Stepwise visualization of membrane pore formation by suilysin, a bacterial cholesterol-dependent cytolysin
Figures
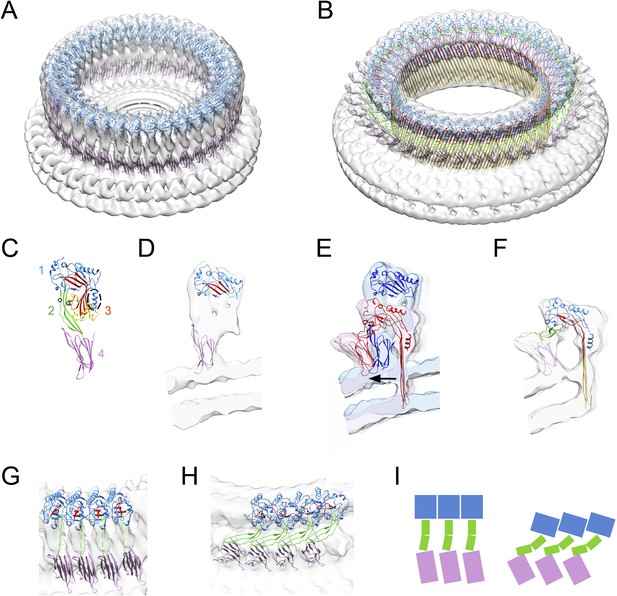
Structural transitions during pore formation.
3D cryo-EM maps of 37-mer prepore and pore forms of suilysin are shown with fitted atomic structures. (A) Density map of prepore, surrounded by the extracted disk of membrane, with domains 1 and 4 fitted. (B) Density map of pore with all domains fitted, including the β-barrel with strands at 20° tilt. (C) Suilysin crystal structure with the domains labelled, showing positions of cysteines introduced in the locked form (black circles) and a helical domain adjacent to the bend in the central β-sheet (dashed oval). (D) Cross-section through one side of the prepore map with the partial fit of atomic structures. (E) Overlay of one side of the prepore (blue) and pore maps (red), aligned to the same centre, showing the displacement of domain 4 (arrow). (F) Pore section with fit. (G) View of 4 subunits from outside the prepore. (H) View of 4 subunits from outside the pore. (I) Cartoons of domain packing in prepore and pore.
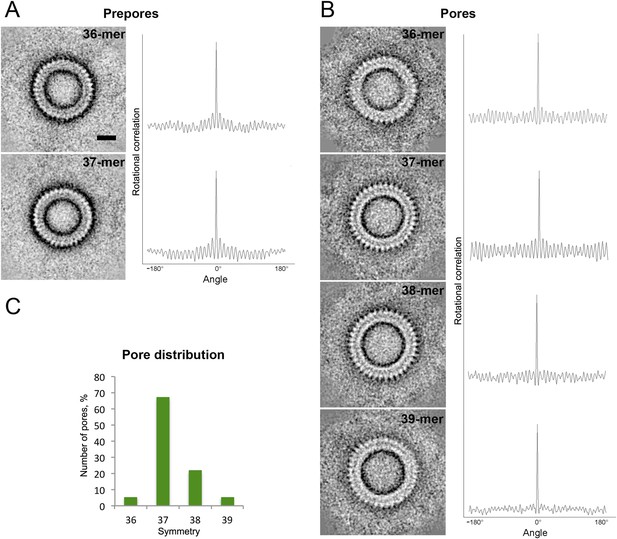
Symmetry of suilysin prepores and pores, determined by negative-stain EM.
(A) Averaged views of 36-fold (upper row) and 37-fold (lower row) symmetric prepores with their rotational autocorrelations. (B) Averaged views of 36-fold (first row), 37-fold (second row), 38-fold (third row), and 39-fold (fourth row) symmetric pores with their rotational autocorrelations. (C) Plot of pore distribution vs symmetry. Scale bar (see A, upper row): 10 nm.
