The neuronal architecture of the mushroom body provides a logic for associative learning
Figures
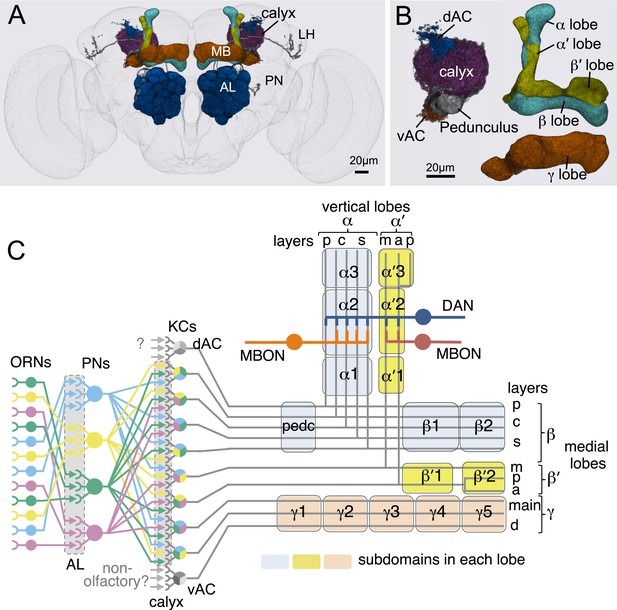
Anatomy of olfactory pathways in the adult fly brain.
(A) An image of the adult female brain showing the antennal lobes (AL) and subregions of the mushroom bodies (MB; see panel B for more detail). The image was generated using a 3D image rendering software (FluoRender) (Wan et al., 2009; Wan et al., 2012). The 51 glomeruli of the AL extend projection neurons (PN) to the calyx of the MB and the lateral horn (LH). There are a total of ∼200 PN; 6 from the DL3 glomerulus are shown. See Video 1 for an introduction to olfactory circuit. (B) Subregions within the MB. The γ lobe, calyx, and pedunculus (ped) are displayed separately from other lobes; their normal positions are as shown in panel A and Video 1. Color-coding is as in panel A. See below for a detailed description of dorsal accessory calyx (dAC) and ventral accessory calyx (vAC). (C) A schematic representation of the key cellular components and information flow during processing of olfactory inputs to the MB (see text for references and more details). Olfactory receptor neurons expressing the same odorant receptor converge onto a single glomerulus in the AL. A small number (generally 3–4) of PNs from each of the 51 AL glomeruli innervate the MB calyx where they synapse on the dendrites of the ∼2000 Kenyon cells (KCs) in a globular structure, the calyx. Each KC exhibits, on average, 6.4 dendritic ‘claws’ (Butcher et al., 2012), and each claw is innervated by a single PN. There is little order in connection patterns of PNs to KCs. The axons of the KCs project in parallel anteriorly through the pedunculus to the lobes, where KCs terminate onto the dendrites of MB output neurons (MBONs). KCs can be categorized into three major classes α/β, α′/β′, and γ based on their projection patterns in the lobes (Crittenden et al., 1998). The β, β′, and γ lobes constitute the medial lobes (also known as horizontal lobes), while the α and α′ lobes constitute the vertical lobes. These lobes are separately wrapped by ensheathing glia (Awasaki et al., 2008). The α/β and α′/β′ neurons bifurcate at the anterior end of the pedunculus and project to both the medial and vertical lobes (Lee et al., 1999). The γ neurons project only to the medial lobe. Dendrites of MBONs and terminals of modulatory dopaminergic neurons (DANs) intersect the longitudinal axis of the KC axon bundle, forming 15 subdomains, five each in the α/β, α′/β′, and γ lobes (numbered α1, α2, and α3 for subdomains in the α lobe from proximal to distal) (Tanaka et al., 2008). Additionally, one MBON and one DAN innervate the core of the distal pedunculus intersecting the α/β KCs (pedc, see below). There are seven types of KCs; five of the seven types have their dendrites in the main calyx, while those of the γd cells form the vAC (Aso et al., 2009; Butcher et al., 2012) and those of the α/βp cells the dAC (Tanaka et al., 2008). The accessory calyces are thought to receive non-olfactory input since they do not receive input from the PNs from the AL (Tanaka et al., 2008). Different KCs occupy distinct layers in the lobes as indicated (p: posterior; c: core; s: surface; m: medial; a: anterior; and d: dorsal). Some MB extrinsic neurons extend processes to only a specific layer within a subdomain, defining elemental subdivisions in the lobes, or ‘synaptic units’ as proposed by Tanaka et al. (2008).
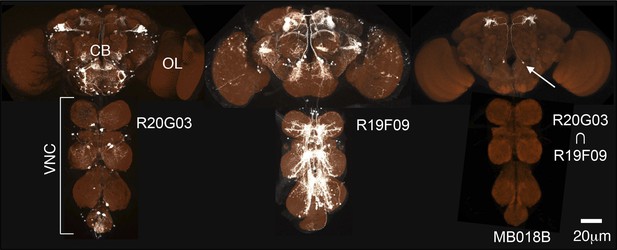
Generation of split-GAL4 drivers for the MB neurons.
An example of the use of the split-GAL4 approach to generate a driver line specific for MBON-α′2 (see Table 1 for the naming convention of MBONs and DANs). R20G03-GAL4 in attP2 (left) and R19F09-GAL4 in attP2 (center) both show expression in MBON-α′2 when crossed to pJFRC2-10XUAS-IVS-mCD8::GFP in attP2 and in many other neurons that differ between the two GAL4 lines. The optic lobes (OL), central brain (CB), and ventral nerve cord (VNC) are indicated. The enhancer fragments from these lines were used to generate the fly line MB018B carrying both R20G03-p65ADZp in attP40 and R19F09-ZpGAL4DBD in attP2 (right). The p65ADZp and ZpGAL4DBD proteins are themselves inactive; the reconstitution of an active GAL4 transcription factor requires heterodimerization that occurs only in cells expressing both proteins (Luan et al., 2006; Pfeiffer et al., 2010). This approach, therefore, labels cells in which both enhancers are active. The arrow indicates the cell body of one MBON-α′2 cell visualized using pJFRC225-5xUAS-IVS-myr::smGFP-FLAG reporter in VK00005 (white). Neuropils were visualized with nc82 antibody (orange). Genotypes of 92 split-GAL4 lines and the cell types they label are listed in Supplementary file 1 and raw confocal images are available online (http://www.janelia.org/split-gal4). The expression pattern observed using a split-GAL4 line depends to some extent on the UAS reporter construct used, as illustrated in Figure 2—figure supplement 1. Expression patterns of split-GAL4 lines for KCs (Figure 2—figure supplement 2), PPL1-cluster DANs (Figure 2—figure supplement 3), PAM cluster DANs (Figure 2—figure supplement 4), and MBONs (Figure 2—figure supplement 5) are shown. We also generated split-GAL4 lines for a variety of other modulatory cell types that project to the MB including serotonergic, GABAergic, octopaminergic, and peptidergic neurons (Figure 2—figure supplement 6). We chose lines with minimal off-target expression in neuronal and non-neuronal cells (Figure 2—figure supplement 7) to facilitate the use of these lines in future functional analyses to manipulate the activity of individual cell types.
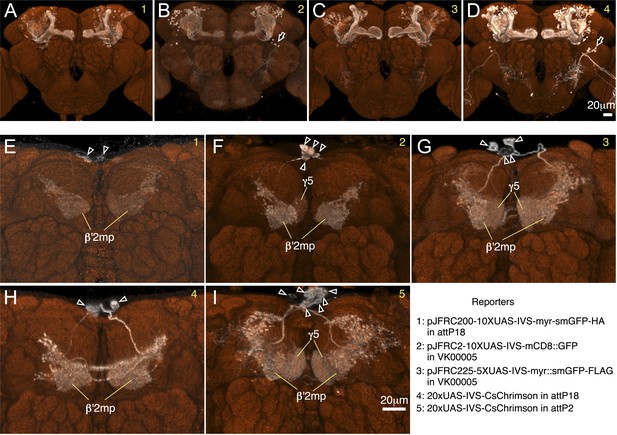
Expression pattern of split-GAL4 drivers with various reporters.
Expression pattern of the MB005B (A–D) or MB002B (E–I) visualized with different UAS-reporters, as indicated by the number in the top left corner of each panel: 1, pJFRC200-10XUAS-IVS-myr::smGFP-HA in attP18; 2, pJFRC2-10XUAS-IVS-mCD8::GFP in VK00005; 3, pJFRC225-5XUAS-myr::smGFP-FLAG in VK00005; 4, 20xUAS-IVS-CsChrimson in attP18; 5, 20xUAS-IVS-CsChrimson in attP2. UAS expression was imaged by confocal microscopy and then rendered using FluoRender (white; see ‘Materials and methods’). For reference, the neuropil was visualized (orange) using an nc82 antibody. Reporters in attP18 tended to show weaker but more restricted labeling compared to those in attP2 or VK00005 (Pfeiffer et al., 2010). (A–D) The α′/β′ Kenyon cells were consistently visualized with the four reporters tested, although intensity of signals differed. Expression pattern was very specific to the α′/β′ Kenyon cells with pJFRC-10XUAS-IVS-myr::smGFP-HA in attP18 (A), whereas other reporters visualized additional expression in off-target cell types (arrows in B and D). (E–I) MB002B consistently visualized the MBON-β′2mp with all five reporters tested. The two reporters in attP18 visualized only MBON-β′2mp (E and H; cell bodies are indicated by arrowheads). However, we detected additional expression in other MBONs (but no other cell types) with reporters in VK00005, which also visualized MBON-γ5β′2a (F and G), and in attP2, which also visualized MBON-γ5β′2a and MBON-β′2mp_bilateral (I). These cell types have similar cell body locations (arrowheads) and axon tracts, consistent with those arising from the same developmental lineage.
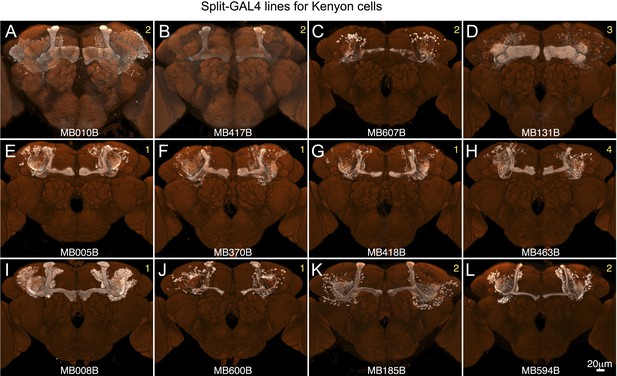
Expression patterns of split-GAL4 drivers for KCs.
(A–L) Expression patterns obtained with a UAS-reporter that showed high-specificity are shown; the number in the top right corner in each panel indicates the reporter (see Figure 2—figure supplement 1). See Supplementary file 1 for the list of cell types in each driver line and the online database (http://www.janelia.org/split-gal4) to view original confocal stacks as well as expression patterns in the VNC and expression observed with other reporters.
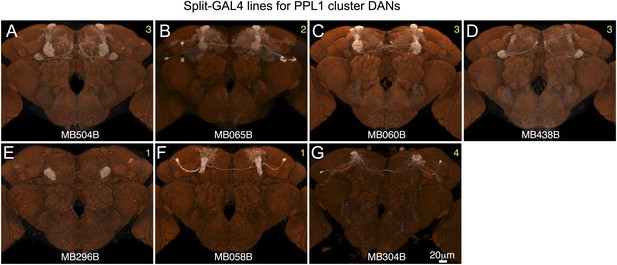
Expression patterns of split-GAL4 drivers for PPL1 cluster DANs.
(A–G) Expression patterns obtained with a UAS-reporter that showed high-specificity is shown; the number in the top right corner in each panel indicates the reporter (see Figure 2—figure supplement 1). See Supplementary file 1 for the list of cell types in each driver line and the online database (http://www.janelia.org/split-gal4) to view original confocal stacks as well as expression patterns in the VNC and expression observed with other reporters.
