AVA, AVB, AIA, and AIB neurons aligned to the first frame of backward locomotion (H), or to the first frame of forward movement (I), during spontaneous reversals. Reversal initiation correlates with a rise in Ca2+ in AIB and AVA and a fall in Ca2+ in AIA and AVB. The converse pattern is observed when reversals are terminated.
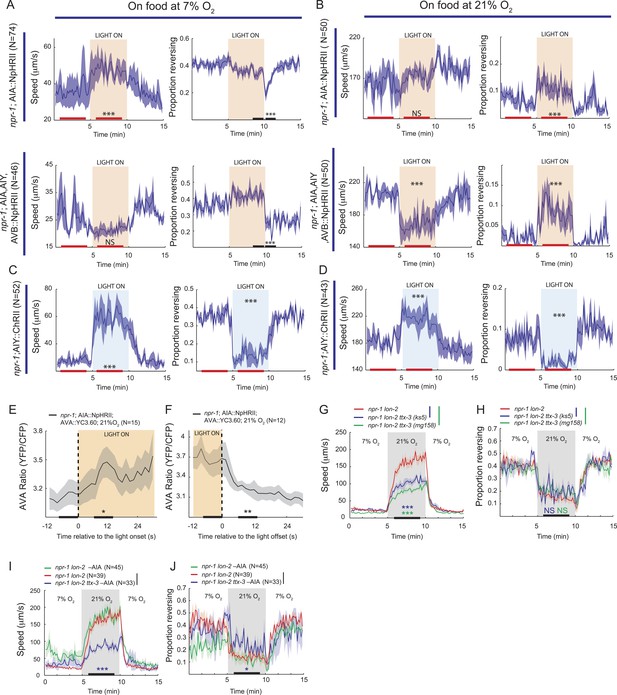
AIY and AVB interneurons contribute to the switch in locomotory activity evoked by 21% O2.
(A and B) Behavioral effects of inhibiting AIA or AIA, AIY and AVB using halorhodopsin in animals kept at 7% O2 (A) or 21% O2 (B). (C and D) Behavioral effects of activating AIY neurons using channelrhodopsin in animals kept at 7% O2 (C) or 21% O2 (D). (E and F) Ca2+ responses evoked in AVA interneurons by inhibition (E) or disinhibition (F) of AIA neurons using halorhodopsin. (G and H) Disrupting the ttx-3 homeobox transcription factor required to specify AIY cell fate attenuates the rapid movement evoked in npr-1 animals by 21% O2 but not the inhibition of reversals. ttx-3 (ks5) and ttx-3(mg158) are different null alleles. (I and J) Ablating AIA neurons alone does not disrupt responses of npr-1 animals to 21% O2. npr-1 ttx-3 mutants ablated for AIA neurons both move more slowly and reverse more frequently than controls.
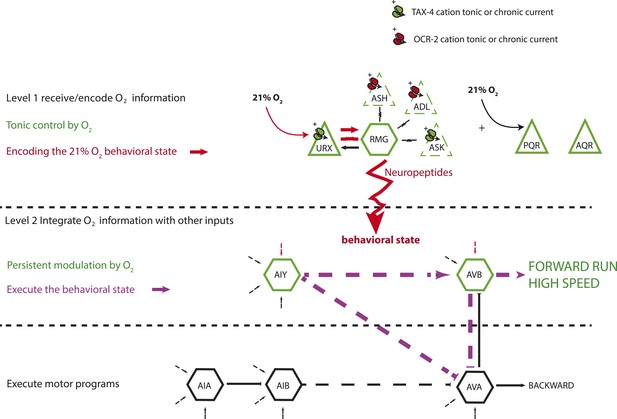
A model for the circuits controlling the behavioral switch.
The URX O2-sensing neurons and the RMG interneurons tonically encode O2 concentration. These neurons communicate to downstream neurons predominantly through sustained changes in neuropeptide secretion. RMG is connected through gap junctions to sensory neurons that can act as shunts, downregulating RMG neurosecretion when the OCR-2 or TAX-4 cation channels they express are less active. URX–RMG output continuously modulates the activity of downstream neurons, including AIY and AVB, but these neurons also respond to other cues, and their activity at any time point reflects both O2 concentration and the behavior being executed. Increased activity of these neurons promotes rapid forward movement. Other neurons, such as AIA and AVA, participate in the execution of the behavioral state but do not appear to be under tonic control of the URX–RMG circuit.
Additional files
-
Supplementary file 1
Comparison of RNA sequence data from npr-1 young adults grown at 21% and 7% O2.
- https://doi.org/10.7554/eLife.04241.020
-
Supplementary file 2
Comparison of RNA sequence data from gcy-35; npr-1 and npr-1 young adults grown at 21% O2.
- https://doi.org/10.7554/eLife.04241.021
-
Supplementary file 3
Comparison of RNA sequence data from gcy-35; npr-1 young adults grown at 21% and 7% O2.
- https://doi.org/10.7554/eLife.04241.022
-
Supplementary file 4
List of genes differentially expressed in Supplementary file 1 (npr-1 21% O2 vs npr-1 7% O2) and Supplementary file 2 (gcy-35; npr-1 21% O2 vs npr-1 21% O2) but not Supplementary file 3 (gcy-35; npr-1 21% O2 vs gcy-35; npr-1 7% O2).
- https://doi.org/10.7554/eLife.04241.023
-
Supplementary file 5
Lists of strains and constructs.
- https://doi.org/10.7554/eLife.04241.024