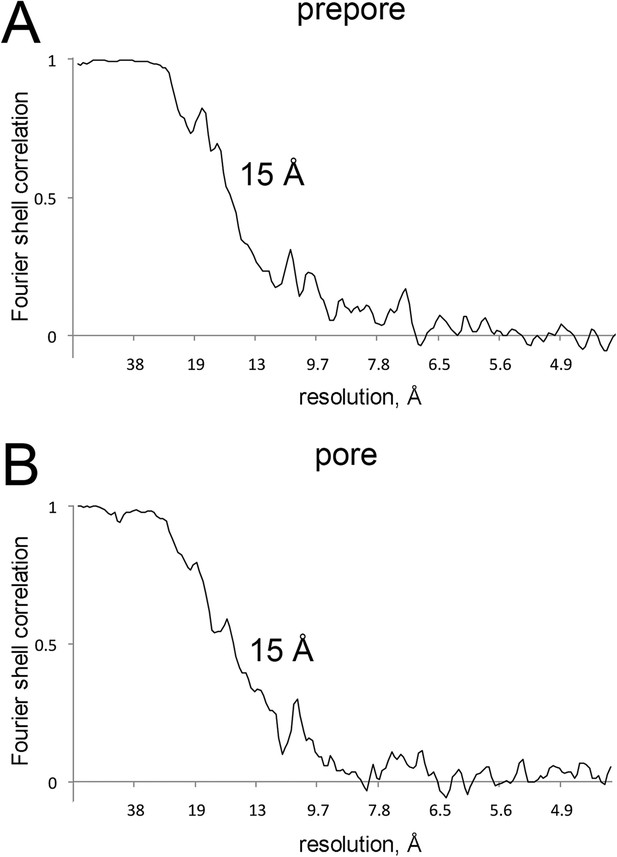
Resolution curves for EM maps.
(A and B) Fourier-shell correlation curves reporting 15 Å resolution at 0.5 correlation for the EM maps of prepore and pore.
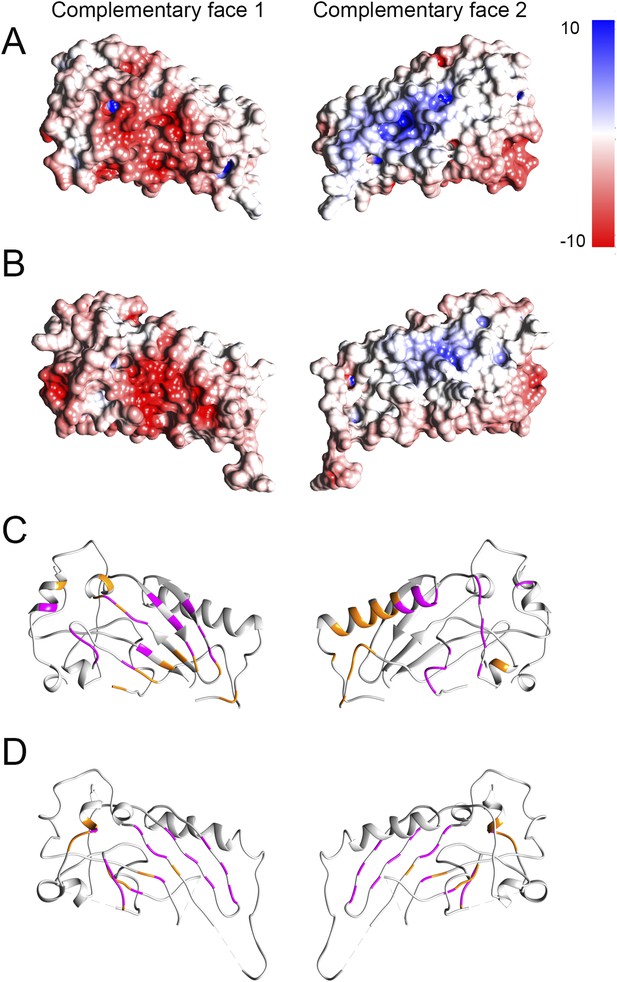
Electrostatic potential maps and interacting residues.
(A and B) Electrostatic potential mapped onto the surfaces in crystal structures of domain 1 fitted into the prepore (A) and pore (B) maps respectively. Red and blue colored regions denote negative and positive, colored by charges, respectively according to the color scale bar. (C and D) Interacting residues between a given dimer of domain 1 (shown in orange) mapped onto the crystal structure in the prepore (C) and pore (D) respectively. We consider two residues as interacting (interface residue) if their corresponding Cβ atoms were found within the distance of 7 Å (Malhotra et al., 2014). 32 common interacting residues (shown in magenta) for domain 1 were identified by comparing the interacting residues of the corresponding prepore and pore dimers, suggesting that approximately 50% of the interface is preserved between the prepore and pore fit.