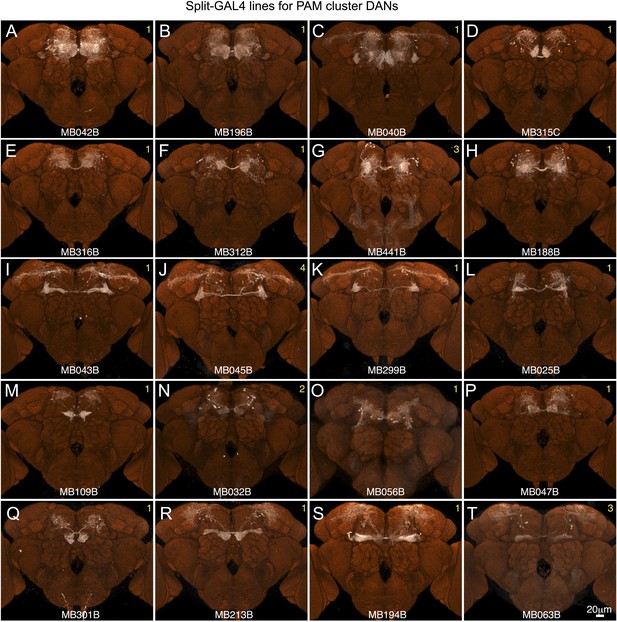
Expression patterns of split-GAL4 drivers for PAM cluster DANs.
(A–T) Expression patterns obtained with a UAS-reporter that showed high-specificity is shown; the number in the top right corner in each panel indicates the reporter (see Figure 2—figure supplement 1). See Supplementary file 1 for the list of cell types in each driver line and the online database (http://www.janelia.org/split-gal4) to view original confocal stacks as well as expression patterns in the VNC and expression observed with other reporters.

Expression patterns of split-GAL4 drivers for MBONs.
(A–X) Expression patterns obtained with a UAS-reporter that showed high-specificity is shown; the number in the top right corner in each panel indicates the reporter (see Figure 2—figure supplement 1). See Supplementary file 1 for the list of cell types in each driver line and the online database (http://www.janelia.org/split-gal4) to view original confocal stacks as well as expression patterns in the VNC and expression observed with other reporters.
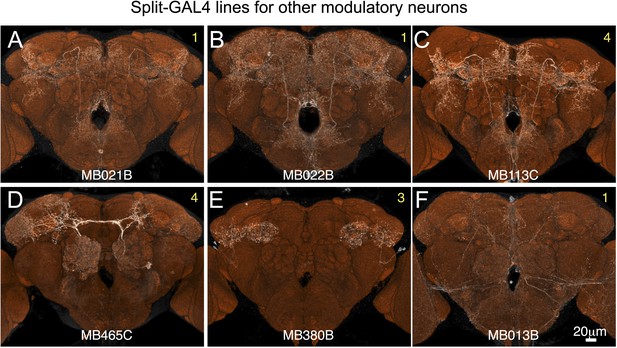
Expression patterns of split-GAL4 drivers for other modulatory input cells.
(A–F) Expression patterns obtained with a UAS-reporter that showed high-specificity is shown; the number in the top right corner in each panel indicates the reporter (see Figure 2—figure supplement 1). See Supplementary file 1 for the list of cell types in each driver line and the online database (http://www.janelia.org/split-gal4) to view original confocal stacks as well as expression patterns in the VNC and expression observed with other reporters.
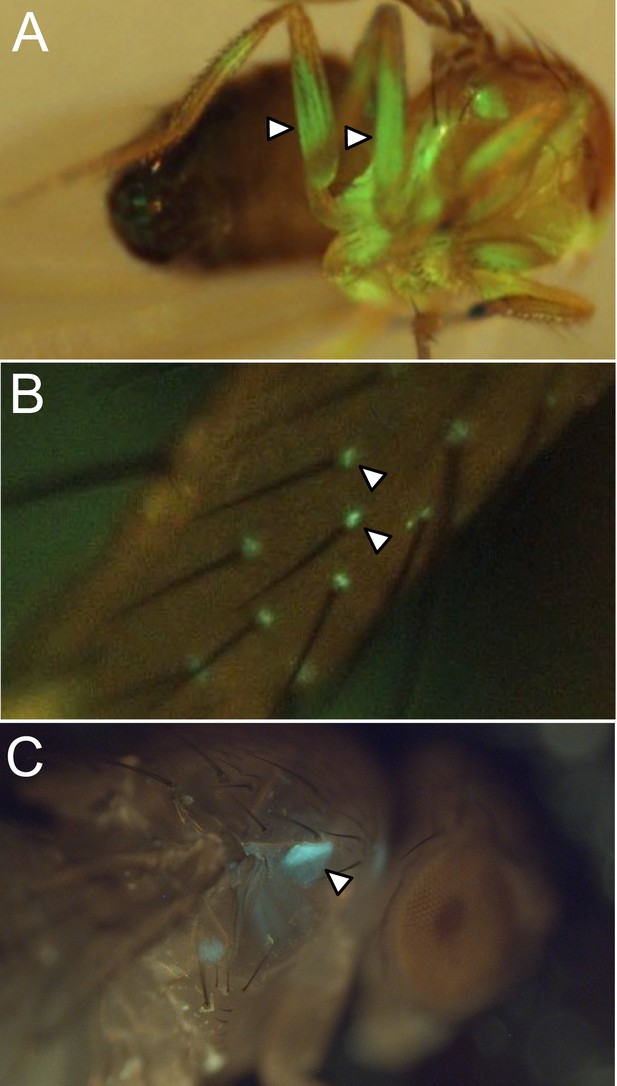
Examples of off-targeted non-neuronal expression.
We screened split-GAL4 lines for expression in tissues outside the central nervous system by imaging the native fluorescence of GFP from the pJFRC2-10xUAS-IVS-mCD8::GFP reporter in VK00005 (Pfeiffer et al., 2010).
Examples of lines that we excluded from our collection for use in behavioral assays because of expression in leg muscles (A), cells in the sensory bristles (arrow heads) on the leg (B), or a prothoracic muscle (C; MB062C) are shown. Such lines are not recommended for use in behavioral experiments but can be utilized for anatomical analyses; for example, MB062C is used in Figure 11D.
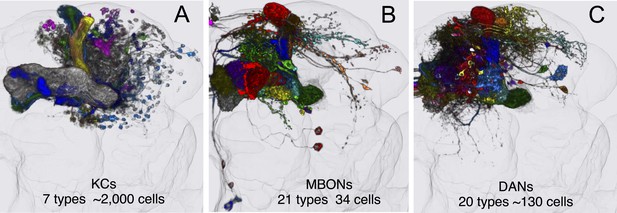
MB cell types.
Registered images of KCs (A; Video 2), MBONs (B; Video 3), and DANs (C; Video 4). After alignment to the standard brain and segmentation, different colors were assigned to each cell type, while the outlines of the neuropils of the brain are shown in light gray. These images illustrate the overall extent and position of these cell types in the brain; the morphology of individual cell types can be seen in Videos 2–4. For MBONs, the two neurons found upon PA-GFP tracing experiments (see below) are not included, thus the image represents 19 different MBON cell types. These are the three major intrinsic and extrinsic neuron types innervating the MB lobes. Additionally, there are two intrinsic neurons (MB-APL and MB-DPM) and 10 extrinsic neuron types that innervate the MB; see Table 1 for references. The extrinsic neurons with processes in the lobes include two types of octopaminergic neurons (OA-VPM3 and OA-VPM4) and one type of peptidergic neuron (SIFamide). The extrinsic neurons with processes in the calyx include two types of octopaminergic neurons (OA-VPM5 and OA-VUMa2), one type of GABAergic neuron (MB-C1), one serotonergic neuron (MB-CSD), two types of DANs (PPL2ab-DANs), and one neuron with dendritic arbors in the calyx and the proximal pedunculus as well as in the LH (MB-CP1). See Figure 3—figure supplement 1 for images of some of these cell types.
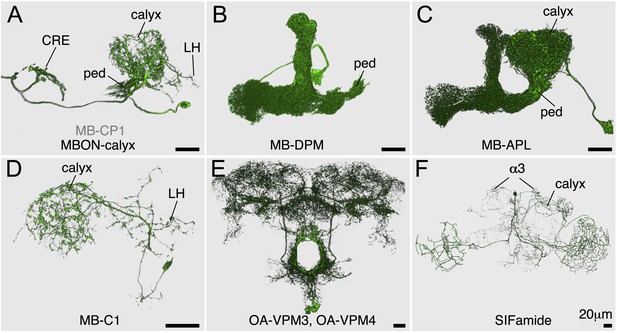
MBON-calyx and other modulatory neurons.
Each cell type was segmented and visualized using FluoRender. Oblique view (A–C) or frontal views (D–F) are shown. (A) A single MB-CP1 in MB622B was visualized with pJFRC225-5XUAS-myr::smGFP-FLAG in VK00005. The dendrites of this neuron are in the calyx, pedunculus, and the lateral horn (LH) and its terminals bilaterally project to the crepine (CRE). The neurotransmitter used by this neuron has not been determined. (B) A single MB-DPM in MB034C was visualized with pJFRC225-5XUAS-myr::smGFP-FLAG in VK00005. (C) A single MB-APL in VT43924 was visualized by multi-color flp-out (MCFO; see ‘Materials and methods’). (D) One of the two MB-C1 neurons in MB380B was visualized by MCFO. They were immunoreactive to GAD1 (data not shown). (E) OA-VPM3 and OA-VPM4 (four cells in total per brain) in MB022B were visualized with pJFRC200-10XUAS-IVS-myr::smGFP-HA in attP18. (F) One of the four SIFamide neurons in MB013B was visualized by MCFO. In addition to the reported innervation of the calyx (Verleyen et al., 2004), we also found terminals in α3.
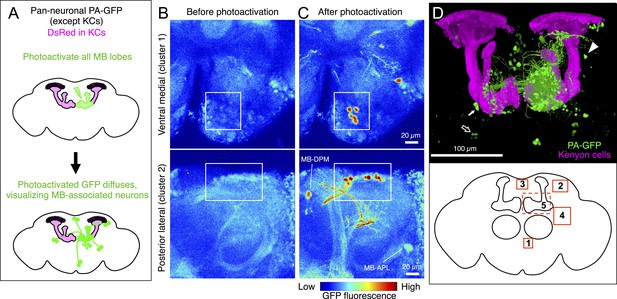
Identification of neurons innervating the MB lobes by PA-GFP tracing.