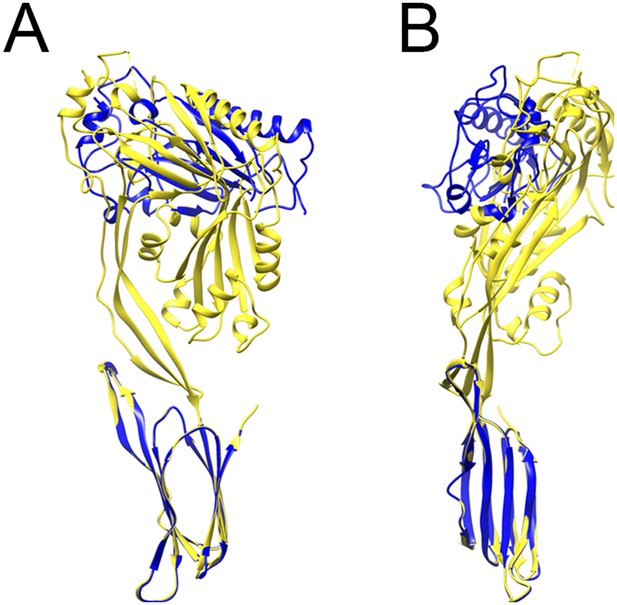
Comparison between prepore and crystal structure conformations.
Overlays of the monomer structure in the crystal (yellow) and in the partial model of the prepore (blue), seen from the oligomer interface (A) and from the outside of the ring (B), showing a sideways tilt and inward rotation of domain 1.
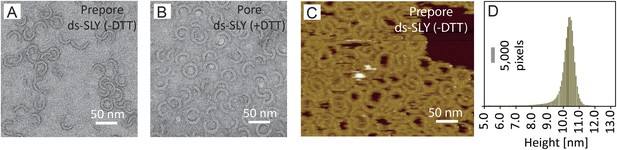
Negative-stain EM and AFM of disulphide-locked suilysin.
(A) Negative-stain EM disulphide-locked suilysin (ds-SLY) on egg PC:cholesterol monolayers (45:55%), locked in the prepore state (−DTT). (B) as (A), for disulphide-locked suilysin incubated in the presence of 5 mM DTT in solution to reduce the disulphide bridge, so that the suilysin is rapidly converted to the pore conformation. (C) AFM of densely packed suilysin prepores, confined to the egg PC-rich domain of a phase-separated egg PC:DDAB:Cholesterol (33:33:33%) supported lipid bilayer, with its corresponding height distribution (D) referenced to the membrane surface.
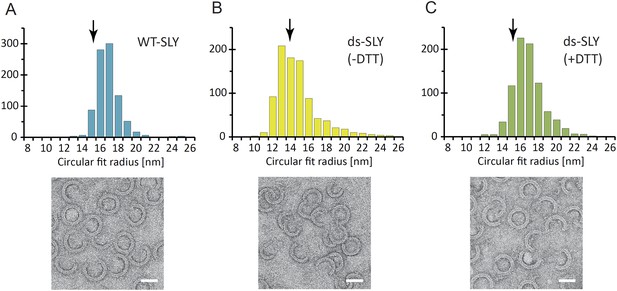
Radius of curvature for arc-shaped suilysin assemblies in the prepore and pore states.
(A) For wild-type suilysin (WT-SLY), the curvature distribution of the arc-shaped assemblies shows a sharp peak close to the radius of the complete ring with 37-fold symmetry. (B) For disulphide-locked suilysin in the prepore state (ds-SLY, −DTT), the curvature distribution peaks at slightly lower radius but also shows a larger spread to radii of curvature far exceeding that of the complete 37-mer ring. (C) When the disulphide bridge is unlocked by DTT (ds-SLY, +DTT, cf. Figure 2B), the insertion of the transmembrane hairpins in the lipids and formation of the β-barrel leads to a shaper distribution of the arc-shaped oligomers, similar to the wild-type suilysin. Corresponding negative stain EM views are shown under each plot. These observations are further evidence that the prepore intermediate is a structurally flexible state. The arrows refer to the circular fit radius to a 37-mer suilysin ring, which corresponds to 13.9 nm for the prepore assembly and 15.1 nm for the pore state assembly. Scale bars: 25 nm.