(A) Schematic representation of the experiment. PA-GFP was expressed pan-neuronally, using a synaptobrevin-GAL4 driver, except in the KCs where GAL4 activity was suppressed by MB247-GAL80. Photoactivation of the MB lobes was guided by a red fluorescent protein expressed broadly in the KCs (MB247-DsRed). A two-photon laser was used to achieve three-dimensional precision of the photoactivation. Photoactivation results in an increase in the fluorescence of PA-GFP molecules in the processes of neurons innervating the MB lobes. The photoactivated GFP molecules then diffuse throughout the cytoplasm of these neurons, revealing both cell bodies and processes (see ‘Materials and methods’ for details and limitations). (B) Baseline fluorescence of PA-GFP before photoactivation in the ventral medial (top; cluster 1 in panel D) or posterior lateral region of the brain (bottom; cluster 2 in panel D). Each panel represents a maximum intensity projection of z-stack images obtained with two-photon microscopy. (C) Fluorescence of PA-GFP after photoactivation of the right MB lobes in the same brain regions as in (B). The cell bodies as well as the processes of photoactivated neurons are labeled strongly with PA-GFP. The cell bodies of MB-DPM and MB-APL neurons were also visualized. (D) MB extrinsic neurons as identified by PA-GFP tracing upon photoactivation of the right MB lobes. Shown here is a three-dimensional reconstruction of a confocal stack. KCs are labeled in magenta (MB247-DsRed) and the cell bodies and the processes of photoactivated neurons are shown in green (native PA-GFP fluorescence). Five clusters of cell bodies comprising more than two cells were detected reproducibly (see ‘Materials and methods’) and are schematically indicated by the boxes (bottom): 1, ventral medial cluster; 2, posterior lateral cluster; 3, dorsal medial cluster; 4, anterior lateral cluster; and 5, delineated by the dashed lines, the anterior medial cluster that includes the PAM-cluster DANs. PA-GFP labeling in the contralateral (i.e., left) hemisphere includes the cell bodies of DANs (the PAM cluster DANs in the anterior medial region and the PPL1 cluster DANs in the posterior lateral region), as they innervate the MB lobes bilaterally. Eight individual neurons were also detected, which include MB-APL and MB-DPM in the ipsilateral hemisphere, and one MBON-β1>α, one MBON-γ4>γ1γ2 (closed arrow), one MBON-γ3, and one MBON-γ3β′1 (open arrow) in the contralateral hemisphere, because these extend dendrites in the contralateral MB lobes (see below). In addition, one cell body ventral to the calyx (arrowhead) and one cell body ventral lateral to the antennal lobe were reproducibly observed, which represent MBONs not identified in the split-GAL4 lines (see Figure 5G and Figure 5—figure supplement 1, also see ‘Materials and methods’). The comparison of number of cells identified in split-GAL4 lines and in the PA-GFP tracing experiments is as follows: cluster name; cell counts by split-GAL4 lines, cell counts by PA-GFP tracing (sample size): (1) Ventral medial; 5, 5.2 ± 0.8 (n = 5). (2) Posterior lateral; 14–15, 15.9 ± 2.1 (n = 9). (3) Dorsal medial; 4, 5.4 ± 1.2 (n = 13). (4) Anterior lateral; 8–10, 5.9 ± 2.0 (n = 8). Note that the split-GAL4 line labeling the MBON-β′1 type, which comprises of 7–9 cells in the anterior lateral cluster (MB078C, see Table 1) includes at least three cells that exhibit very sparse dendritic arbors in the MB lobes as examined by multicolor flp-out experiments (data not shown). This was also observed in an independent experiment, in which the β′1 compartment was specifically photoactivated (Figure 5B) and may account for the fact that PA-GFP experiments identified fewer cells than the split-GAL4 lines in the anterior lateral cluster. It was not feasible to accurately count the large number of cells in the anterior medial cluster (cluster 5; indicated as a dashed box) comprising mostly the PAM DANs and several MBONs such as MBON-α1 (see Figure 5 and ‘Materials and methods’). Genotype of the fly was yw/yw; UAS-C3PA-GFP(attP40),MB247-DsRed/UAS-SPA-GFP(attP40),MB247-GAL80; NSyb-GAL4/UAS-C3PA-GFP(attP2),UAS-C3PA-GFP(VK00005),UAS-C3PA-GFP(VK00027).
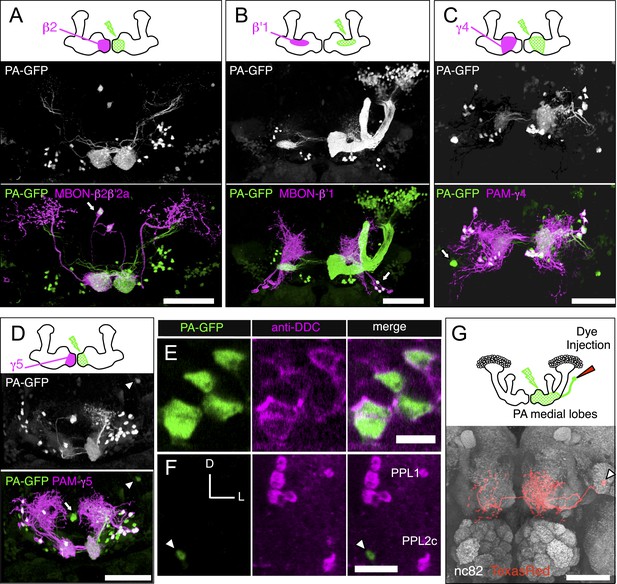
Identification of the MB extrinsic neurons innervating each MB lobe compartment by PA-GFP tracing.
We photoactivated each of the 15 MB lobe subdomains (Figure 1C), or compartments (see below), individually to provide an independent approach to identify the extrinsic neurons associated with each compartment. PA-GFP was expressed pan-neuronally using a synaptobrevin-QF driver (see ‘Materials and methods’) but was suppressed in α/β and γ KCs using MB247-QS. Note that the α′/β′ KCs were visualized when compartments in the α′/β′ lobes were photoactivated (e.g., panel B). Different split-GAL4 lines (e.g., MBON lines for panels A and B, and DAN lines for panels C and D) were used to drive expression of membrane-targeted red fluorescent protein (myr::tdTomato) to demarcate a specific compartment within the lobes for photoactivation. The photoactivated samples were subsequently immunostained for a marker of dopaminergic cells (tyrosine hydroxylase [not shown] or dopa-decarboxylase [DDC, see panels E and F]) to classify individual photoactivated neurons as MBONs or DANs (see ‘Materials and methods’). (A–D) Each panel shows a diagram of the experiment and a three-dimensional reconstruction of confocal images showing DANs and MBONs innervating the photoactivated compartment. Photoactivation was performed unilaterally (i.e., right) as indicated in the diagram. Native PA-GFP fluorescence is shown alone in gray scale in the middle panel and in green in the bottom panel together with myr::tdTomato signals driven by the indicated split-GAL4 in magenta; overlap is white. We identified all of the MBONs found in the split-GAL4 lines by photoactivation of each compartment (arrows and data not shown). We also identified DANs innervating each compartment (for example, myr::tdTomato negative and PA-GFP positive cells in panels A and B). We noted additional DANs in the PAM cluster that are not labeled by the split-GAL4 lines (green cell bodies in panels C and D). We therefore counted PAM cluster DANs associated with each medial lobe compartment based on these PA-GFP experiments (see ‘Materials and methods’). The comparison of numbers of DANs in the PAM cluster identified in split-GAL4 lines and in the PA-GFP tracing experiments (i.e., photoactivated cells positive for tyrosine hydroxylase or dopa-decarboxylase) is as follows: compartment name; cell counts by split-GAL4 lines, cell counts by PA-GFP tracing (sample size): γ1; 13 (as mixtures of PAM-γ4 and PAM-γ4>γ1γ2 in MB312B), 3.8 ± 1.3 (n = 5). γ2; 13 (as mixtures of PAM-γ4 and PAM-γ4>γ1γ2 in MB312B), 7.8 ± 2.2 (n = 6). γ3; 9 (MB441B), 23.6 ± 6.3 (n = 5). γ4; 13 (as mixtures of PAM-γ4 and PAM-γ4>γ1γ2 in MB312B), 17.3 ± 1.3 (n = 6). γ5; 8 (MB315C), 21.5 ± 1.3 (n = 6). β′1; 14 (as mixtures of PAM-β′1ap and PAM-β′1m in MB025B), 12.8 ± 1.3 (n = 4). β′2; 35 (sum of PAM-β′2a in MB109B, PAM-β′2m in MB032B, PAM-β′2p in MB056B, and PAM-β2β′2a in NP5272), 41.3 ± 6.6 (n = 4). β1; 5 (sum of PAM-β1 in MB063B and at least one PAM-β1 in MB194B), 6.6 ± 1.1 (n = 5). β2; 11 (sum of PAM-β2β′2a in NP5272 and PAM-β2 in MB209B), 18.8 ± 4.3 (n = 5). Thus, by simply summing the number of cells associated with these compartments, PA-GFP experiments identified ∼154 DANs in the anterior medial cluster (i.e., PAM cluster) as compared to 121 cells labeled by the split-GAL4 lines. Note these numbers include PAM DANs innervating multiple compartments (PAM-γ4>γ1γ2 and PAM-β2β′2a) and are therefore an overestimate. The split-GAL4 collection identified 98 DANs of 14 types in the PAM cluster, whereas anti-dopamine immunostaining identified 115–135 DANs in the PAM cluster (Liu et al., 2012). (E) An example of immunostaining for dopa-decarboxylase (DDC) upon photoactivation of a single compartment. The panels represent a single confocal section of the PAM cluster upon photoactivation of the γ5 compartment. (F) The γ4γ5 extrinsic neuron identified by the PA-GFP tracing and not in the split-GAL4 screen shown in D (arrowhead) is DDC-negative and thus is likely an MBON. Images are maximum intensity projections. Dorsal is to the top and lateral is to the right. The cell body of this γ4γ5 MBON is positioned between the PPL1 and PPL2c DAN clusters. (G) Morphology of the γ4γ5 MBON identified by PA-GFP experiments with subsequent dye injection. Diagram of the experiment (top): Texas-Red dye was iontophoresed into the cell body identified by photoactivation (see ‘Materials and methods’). A three-dimensional reconstruction of a confocal stack with neuropil labeled by nc82 immunostaining (gray) and the γ4γ5 MBON labeled by anti-Texas-Red immunostaining (red) is shown (bottom). The arrowhead indicates the cell body that is located close to the posterior surface of the brain ventral to the MB calyx. This neuron as well as an additional MBON with its cell body ventral lateral to the antennal lobe observed in PA-GFP experiments was found in a VT-GAL4 line (see Figure 5—figure supplement 1 for single cell morphologies). Genotypes used: (A–F) yw/yw; MB247-QS,QUAS-C3PA-GFP,QUAS-SPA-GFP/QUAS-C3PA-GFP,QUAS-SPA-GFP,UAS-myr::tdTomato(attP40); NSyb-QF,UAS-myr::tdTomato(attP2)/SplitGAL4DBD(attP2),SplitGAL4AD(VK00027). Split-GAL4 lines used: (A) MB399C; (B) MB078C; (C) MB312C; and (D–F) MB315C. Genotype of the animals in panel (G): yw/yw; MB247-GAL80,UAS-C3PA-GFP/UAS-SPA-GFP; NSyb-GAL4/UAS-C3PA-GFP (attP2),UAS-C3PA-GFP (VK00005),UAS-C3PA-GFP (VK00027). Scale bars are 50 μm in (A–D) and (G), 5 μm in (E), and 20 μm (F).
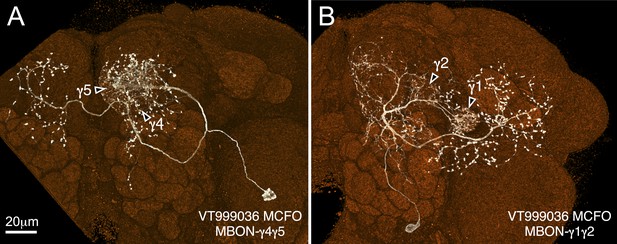
Two MBONs not identified in the split-GAL4 screen.
Single cell morphologies were obtained by MCFO of VT999036, segmented and visualized by FluoRender (white, neurons: orange, nc82). Interestingly, VT999036 carries an enhancer fragment from the Tyramine β hydroxylase gene, a synthetic enzyme for octopamine, raising the possibility that these MBONs might be octopaminergic. Because we only discovered these cell types near the end of this study, and we did not have available split-GAL4 lines for them, they were not included in our subsequent analyses. (A) MBON-γ4γ5 (also shown in Figure 5G). Similar to MBON-calyx, the cell bodies of MBON-γ4γ5 neurons are located in the posterior protocerebrum and their primary neurites run through the pedunculus. Their dendrites arborize in γ4 and γ5 and axon terminals are largely confined in the CRE. (B) MBON-γ1γ2. The cell bodies of MBON-γ1γ2 are located ventral to the antennal lobe. Their dendrites sparsely arborize in γ1 and γ2 as well as in the CRE and SMP. They project to the inferior and superior clamp, neuropils surrounding the pedunculus, and the anterior part of the superior lateral protocerebrum (SLP), a region where other MBONs do not project.