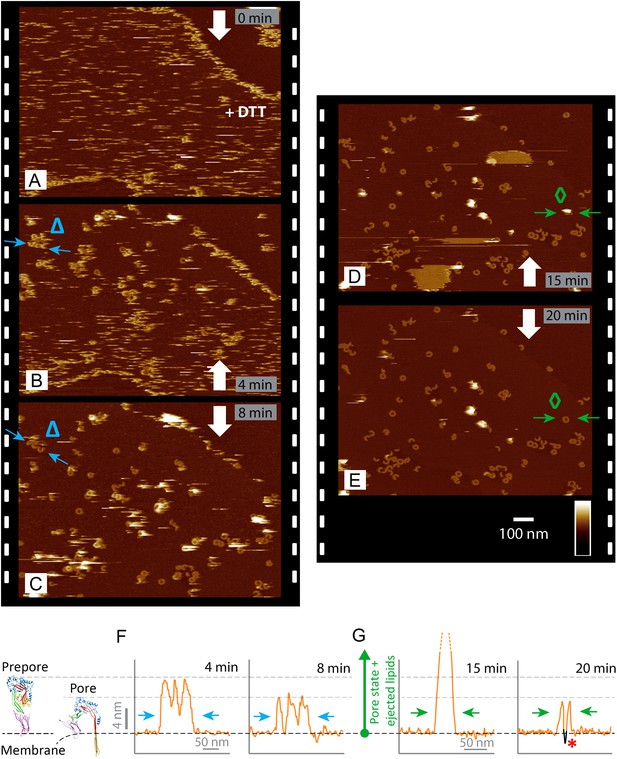
Real-time imaging of the prepore-to-pore transition and membrane perforation by suilysin.
Subsequent AFM frames of the same area were alternatively recorded from top to bottom and from bottom to top, as indicated by white arrows. Frame time: 4 min, colour scale: 35 nm. (A) Loosely bound to sphingomyelin-rich domains in the phase-separated lipid mixture (DOPC:sphingomyelin:cholesterol, 33:33:33%), the prepore intermediates of disulphide-locked suilysin appear as diffuse streaks. 5 mM of DTT is injected on about 50% completion of the scan. (B) On consecutive scanning, the streaks become more clearly defined as arc-shaped oligomers and complete rings. Towards the top end of the scan, clusters of arc-shaped complexes, mostly in the prepore intermediate (∼10.5 nm high), can be distinguished (Δ). (C) With the scan direction reversed, and the same area scanned again, the cluster of prepore complexes has converted into the pore state (∼7.5 nm high), within ∼2 min. The prepore to pore transition is followed by the ejection of globular features of varying dimensions exceeding 15 nm above the suilysin in the pore state. We interpret these as ejected lipids. (D) These lipids gradually detach from the surface on the pore state suilysin assemblies and can be observed as patches of lipids condensing back onto the membrane. The prepore to pore transition of the suilysin is now complete. (E) After ∼20 min, the surface is almost clear of the ejected lipids. (F) Cross-sectional line profile extracted as indicated (Δ in B–C), illustrating the prepore to pore transition. (G) Cross-sectional line profile extracted as indicated (◊ in D–E), illustrating the lipid ejection and eventual formation of an aqueous pore in the membrane (*).
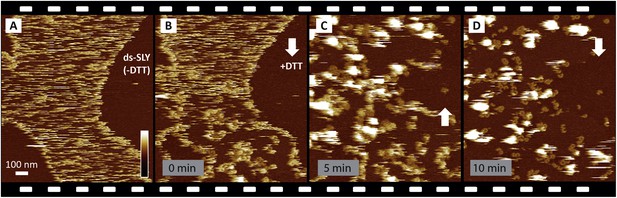
Reproducibility of real-time imaging of the prepore-to-pore transition and membrane perforation by suilysin.
Recorded in a separate experiment but otherwise similar to Figure 3, a sequence of AFM images was captured from top to bottom and from bottom to top, as indicated by white arrows. (A) Prepore intermediates of ds-SLY appear as diffuse streaks on the sphingomyelin-rich phase of the membrane. (B) Upon injection of 5 mM of DTT at about 25% of the way through the scan, the diffuse streaks gradually disappear to be replaced by more clearly resolved arcs and rings of SLY. The brighter assemblies measure ∼10.5 nm in height corresponding to the prepore intermediate state, next to the pore state suilysin which is ∼7.5 nm in height. (C) In the next scan, ∼6 min after DTT injection, large globular features are observed, as in Figure 3C,D, which we interpret as ejected lipids. (D) Some lipids detach into the supernatant revealing the pore state suilysin assemblies. Frame time: 5 min, full z-colour scale: 35 nm.