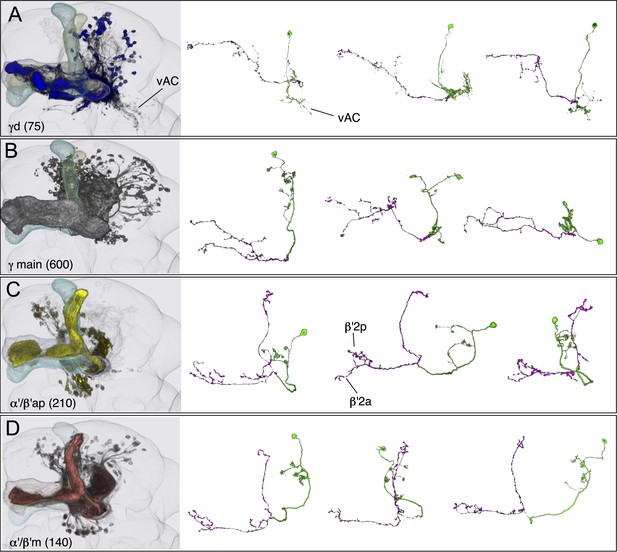
KCs of the γ and α′/β′ lobes.
Seven KC types identified by split-GAL4. Representative aligned images of each KC type (see also Figure 7) are shown; the name of the cell type and the approximate number of cells per brain hemisphere are indicated. Three examples of single cell morphologies, segmented from multicolor flip-out experiments, are presented for each cell type illustrating the branching pattern of the KC axons as they project through the lobes. In the single cell images, the cell body, primary neurite, dendrites, and axon in the pedunculus have been false-colored green and axons in the lobes are magenta. Based on co-expression in specific split-GAL4 lines and single cell morphologies, we have divided the KC population into 7 cell types. The number of cells of each type was estimated by counting labeled nuclei in split-GAL4 lines (see ‘Materials and methods’) and is shown in parentheses. (A) The γd KCs are thought to be of embryonic origin, because they are not included in a clonal analysis that visualized all post-embryonic KCs (Lin et al., 2007; Yu et al., 2013), and they have morphological similarity to the embryonic born KCs of basal cockroaches (Farris and Strausfeld, 2003). Their dendritic arbors form a protrusion extending ventral lateral to the main calyx, which we named the ventral accessory calyx (vAC). Their axons occupy the most peripheral layer in the pedunculus, the ventral and anterior layers in the γ1–γ4 compartments, and the dorsal layer in γ5. Single cell morphologies (from MB028B and MB355B) reveal that the γd axons have more branches in γ3–γ5 than in γ1 and γ2. The vAC may be devoted to non-olfactory inputs; the major types of olfactory projection neurons from the antennal lobe do not innervate this structure (Butcher et al., 2012). (B) The γ main KCs have their dendrites in the main calyx and their axons occupy about 75% of the volume of the γ lobes. Single cell morphologies (from MB369B and MB355B) reveal that each γ main KC branches in all the five compartments of the γ lobe. (C) The α′/β′ap KCs have dendrites in the main calyx and project axons to the anterior and posterior layers of the α′/β′ lobes. Single cell morphologies (from MB461B and MB463B) reveal that axonal branches from single KCs project to both β′2a and β′2p, where they overlap with distinct sets of output and dopaminergic neurons (see below). (D) The α′/β′m KCs have dendrites in the main calyx. In β′2, their axons are located in the area between the bifurcating axons of α′/β′ap neurons shown in panel C. In the α′ lobe, their axons are medial to those of the α′/β′ap cells. Single cell images are from MB418B and MB369B.
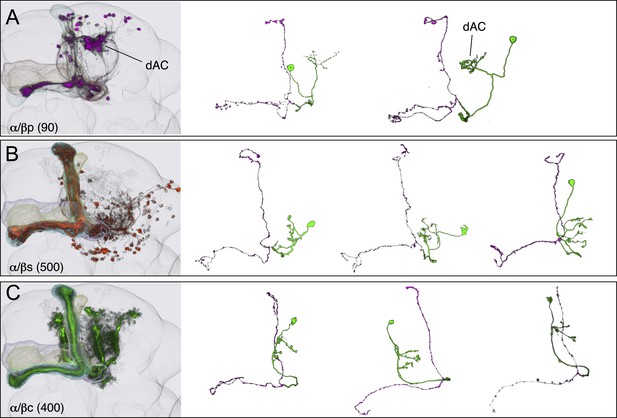
KCs of the α/β lobes.
(A) The dendrites of the α/β posterior (α/βp) KCs form a protrusion extending to the dorsal lateral side of the main calyx. This structure has been called the accessory calyx, but we have renamed it as the dorsal accessory calyx (dAC) to distinguish it from the ventral AC (vAC) (Figures 1C and 6A). The α/βp KC axons project to the posterior layer of the α/β lobe. These are the firstborn α/β KCs and are also known as pioneer α/β KCs (Lin et al., 2007). The single cell images were segmented from multicolor flp-out (MCFO) brains of MB469B and MB371B. (B) The α/β surface (α/βs) KCs have dendrites in the main calyx and project axons to the surface layer of the α/β lobes where they form a continuous layer surrounding the α/β core KCs shown in (C). Single cell morphologies of cells (from MB185B) reveal that the α/βs KCs have relatively smooth axonal projections in the lobes. (C) The α/β core (α/βc) KCs have dendrites in the main calyx. They are the last born KCs and their axons occupy the core of the pedunculus and the α/β lobes. They can be morphologically subdivided into inner and outer core cells (Tanaka et al., 2008), although the border between the inner and outer core is not well defined and we were unable to make a split-GAL4 driver line that labels only the outer core cells. Single cell morphologies (from MB594B) reveal that the axons of the α/βc cells have the fewest branches of the 7 types of KCs.
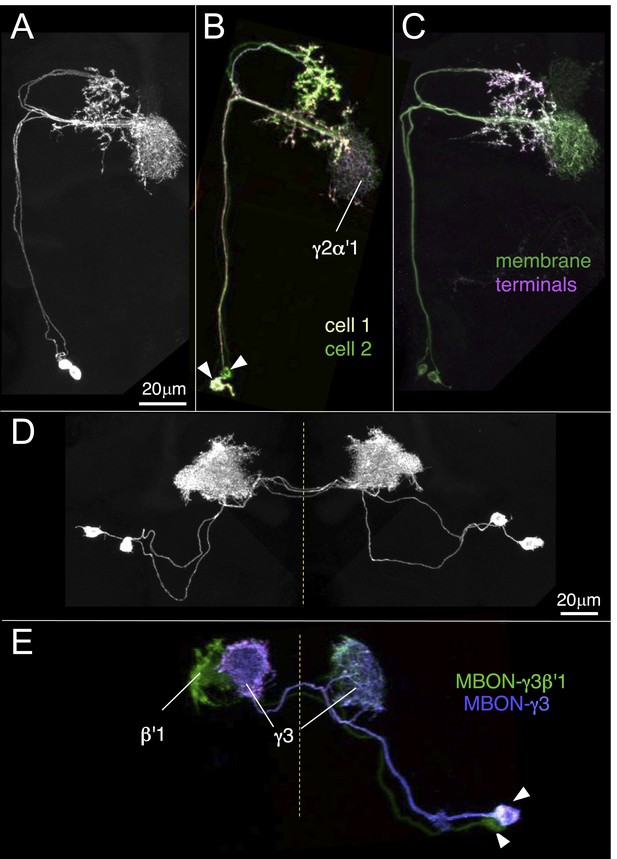
Identification of MBONs and visualization of their single cell morphologies.
Each MBON is named according to the compartment(s) in the MB lobes where its dendrites arborize (see Table 1 and below). For example, MBON-γ2α′1 neurons exhibit dendritic arbors in γ2 and α′1 compartments. (A) The projection patterns of MBON-γ2α′1 neurons. Maximum intensity projection confocal images of MB077B driven expression in one brain hemisphere are shown. Visualization with pJFRC225-5XUAS-IVS-myr::smGFP-FLAG in VK00005 labels two MBON-γ2α′1 neurons per hemisphere. (B) Labeling of the two MBON-γ2α′1 neurons in different colors using multicolor flp-out (MCFO; Nern et al., in preparation; see ‘Materials and methods’). The arbors of the two neurons overlap and are indistinguishable, thus these two cells represent a single cell type. Arrowheads indicate cell bodies. (C) MB077B driven expression of a membrane targeted epitope (green; pJFRC225-5XUAS-IVS-myr::smGFP-FLAG in VK00005) and a presynaptically targeted epitope (magenta; pJFRC51-3XUAS-IVS-Syt::smGFP-HA in su(Hw)attP1). The fine processes in the MB lobes are typical of dendrites, whereas the processes of this neuron that are outside the lobes end with varicosities containing the presynaptic marker. (D) The morphologies of MBON-γ3 and MBON-γ3β′1 as identified by MB083C driven expression. Using the pJFRC225 membrane-targeted reporter, two neurons innervating the γ3 and β′1 MB compartments in each hemisphere can be seen. Dashed vertical line shows the position of the mid-line. (E) Using MCFO, the two cells in one hemisphere were labeled in different colors. Both cells have dendrites in γ3 in both hemispheres but axonal terminals in just the contralateral brain hemisphere. However, one of them (the green cell) also has dendrites in the contralateral β′1 compartment, demonstrating that these two cells represent different cell types, which we named MBON-γ3 and MBON-γ3β′1.
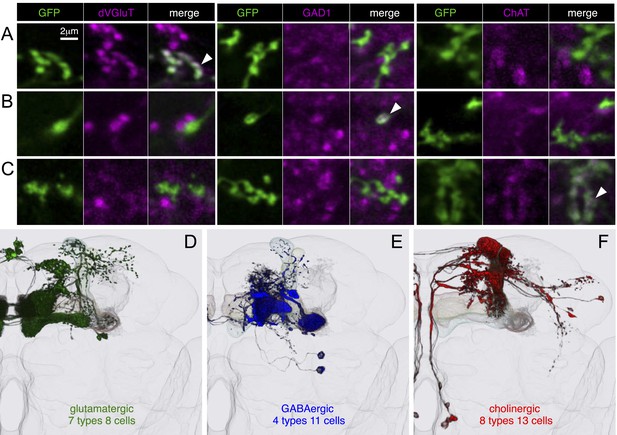
Neurotransmitters used by MBONs.
The putative neurotransmitters used by MBON cell types were assigned by assessing the immunoreactivity of their axon terminals to antibodies raised against Drosophila vesicular glutamate transporter (dVGluT), Drosophila glutamate decarboxylase 1 (GAD1), and Drosophila choline acetyl transferase (ChAT) (see ‘Materials and methods’). Single confocal optical sections; axon terminals of different MBONs are shown in green and antibody staining in magenta. (A) Axon terminals of MBON-γ5β′2a (MB210B) were labeled with anti-dVGluT (arrowhead) but not with either anti-GAD1 or anti-ChAT, suggesting that this cell type is glutamatergic. (B) Axon terminals of MBON-γ1pedc>α/β (MB112C) were labeled with anti-GAD1 (arrowhead), suggesting that this cell type is GABAergic. (C) Axon terminals of MBON-γ2a′1 (MB077B) were labeled with anti-ChAT (arrowhead), suggesting this cell type is cholinergic. (D–F) MBONs of the same neurotransmitter type were given the same color and are displayed together. (D) Seven types of glutamatergic MBONs. (E) Four types of GABAergic MBONs. (F) Eight types of cholinergic MBONs. The neurotransmitter of the two MBON types found in the VT-GAL4 collection was not determined (but see Figure 5—figure supplement 1). Video 5 illustrates the relative positions of these neurons in the standard brain.
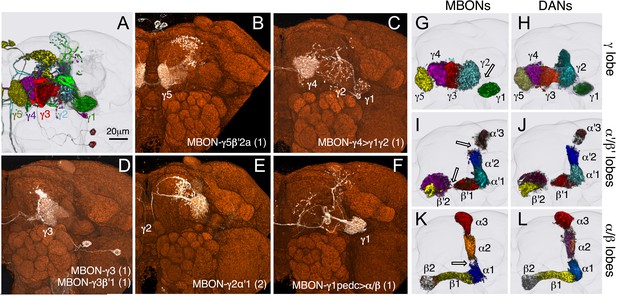
Compartmentalization of the MB lobes.
(A–F) Tiling of MBON dendrites in the γ lobe. (A) A registered image of a brain hemisphere showing 5 MBON cell types that innervate contiguous compartments of the γ lobe. (B–F) Confocal images of brains showing expression in the MBONs shown in A; pJFRC225-5xUAS-IVS-myr::smGFP-FLAG in VK00005 and the following split-GAL4 lines were used to generate the images: B, MB210B; C, MB298B; D, MB083C; E, MB077B; and F, MB112C. The cell types are indicated in the panels with the number of neurons of each type in parenthesis; the neurons are shown in white and the nc82 reference stain in orange. (G–L) The dendrites of MBONs and the axon terminals of DANs tile the MB lobes, defining 15 compartments. Dendrites of MBONs (G, I, and K) and axon terminals of DANs (H, J, and L), aligned to the standard brain, are shown for each lobe. The same false colors were assigned to the DANs and the MBONs of the same compartment. The arrows in (G, I, and K) show the four compartment borders where gaps were routinely seen between the MBON dendrites in adjacent compartments; these gaps correspond to areas of reduced synaptic density (see Figure 10—figure supplement 1). Note that the anterior layer of the β′2 compartment contains dendrites of MBON-γ5β′2a (I, yellow) and axon terminals of PAM-β2β′2a (J, yellow).
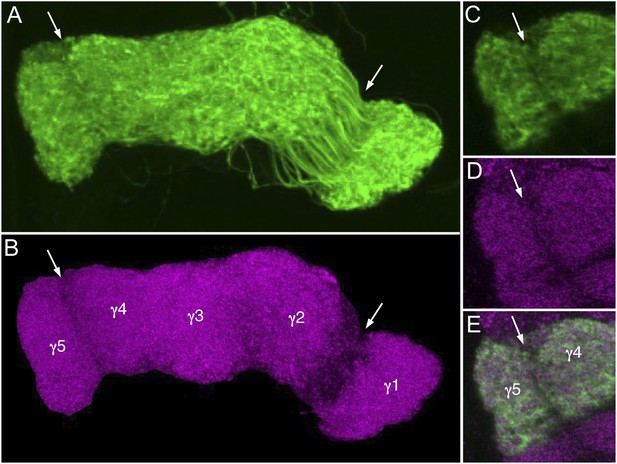
Lower density of presynaptic sites at the border between compartments.
Comparison of the KC membrane and presynaptic labeling in the γ lobe. (A) Membrane-labeled γd and γmain KCs (MB131B, pJFRC225-5xUAS-IVS-myr::smGFP-FLAG in VK00005); a substack projection of the γ lobe is shown. (B) Presynaptic sites within the γ lobe (nc82; magenta); nc82 staining outside the γ lobe has been eliminated for clarity. Arrows indicate borders between compartments of the γ lobe where synaptic density is low. (C–E) Single confocal slice at the border between the γ5 and γ4 subdomains showing KCs (C), nc82 staining (D), and a merged image (E).
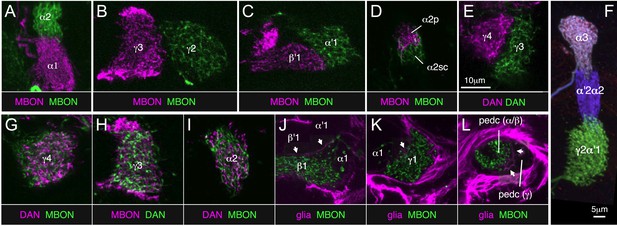
Two-color labeling experiments demonstrating compartmentalization of the MB lobes.
(A–E) Two-color labeling of MBONs (A–D) or DANs (E) from adjacent compartments, or in the case of (D), subdivisions of the same compartment. Neurons were visualized by split-GAL4 and LexA drivers in combination with pJFRC200-10XUAS-IVS-myr::smGFP-HA in attP18 and pJFRC216-13XLexAop2-IVS-myr::smGFP-V5 in su(Hw)attP8, respectively. Substack projections of the compartments are shown. Clear segregation was observed between dendrites of MBONs or axon terminals of DANs from neighboring compartments. (F) MCFO labeling of a single brain showing the termini in the MB lobes of three types of PPL1 cluster DANs. The image was generated from line MB060B, which expresses in four types of PPL1 neurons. We were able to confirm all 11 compartment borders tested using either two-color labeling or MCFO experiments; we did not have the required genetic lines to test one of the 12 compartment borders (between β1 and β2). (G–I) Two-color labeling of DANs and MBONs from the same compartment. Single confocal slices are shown. The DANs and MBONs coextend, with each densely arborizing in the entire compartment. (J–L) Two-color labeling of ensheathing glia and MBONs. Whereas each of the three lobes (i.e., γ, α′/β′, and α/β) is separated clearly by ensheathing glia, we did not observe glia between MBON compartments within each lobe. Single confocal slices are shown. Arrows indicate the ensheathing glia separating the axon bundles of the γ, α′/β′, and α/β neurons in the lobes and pedunculus. The driver lines used are as follows: (A) MB310C (magenta), R34B02-LexA (green); (B) MB083C (magenta), R25D01-LexA (green); (C) MB083C (magenta), R25D01-LexA (green); (D) MB062C (magenta), R34B02-LexA (green); (E) MB316C (magenta), R48B03-LexA (green); (G) MB312C (magenta), R53C03-LexA (green); (H) MB083C (magenta), R48B03-LexA (green); (I) MB058B (magenta), R34B02-LexA (green); (J) MB434B (green), R16D08-LexA (magenta); (K) MB112C (green), R16D08-LexA (magenta); (L) MB112C (green), R16D08-LexA (magenta). Scale bar in (E) applies to all panels except (F).
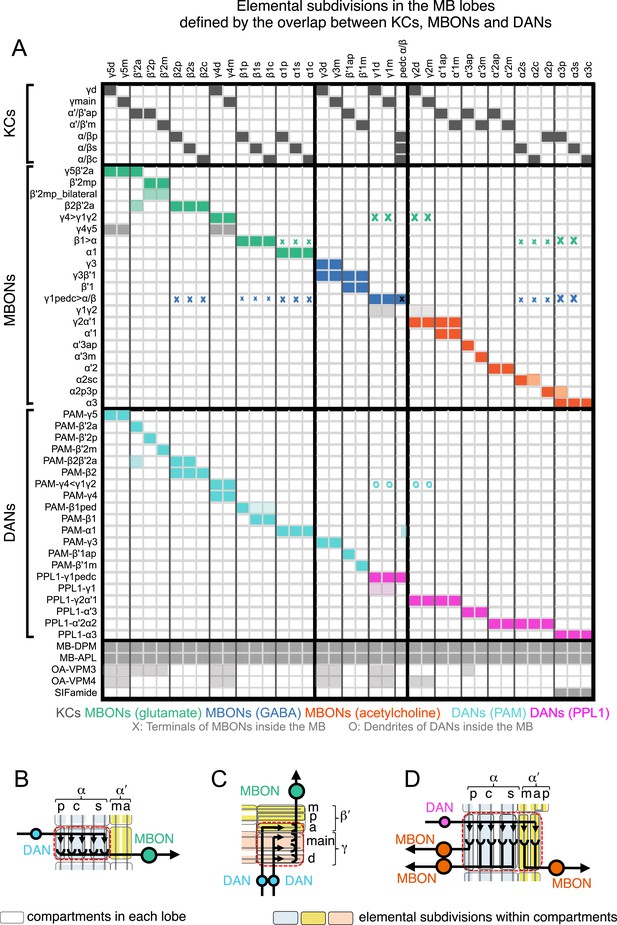
The arborization patterns of individual cell types within the MB lobes.
(A) A matrix summarizing the projection patterns in the MB lobes of the KC axons, the MBONs, the DANs, and other modulatory neurons. The 15 lobe compartments and the core of distal pedunculus (pedc) are separated with thick vertical lines, and each compartment is further divided into columns representing ‘synaptic units’ (Tanaka et al., 2008), elemental subdivisions that are the smallest regions in the MB lobes where a specific set of MBONs, DANs, and KC types overlap. For example, the β1 compartment can be divided into posterior (β1p), surface (β1s), and core (β1c) subdivisions containing the axons of the α/βp, α/βs, and α/βc KCs, respectively. The processes of some MBONs and DANs do not arborize in an entire compartment, but only in a subset of its elemental subdivisions. Tanaka et al. (2008) first described this anatomical feature and called such regions ‘synaptic units’. An example is shown in Figure 11D, which shows a two-color labeling experiment illustrating the subdivision of the α2 compartment by the dendrites of the two MBONs, one of which arborizes in the domain of the posterior KCs (α2p) and the other in the domain of the surface and core KCs (α2sc); this arrangement is diagrammed in Panel D. The heavy vertical lines divide subdivisions into three groups representing different MBON neurotransmitter types (also indicated by the colors filling the cells of the matrix). The rows of the matrix correspond to the cell types of KCs, MBONs, and DANs. See Table 1 for synonyms for cell type names and references. The colors of the cells of the matrix indicate the putative transmitter for MBONs and cluster of origin for DANs. The color correspondence is given below the matrix. The two MBON types of unknown neurotransmitter type are indicated in light gray. The fully-colored cells of the matrix represent processes of neurons (dendrites for MBONs and synaptic terminals for DANs) that are uniformly and densely distributed in that subdivision; fainter colors represent subdivisions that are innervated only sparsely. In three cases, output neurons send axon terminals back into the MB. Such cases are represented by an ‘X’ with the size representing the density of terminals in that subdivision. One DAN cell type (PAM-γ4<γ1γ2) has dendrites within (as well as outside) the lobes in the subdivisions indicated by an ‘O’ (see Figure 14—figure supplement 1E). The projection patterns of the GABAergic MB-APL and serotonergic MB-DPM are diagramed; these neurons are MB intrinsic neurons that broadly innervate the MB (Figure 3—figure supplement 1B,C) (Waddell et al., 2000; Tanaka et al., 2008). Also diagrammed are the projection patterns of the octopaminergic OA-VPM3 and OA-VPM4 (Figure 3—figure supplement 1E) (Busch et al., 2009; Busch and Tanimoto, 2010) and SIFamide peptidergic neurons (Figure 3—figure supplement 1F) (Verleyen et al., 2004) within the lobes; these neurons have only a small fraction of their terminals within the MB (Figure 2—figure supplement 6) and these sparsely innervate only a subset of the compartments. The following abbreviations are used in the names of the subdivisions: a, anterior; m, middle; p, posterior; d, dorsal; s, surface; c, core; and pedc, pedunculus core. (B–D) Diagrams illustrating different circuit motifs found in the MB lobes. (B) The terminals of a single DAN type (PAM-α1) and the dendrites of a single MBON type (MBON-α1) occupy a compartment. 16 of the 21 MBON types, like MBON-α1, arborize dendrites within just one of the three lobes (i.e., γ, α′/β′, and α/β), indicating that these MBONs receive inputs from only one of the three KC classes. (C) Two DANs (PAM-γ5 and PAM-β′2a) innervate the region occupied by one MBON (MBON-γ5β′2a). One DAN fills the γ5 compartment and the other only innervates the anterior elemental subdivision of the β′2 compartment (β′2a); a single MBON has inputs from the areas defined by both DANs. Four MBON types, like MBON-γ5β′2a, extend dendrites spanning lobe boundaries. (D) A single DAN (PPL1-α′2α2) innervate two compartments (the α′2 and α2), an area covered by three MBON types (MBON-α′2, -α2sc, and -α2p3p). Eight MBON types, like MBON-α2sc and MBON-α2p3p, arborize dendrites further confined to elemental subdivision(s) within a compartment. This suggests that these MBONs receive input exclusively from subtypes of KCs; for example, MBON-α2p3p receives input from α/βp KCs, which presumably carry non-olfactory information (see Figure 7A). Nearly all DANs have their termini confined to a single compartment; however, we identified 3 DAN types that have axon terminals in two compartments.