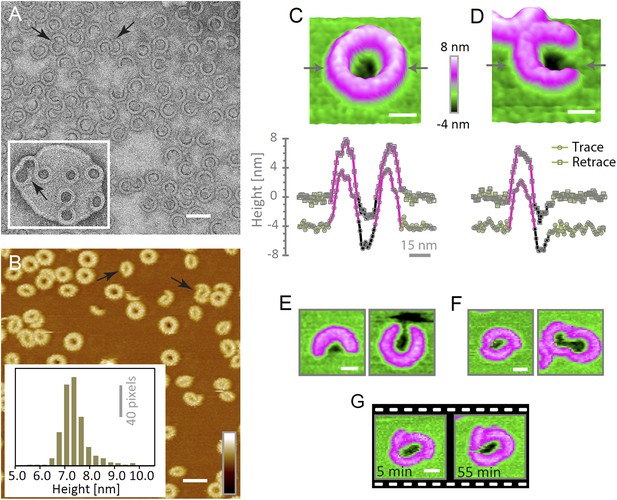
Suilysin assembles into ring- and arc-shaped oligomers that perforate the membrane.
(A) Negatively stained EM of arc- and ring-shaped assemblies of wild-type suilysin on an egg PC:cholesterol (45:55%) lipid monolayer, and (inset) on a liposome of egg PC:cholesterol (45:55%). (B) AFM topography of wild-type suilysin on a supported egg PC:cholesterol (67:33%) lipid bilayer. The wild-type suilysin extends 7–8 nm above the lipid bilayer background, as indicated by the height histogram for 402 individual particles (inset). (C) The AFM topography of a complete suilysin ring reveals a circular hole (dark) in its lumen, whereas the lipid bilayer surrounding the ring remains intact (green). (D) The topography of a suilysin arc shows a hole (dark) in the membrane only partially enclosed by the suilysin assembly. Images in C and D are shown in a 15° tilted representation, and height profiles measured across the ring/arc confirm membrane perforation. (E) Examples of wild-type suilysin arcs of different lengths. Transmembrane holes are consistently observed. (F) Examples of interlocked-arc assemblies. As shown in the right image, the membrane area removed by the two arcs is larger than the hole in the complete ring (C). (G) Sequence of AFM images of the same interlocked-arc assembly, stable for at least 50 min. Scale bars A–B: 50 nm, C–G: 15 nm, full z colour scale B–G: 12 nm.
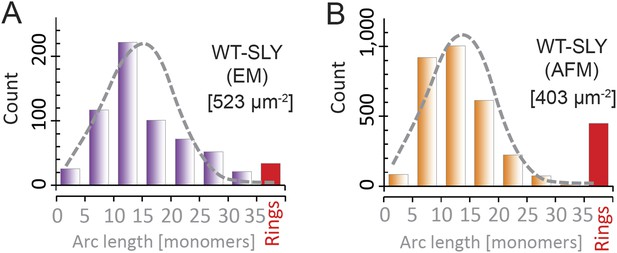
Suilysin pore assemblies by EM and AFM.
The arc-length distributions for wild-type suilysin as measured by negative-stain EM on monolayers (A) and the corresponding AFM data on supported lipid bilayers (B). The grey dashed curves denote the fits of the experimental data with the oligomerization model with ka/kb = 3.461 ± 0.019 µm2 (A), and 3.468 ± 0.004 µm2 (B). The numbers in brackets in (A) and (B) denote the number of monomers per square micron. When using similar lipid compositions (egg PC:Cholesterol 67:33%) and incubation conditions (27°C), both experiments yield very similar distributions.