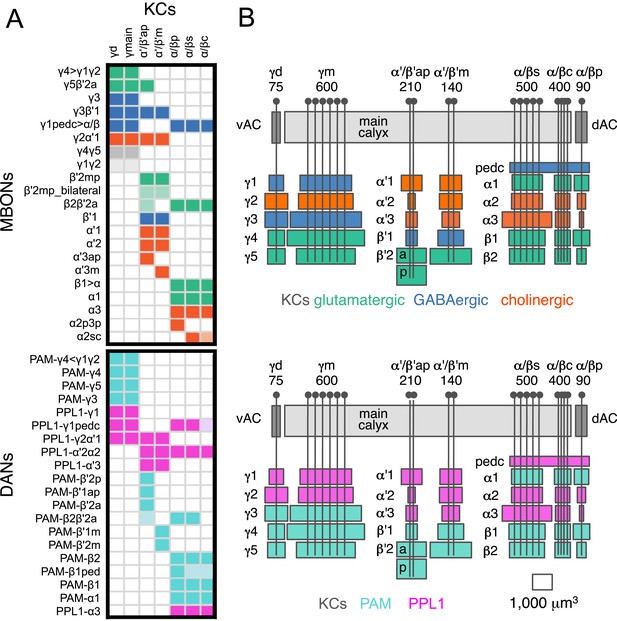
Each KC type transmits information to multiple compartments.
(A) A simplified version of the matrix shown in Figure 12A that illustrates the contacts between the individual MBON (top) and DAN cell types (bottom) with the seven types of KCs (columns). Colors of the cells in the matrix indicate neurotransmitter type for MBONs and cluster of origin for DANs. The two MBON types of unknown neurotransmitter type are indicated in light gray. Interestingly, each KC likely synapses with MBONs of all three neurotransmitter types and receives modulatory DAN input from both PAM and PPL1 clusters. (B) Diagrams of the MB lobes. The axons of the seven types of KCs are shown as straight vertical lines without branches and the boxes represent each of the 37 elemental subdivisions defined in Figure 12A. The number of KCs of each type, based on cell counting (see ‘Materials and methods’), is indicated. The size of the box representing each subdivision and calyces indicates its volume, as determined by measurements performed on confocal stacks. The volumes of lobes are not simply proportional to the number of KCs they contain and are not uniform along their lengths, presumably reflecting differences in synaptic density. The convergence ratio from KCs to single MBONs could range from as high as ∼2300:1 (for MBON-β2β′2a that arborizes in β2 and β′2a of both hemispheres) to ∼90:1 (for MBON-α2p3p), assuming that each KC forms synapses in each elemental subdivision. In the top diagram, the color of the box represents the neurotransmitter used by the MBONs that have dendrites in that subdivision. In the bottom diagram, the color of the boxes represents the cluster of origin of the DANs innervating that subdivision. The following abbreviations are used in the names of the elemental subdivisions: a, anterior; m, middle; p, posterior; d, dorsal; s, surface; c, core; and pedc, pedunculus core.
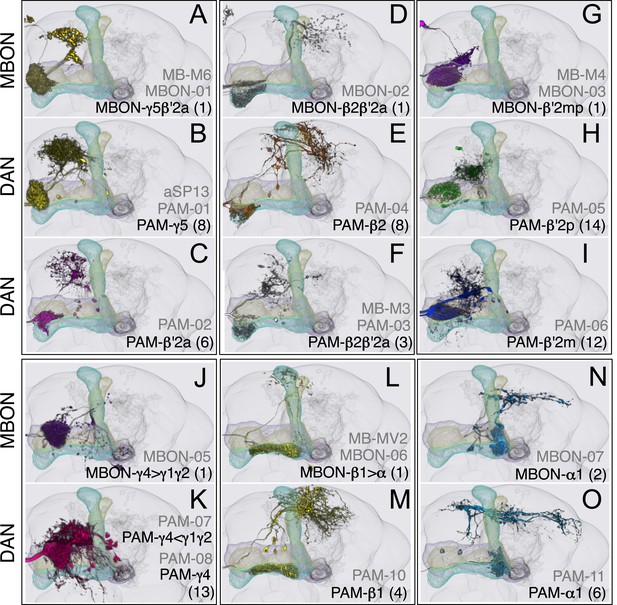
Compartments with dendrites of glutamatergic MBONs.
Representative images of neurons that have been aligned to the standard brain are shown. Glutamatergic MBONs and DANs that project to the same compartments in the lobes are displayed together. The names of cell types are given in a standard format that includes information about the subdivisions innervated. Short names based on simple numbering, as well the original name in cases where the cell type has been previously described at the single cell level, are also shown (gray font). The number of cells per cell type found in each brain hemisphere is shown in parentheses. Some cell types were not separated by split intersections and images show mixtures of cell types in these cases. Except for PAM-γ4<γ1γ2 and PPL1-γ1, DANs have dendritic branches in the ipsilateral hemisphere and axons that bilaterally innervate the same MB compartments in both hemispheres. The distribution of neurites outside the MB lobes is shown in more detail in Figure 18—figure supplement 1. The split-GAL4 drivers for each cell type are listed in Table 1 and Supplementary File 1. (A) The dendrites of MBON-γ5β′2a arborize in the contralateral γ5 and β′2a. The major axon of MBON-γ5β′2a projects ipsilaterally to the superior medial protocerebrum (SMP), whereas a very thin axon projects to the CRE and SMP in the other hemisphere (see Figure 14—figure supplement 1A). (B) PAM-γ5 neurons. Dendrites of these neuron arborize in the same regions of SMP where the MBON-γ5β′2a neurons terminate, suggesting a possible recurrent loop (see text and Figure 20C). (C) PAM-β′2a neurons. (D) The dendrites of MBON-β2β′2a bilaterally arborize in the β2 compartment and β′2a subdivision and its axon projects ipsilaterally to the superior intermediate protocerebrum (SIP) and superior lateral protocerebrum (SLP) (see also Figure 14—figure supplement 1B). (E) PAM-β2 neurons. (F) PAM-β2β′2a neurons have sparse terminals in the anterior layer of β′2a and even sparser terminals in the core layer of β2. The dendrites of PAM-β2β′2a and PAM-β2 are spatially segregated, suggesting that distinct upstream neurons regulate their activity. (G) MBON-β′2mp arborizes in the contralateral β′2mp; its main axon projects to the CRE and SMP on the same side and its minor axon to the ipsilateral side (Figure 14—figure supplement 1C). A second output neuron, MBON-β′2mp-bilateral, sparsely arborizes its dendrites in the β′2mp compartment and projects dense axons bilaterally (see Figure 14—figure supplement 1D). (H) PAM-β′2p neurons. (I) PAM-β′2m neurons. (J) The MBON-γ4>γ1γ2 dendrites arborize in the contralateral γ4 and its axon projects both within the lobes to γ1 and γ2 and to regions outside the lobes. (K) PAM-γ4 neurons and PAM-γ4<γ1γ2 neurons. The image shows a mixture of two cell types; PAM-γ4<γ1γ2 is unusual, in which it has some of its dendrites within the MB (in the γ1 and γ2 compartments; see Figure 14—figure supplement 1E). (L) The MBON-β1>α dendrites arborize in the contralateral β1 and its axon innervates the α1, α2, and α3 compartments within the lobes as well as areas outside the lobes (see Figure 14—figure supplement 1F); the terminals in α3 are concentrated in the surface and posterior layers. (M) PAM-β1 neurons (bottom). The posterior layer of β1 is more densely innervated by a second PAM cluster cell type, PAM-β1ped, that also projects to the posterior end of the pedunculus (see Figure 14—figure supplement 1G). (N) The dendrites of MBON-α1 arborize in α1 and its axons project to the posterior SIP and SLP. Two neurons with identical morphology are present in each hemisphere. Although we observed this cell type in MCFO analysis of the dVGlut-GAL4 line OK371 (Mahr and Aberle, 2006), its terminals showed much weaker immunoreactivity to the anti-dVGluT than the other putative glutamatergic neurons. (O) PAM-α1 neurons (bottom) have terminals that extend slightly outside the area arborized by the dendrites of MBON-α1 to the distal end of the pedunculus.
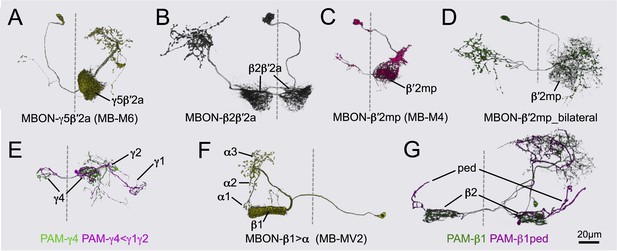
Neurons of the glutamatergic compartments.
Morphologies of individual cells were determined by MCFO using the indicated split-GAL4 lines. Brain midlines are indicated by dashed lines. (A) MBON-γ5β′2a (MB210B). (B) MBON-β2β′2a (MB014B). One of the only three MBON cell types, with MBON-γ3 and MBON-γ3β′1, to have bilateral dendritic branches in the MB. (C) MBON-β′2mp (MB011B). (D) MBON-β′2mp bilateral (MB210B). This neuron has sparse dendritic arbors in the β′2mp. Unlike MBON-β′2mp, this cell type sends dense axonal projections to both hemispheres. This cell type was only found in split-GAL4 lines (for example, MB011B and MB210B) together with other MBONs. (E) PAM-γ4 and PAM-γ4<γ1γ2 (MB312B). Processes of PAM-γ4<γ1γ2 neurons in the γ1 and γ2 are devoid of Syt::smGFP-HA signals and on this basis are considered to be dendritic (data not shown). (F) MBON-β1>α (MB434B). (G) PAM-β1 and PAM-β1ped neurons (MB194B). The PAM-β1ped neurons project through the pedunculus to the anterior edge of the calyx. Thus, unlike PPL1-γ1pedc that broadly innervates the pedc, this neuron appears to extend through the pedc to the remainder of the pedunculus without extensive arborization.
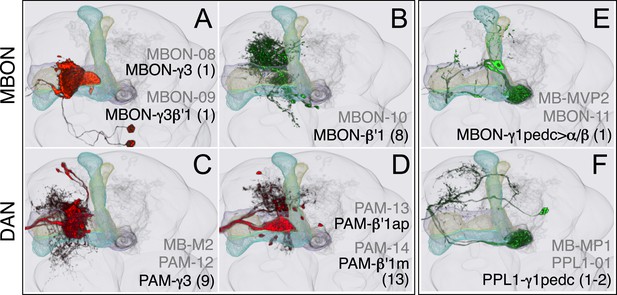
Compartments with dendrites of GABAergic MBONs.
(A) MBON-γ3 and MBON-γ3β′1; the morphologies of these cell types are described in more detail in Figure 8D,E. (B) MBON-β′1 (top right); this MBON type is unusual in a number of ways. First, there are, on average, 8 cells per hemisphere (i.e., the number of cells fluctuate between 7–9 in MB057B) compared with one or two for the other MBON cell types. Second, they are the only MBONs from the lobes whose dendrites arborize, in addition to the lobes, in neighboring neuropils (CRE and SMP). Since CRE and SMP contain zones where the terminals of other MBONs converge (see below), these neurons may sum the outputs from a number of MB compartments. Finally, this is the only MBON cell type that projects to the lateral accessory lobe, an output region of the central complex. (C–D) Three types of PAM cluster DANs innervate the γ3 and β′1 compartments: PAM-γ3 neurons (C); PAM-β′1ap neurons; and PAM-β′1m neurons (D). (E) The dendrites of MBON-γ1pedc>α/β (top) arborize in the ipsilateral γ1 and the core of the pedunculus, where the axons of the α/β KCs are found. Its axon projects bilaterally to the α/β lobes and contralaterally to the core of the pedunculus and, to a lesser extent, outside the MB lobes; in α3, its terminals are enriched in the surface layer (similar to those of MBON-β1>α, see Figure 17E). (F) PPL1-γ1pedc; a DAN of the PPL1 cluster with terminals that overlap with the dendrites of MBON-γ1pedc>α/β. One additional PPL1 cluster DAN innervates γ1 sparsely (PPL1-γ1; not shown, see Table 1).
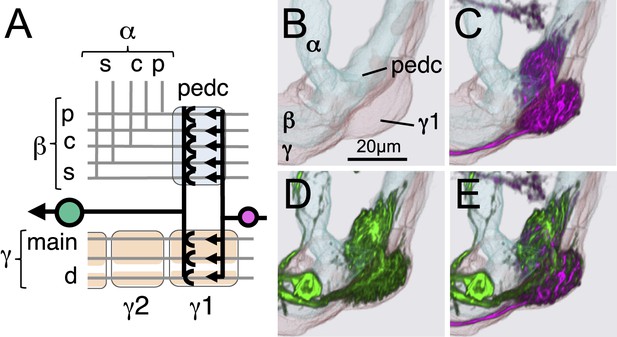
The MBON-γ1pedc>α/β and PPL1-γ1pedc innervates the γ1 lobe compartment as well as the core of the distal pedunculus.
(A) Diagram of innervation by dendrites of MBON-γ1pedc>α/β and axon terminals of PPL1-γ1pedc, which intersect the axon bundle of the two types of γ neurons (γd and γmain) in the γ1 compartment and the three types of α/β neurons (α/βs, α/βp, and α/βs) at the core of the pedunculus. Registered images of the outline of the γ lobe and the α/β lobes (B), PPL1-γ1pedc (C), MBON-γ1pedc>α/β (D), and both neurons (E) are shown from the side together with outline of the γ lobe and the α/β lobes. Ensheathing glia separate the γ1 compartment and the core of the pedunculus; the neurites of MBON-γ1pedc>α/β and PPL1-γ1pedc bifurcate and pass through the glia ensheathing each lobe (see Figure 11K–L).
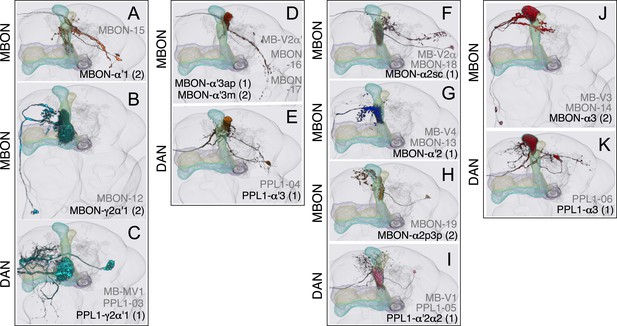
Compartments with dendrites of cholinergic MBONs.
(A) The dendrites of the two MBON-α′1 cells arborize unilaterally in α′1. Their thin dendritic branches project through the edge of the α′2 and α′3 compartments. The MBON-α′1 neurons share an axon tract with MBON-α′3ap and MBON-α′3m and terminate in similar region of SIP, SLP, and LH (see panel D). (B) MBON-γ2α′1. This cell type consists of two morphologically identical cells (see Figure 8B) that project to the CRE and SMP. (C) PPL1-γ2α′1 neuron. (D) The dendrites of MBON-α′3ap and MBON-α′3m arborize in the ipsilateral α′3m or α′3ap, respectively, and their axons project bilaterally to the same regions of the LH, SIP, and SLP. The axonal branches of the MBON-α′3m stop at the LH, whereas the MBON-α3′ap axons extend to a region ventral to the LH. (E) PPL1-α′3 neuron. (F) The dendrites of MBON-α2sc arborize unilaterally in α2sc, and its axon projects bilaterally to the SIP, the SLP, and the dorsal LH. (G) MBON-α′2 projects to the CRE and SMP. A few terminals are also found in SIP. (H) The two MBON-α2p3p cells arborize their dendrites unilaterally in the posterior layer of the α2 and α3 compartments (α2p and α3p) and project to the SMP. The KCs in the posterior layer of the lobes (the α/βp neurons) have their dendrites in the dorsal accessory calyx, a region spatially segregated from the dendritic arbors of other KCs (see Figure 7A), and their activity is decreased in response to odors (Perisse et al., 2013), suggesting a distinct role for these output neurons. (I) PPL1-α′2α2 neuron. (J) Two morphologically identical MBON-α3 neurons have dendrites in α3 and terminals in the SMP, SIP, and SLP. (K) PPL1-α3 neuron.
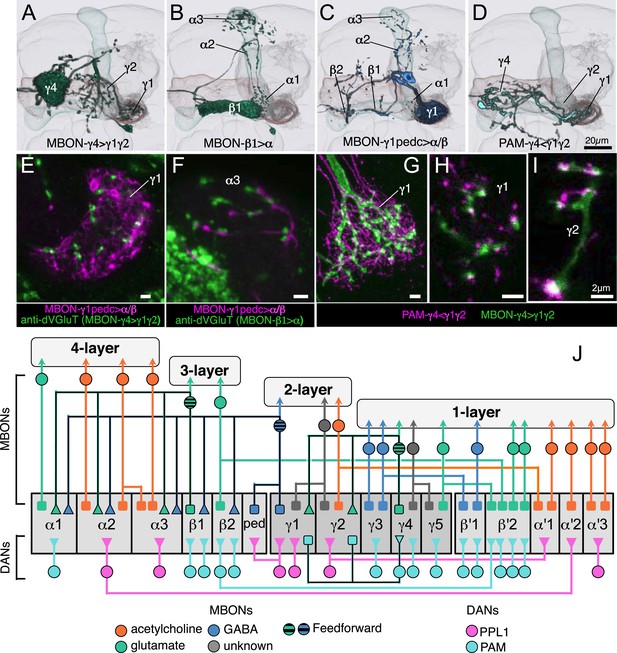
Three types of MBONs and one type of DAN interconnect multiple compartments.
(A–C) Registered images of the three MBON cell types whose axons project to other compartments within the MB lobes. (A) A glutamatergic output (MBON-γ4>γ1γ2) from γ4 sends axons back into the lobes that terminate in γ1 and γ2. (B) A glutamatergic output from β1 (MBON-β1>α) terminates in α1, α2, and α3. (C) A GABAergic output from γ1 and the core of distal pedunculus (MBON-γ1pedc>α/β) terminates in the α/β lobes in both hemispheres and in the pedunculus, where α/β KCs bifurcate, in the contralateral side of the brain. (D) A DAN cell type (PAM-γ4<γ1γ2) that has dendrites in γ1 and γ2 and terminals in γ4. This cell type provides potential feedback signals to the MBON-γ4>γ1γ2 (see panels G–I). (E) Two-color labeling of the GABAergic output neuron shown in panel C (MB112C, pJFRC225-5XUAS-IVS-myr::smGFP-FLAG in VK00005) and an antibody against vesicular glutamate transporter. The dendrites of MBON-γ1pedc>α/β overlap with glutamatergic terminals in γ1. The likely source of these glutamatergic terminals is MBON-γ4>γ1γ2 shown in panel A. (F) The GABAergic terminals of MBON-γ1pedc>α/β and glutamatergic terminals presumably of MBON-β1>α, the neuron shown in panel B, are in close proximity in the surface layer of α3. The green signal in the lower left corner of the panel is from neurons outside the MB. This overlap of glutamatergic and GABAergic feedforward projections suggests that they may cooperatively or competitively regulate the excitatory output of MBON-α3. (G) A substack projection showing two-color labeling of the dendrites of the only DAN (MB312C) that has dendrites in the MB lobes and axon terminals of an MBON in the γ1 compartment (R53C03-LexA). (H and I) Single confocal slices in the γ1 and γ2 compartment showing two-color labeling of the cell types shown in (G). (J) Schematic of the circuits within the MB lobes. DAN inputs (bottom) and MBON outputs (top) from 15 MB lobe compartments and the core of the distal pedunculus (ped) are shown (gray rectangles, middle). Colors indicate neurotransmitter types for MBONs and cluster of origin for DANs as indicated. Dendrites are represented as squares and presynaptic terminals as triangles. Three MBONs, indicated by the heavier outline and striped cell bodies, send axonal terminals (triangles) back into the MB lobes creating a 4-layer feedforward network. See text for details. Eight types of MBONs receive input from more than one of the 15 compartments in the lobes (or a compartment plus the ped) and in five cases those compartments reside in different lobes. As different functions of learning and memory, such as acquisition and retrieval, have been attributed to different KC classes (Isabel et al., 2004; Krashes et al., 2007), MBONs integrating across lobes may function in coordinating different phases of learning and memory. MBON-γ1pedc>α/β and PPL1-γ1pedc innervate the core of the distal pedunculus (ped) as well as the γ1 compartment. Three DAN cell types innervate multiple lobe compartments.
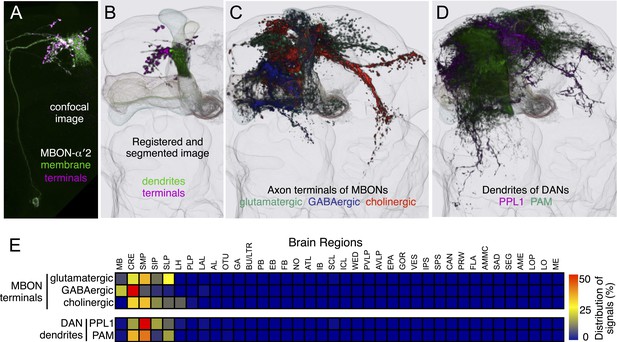
Projection patterns of the MBON axons and DAN dendrites outside the MB lobes.
(A) To analyze the projection patterns of the MBONs outside the MB lobes, we first segmented their axon terminals based on the localization of a presynaptic marker. A maximum intensity projection of a confocal stack showing MBON-α′2 neurons labeled with a presynaptic reporter (magenta) and a general membrane marker (green). The split-GAL4 line MB018B was used to drive the expression of two constructs: a reporter targeted to membranes (pJFRC225-5XUAS-IVS-myr::smGFP-FLAG in VK00005) and a reporter targeted to presynapses (pJFRC51-3XUAS-IVS-Syt::smGFP-HA in su(Hw)attP1). (B) An image of the segmented terminals and dendrites of a MBON-α′2 neuron aligned to the standard brain. After registration of the image, synaptic terminals were segmented based on the preferential labeling by Syt::smGFP-HA. Dendrites were segmented based on their morphology and localization within the MB lobe. (C) A registered image of the segmented presynaptic terminals of MBONs showing the areas of the brain innervated by MBONs. The segmented terminals were false-colored based on their putative neurotransmitter. (D) A registered image of the segmented dendrites of DANs. The segmented dendrites were false-colored based on their cluster of origin. (E) Distribution of MBON terminals and DAN dendrites as quantified in each neuropil found in the adult brain (see Ito et al., 2014 for the location and abbreviation for each neuropil [Ito et al., 2014]). After normalizing the signal intensity from different cell types, we separately summed signals from the terminals of glutamatergic, GABAergic, or cholinergic MBONs and dendrites of PPL1 and PAM cluster DANs. 99% of the MBON terminals are distributed in the MB lobes and five neuropils (CRE, SMP, SIP, SLP, and LH). Colors represent the percent of the processes found in each of 38 brain regions. See ‘Materials and methods’ for a description of the quantification method.