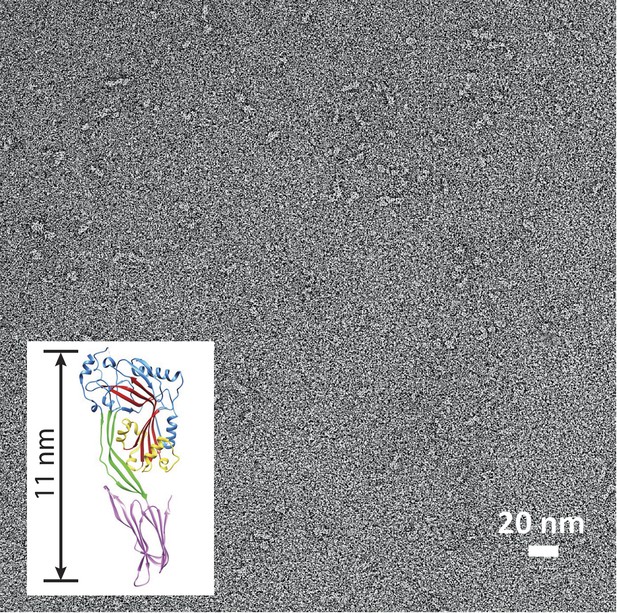
Suilysin is a monomer in solution.
Negative-stain EM of a carbon grid after incubation with wild-type suilysin at a concentration of 10 µg/ml. In the absence of lipids, only monomers are observed. Inset: crystal structure of suilysin (Xu et al., 2010), for comparison.
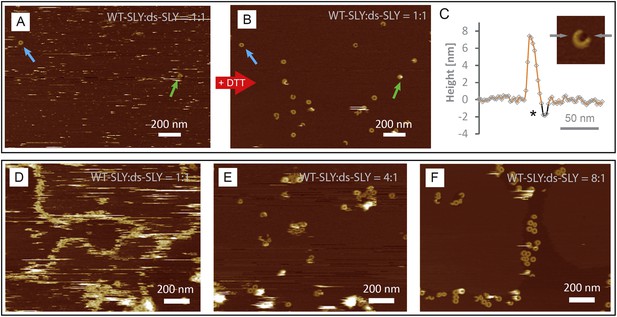
AFM assays of wild-type suilysin (WT-SLY) doped with disulphide-locked suilysin (ds-SLY).
AFM topographic images of WT-SLY doped with an equimolar amount of ds-SLY (A–C) in solution and incubated on egg PC: cholesterol (67:33%) lipid bilayers. (A) The presence of ds-SLY largely traps the WT-SLY in the prepore conformation with only few pores observed (blue and green arrows). Addition of ∼5 mM DTT unlocks the disulphide bond and AFM imaging in the same area (B) reveals more arcs and rings of suilysin in the pore state. (C) Line profile from a suilysin arc after addition of DTT, demonstrating that the membrane is perforated (*). (D–F) AFM images of WT-SLY doped with decreasing amounts of ds-SLY in solution and incubated on DOPC:sphingomyelin:cholesterol, 33:33:33%. (D) At 1:1 ratios of WT-SLY:ds-SLY, the result is similar to (A) with very few suilysin pores observed and prepore locked oligomers prevalent and confined by and at the lipid boundaries. (E) At lower amounts of dopant (WT-SLY:ds-SLY = 4:1), more suilysin pores become visible, with some remaining prepore suilysin oligomers observed as higher arcs and rings and as diffuse streaks. This demonstrates that on reducing the proportion of ds-SLY, the WT-SLY recovers its effectiveness in forming pores in the membrane. (F) With the relative ds-SLY proportion reduced even further (WT-SLY:ds-SLY = 8:1), mostly suilysin pores are prevalent.
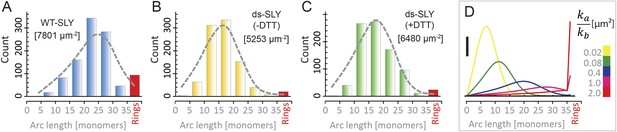
Oligomerization states for arc- and ring-shaped assemblies of suilysin.
(A) The arc-length distribution of wild-type suilysin displays a broad peak for arcs that contain between 15 and 30 monomers, and a smaller, sharp peak for complete rings (37-mers). (B) Arc-length distribution for the disulphide-locked suilysin prepore intermediate. (C) For the disulphide-locked mutant incubated in the presence of DTT (pore-state), the arc-length distribution is practically identical to the distribution for the prepore-locked intermediate. (D) Calculated arc-length distributions for a simple model of kinetically trapped oligomerization, with C = 2000 monomers per square micron (see ‘Materials and methods’). The peak of the arc-length distribution shifts from smaller to larger oligomers on increasing the ratio between the rate constants for monomer association (ka) and monomer binding to the membrane (kb). Vertical scale bar: 40 counts. Grey, dashed lines in A–C denote fits of the experimental data with the oligomerization model, yielding ka/kb = 0.893 ± 0.008 µm2 (A); 0.438 ± 0.012 µm2 (B); 0.425 ± 0.012 µm2 (C). Numbers in brackets in A–C indicate the estimated total number of monomers per square micron. The experimental data here are based on negative-stain EM images on monolayers of egg PC:cholesterol (45:55%), incubated at 37°C.
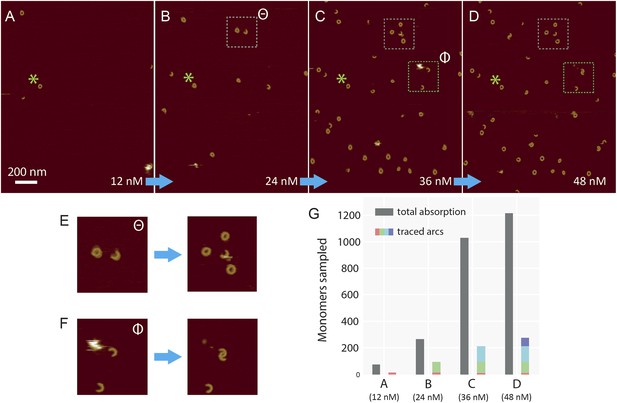
Sequential addition of wild-type suilysin in the pore state.
(A–D) Sequence of AFM images in the same area, showing the effect of sequential injections of wild-type suilysin (WT-SLY) in the solution above the supported lipid bilayer (egg PC:cholesterol, 67:33%) at 27°C. The overall increase in the number of arcs and rings in the pore state can be readily observed while individual arcs in the pore state can be tracked and characterized following each injection. (E) After the third injection, the open-ended arc still persists and the length of the arc does not increase further even as more arcs and rings have assembled in the vicinity of the open-ended arc. (F) After the fourth addition of WT-SLY, the open-ended arcs of SLY are still prevalent and the newly formed arc is interlocked with the arc already present from the previous addition of toxin. (G) The results show that while the total number of monomers in the pore state increases after each WT-SLY addition, the arc length distribution remains unchanged. This implies that after each monomer addition, new arcs (and ring complexes) are formed that do not oligomerize with arcs already in the pore state. Thus suilysin oligomers can only assemble in the prepore state.
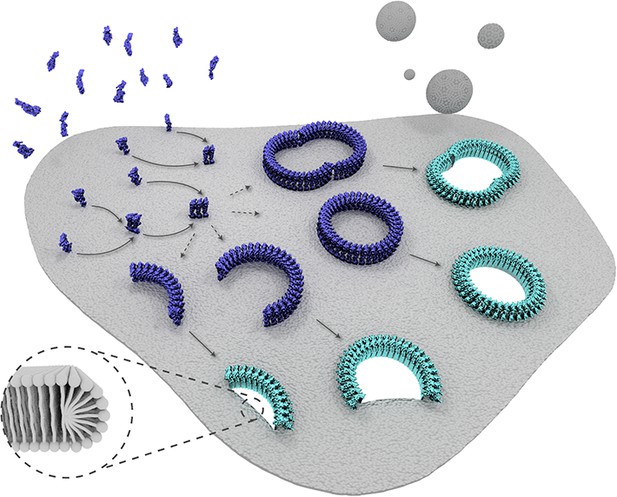
Schematic representation of suilysin membrane binding, assembly, and pore formation.
From left to right: monomers bind to the membrane and oligomerize. The assembly of monomers proceeds in the prepore intermediate and results in either complete rings or kinetically trapped arc-shaped oligomers. The arc- and ring-shaped assemblies subsequently collapse to the pore configuration with the transmembrane β-hairpins unfurled and inserted into the lipid bilayer in a concerted conformational change. Lipids are subsequently ejected from the membrane (shown as grey spheres) and aqueous pores of different sizes are formed in the membrane. The inset shows a possible configuration of lipids at the unsealed edges of the bilayer.
Videos
Mobile disulphide-locked suilysin (prepore) assemblies diffusing on the membrane.
At a temperature of 15°C, the mobility of disulphide-locked suilysin is sufficiently reduced for the assemblies to be resolved by real-time AFM at 15 s/frame. This sequence of images was captured ∼30 min after protein injection and at 384 pixels per line. The timing of the video is accelerated by a factor of ∼100. Full z-colour scale = 20 nm.