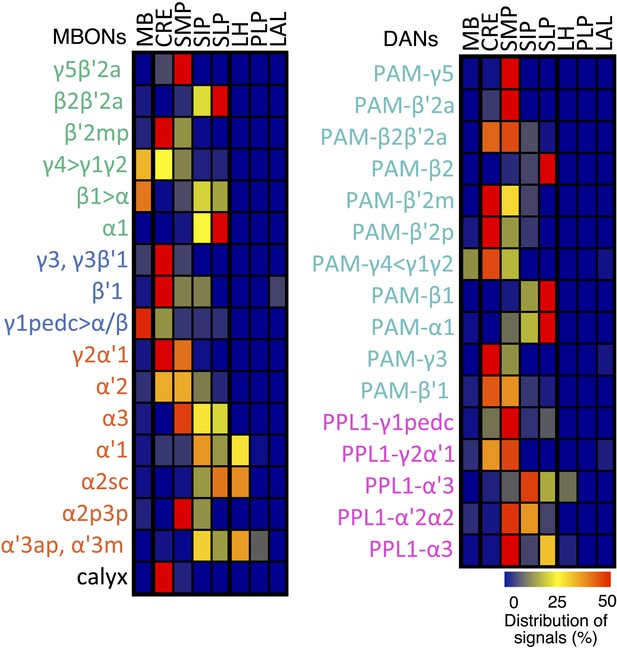
Projection patterns of the individual MBON and DAN cell types.
Distribution of MBON terminals (left panel) and DAN dendrites (right panel) of selected cell types is shown separately. Colors represent the percent of the processes found in each brain region. Note that each MBON type typically projects to multiple neuropils and each DAN type typically has dendrites in multiple neuropils. Note that the atypical neuron with dendrites in the calyx (MB-CP1, see Table 1) is also shown at the bottom row (labeled ‘calyx’).
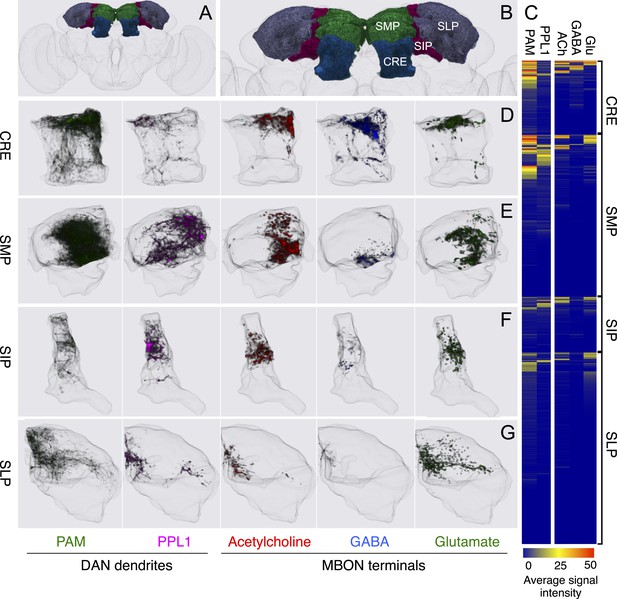
Distribution of MBON terminals and DAN dendrites in four brain areas.
(A) Schematic of the brain highlighting the areas that exhibit the highest density of MBON terminals and DAN dendrites. (B) A magnified view of a portion of the brain shown in panel A with the CRE, SMP, SIP, and SLP neuropils indicated. (C) Distribution of MBON terminals and DAN dendrites showing their further clustering within the CRE, SMP, SIP, and SLP. Heat map representations of neurite density were computed separately for PAM and PPL1 cluster DAN dendrites and for cholinergic (ACh), GABAergic, and glutamatergic (Glu) MBON terminals. Each horizontal row shows mean intensities in a 10 × 10 × 10 voxel (3.8 × 3.8 × 3.8 μm) cube for each of the indicated neuronal types (columns), represented in an 8-bit scale (0–255). The rows have been sorted based on the sum of the intensity in each volume for all neuronal types. (D–G) Distributions of MBON terminals and DAN dendrites in the CRE (D), SMP (E), SIP (F), and SLP (G) are shown.
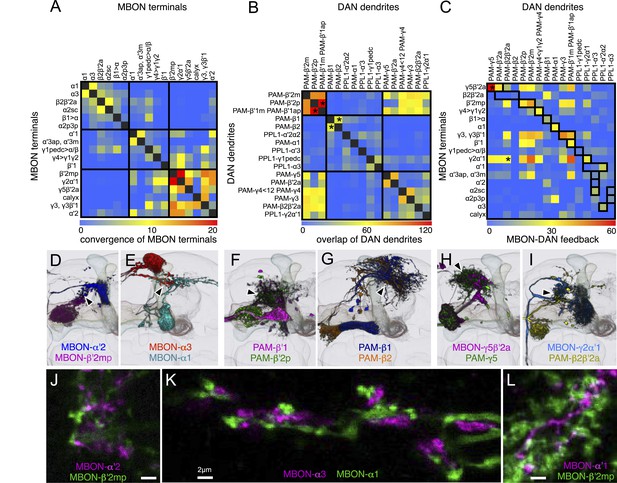
Convergence of MBON and DAN processes outside the MB lobes.
(A–C) Matrices showing co-localization of the processes of MB extrinsic neurons outside the MB. Axon terminals and dendrites from each cell type were first segmented and the degree of overlap calculated (see ‘Materials and methods’) and displayed in the form of a linear heat map with arbitrary units. For those cells in the matrices marked with an asterisk, images of the overlapping neurons are shown in (D–I). The heavy black lines in (A) and (B) show the three major groups as determined by hierarchical clustering (Ward's method). (A) Overlap of the MBON terminals. (B) Overlap of DAN dendrites. (C) Overlap of the MBON terminals and DAN dendrites. The cells of the matrix outlined by the heavy black lines indicate potential feedback loops where MBON terminals from a compartment lie in close proximity to the dendrites of DANs that innervate that same compartment. Note that the atypical neuron with dendrites in the calyx (MB-CP1, see Table 1) is also shown in panels A and C (labeled ‘calyx’). (D and E) Convergence (arrowhead) of the terminals of MBONs from α′2 and β′2mp (D) and of the terminals of MBONs from α3 and α1 (E). The overlap of the axon terminals (also shown in two-color labeling experiments, panels J and K) suggests that different MBONs may target the same post-synaptic neurons. It is interesting that MBONs of different neurotransmitter types exhibit overlapping axon terminals (e.g., cholinergic MBON-α3 and glutamatergic MBON-α1, E), suggesting that the target neuron may be modulated differently by each of the MBONs; for example, receiving excitatory input from cholinergic MBONs and inhibitory inputs from glutamatergic MBONs. (F) Overlap (arrowhead) of dendrites of PAM cluster DANs that innervate β′1 and the β′2p. (G) Overlap (arrowhead) of dendrites of PAM cluster DANs that innervate β1 and β2. (H) Overlap (arrowhead) of the terminals of the MBON-γ5β′2 and dendrites of PAM-γ5. (I) Overlap (arrowhead) of the terminals of the MBON-γ2α′1 and dendrites of PAM-β2β′2a. (J–L) Single confocal slices showing two-color labeling of the axonal terminals of the indicated MBONs. The following split-GAL4 and LexA driver lines were used: (J) MB081C and R25D01-LexA; (K) G0239 (Chiang et al., 2011) and R71C03-LexA; (L) MB026B and R12C11-LexA.
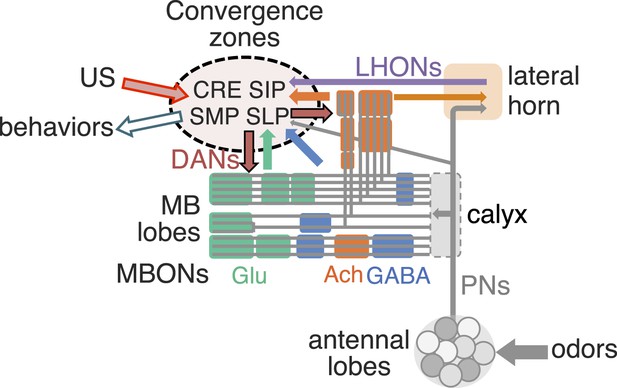
Schematic of the proposed convergence zone.
MB lobes consist of three groups of compartments based on the putative transmitter of MBONs (glutamate, GABA, and acetylcholine, shown color-coded). These compartments are interconnected inside the lobes and the MBONs send converging outputs (color-coded arrows) to small subregions within five neuropils: the lateral horn (LH), CRE, SMP, SIP, and SLP. The dendrites of DANs are also confined mostly in the convergence zones within the CRE, SMP, SIP, and SLP. These neuropils, therefore, must receive information encoding the unconditioned stimuli (US), such as sugar and shock, which recruit DANs for memory formation. In the simplest model, these same sensory inputs would activate not just the DANs but also other output neurons of these neuropils that elicit appropriate unconditioned behaviors. MBON, conveying the learned valence of stimuli, might then terminate onto these same output neurons. A subset of projection neurons from the antennal lobe and LH output neurons (LHONs) also converge with MBONs in these zones (see accompanying paper Aso et al., 2014). In this way, learned and innate responses could use a common set of downstream circuits, originating in the LH, CRE, SMP, SIP, and SLP, to drive behavior.
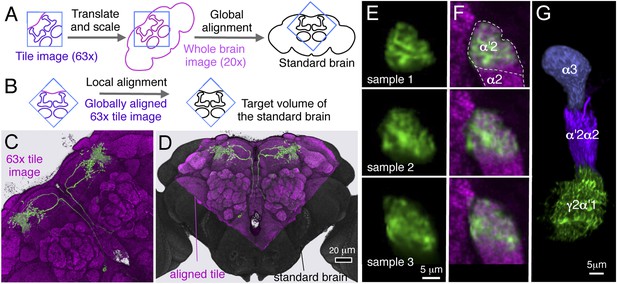
Alignment of partial brain images to a standard brain model.
(A) A low-resolution (20×) confocal stack covering the entire brain and optic lobes (magenta diagram) and a single high-resolution (63×) confocal stack of the portion of the brain containing the MB (blue square) are collected for each specimen. The 63× tile image is first aligned to a whole brain image of the same brain by scaling and rigid translation using labeling of the presynaptic active zone protein Bruchpilot by the mouse monoclonal antibody nc82 (Laissue et al., 1999; Hofbauer et al., 2009) as the reference. The whole brain image is then globally aligned to the JFRC2013 standard brain (black diagram) by affine registration. In this way the high-resolution tile image is globally aligned to the standard brain and corresponding target volume in the standard brain is identified. (B) The nc82 pattern of the high-resolution tile image is non-rigidly aligned to that of the standard brain. See ‘Materials and methods’ for more detail. (C) A 63× image tile of a brain (green, MB018B-driven expression pattern showing MBON-α′2 neurons; magenta, nc82 staining). (D) The same tile after alignment (gray; nc82 pattern of the standard brain). (E) Portions of optical slices from confocal stacks of three brains from the MB018B driver line. (F) After alignment, the dendrites of MBON-α′2 neurons in all three samples can be seen to be confined within the α′2 compartment of the standard brain. (G) An alignment to the standard brain of three separately imaged brains, each of which visualized a different PPL1 cluster dopaminergic neuron; compare to Figure 11F, where the same three neurons were imaged in a single brain using MCFO.
Videos
Introduction to MB anatomy and the olfactory circuit.
https://doi.org/10.7554/eLife.04577.004KCs.
https://doi.org/10.7554/eLife.04577.017MBONs.
https://doi.org/10.7554/eLife.04577.018PAM and PPL1 cluster DANs.
https://doi.org/10.7554/eLife.04577.019Arrangement of MBONs by transmitter type.
MBONs was color-coded based on putative neurotransmitter as in Figure 9: green, glutamatergic; blue, GABAergic; red, cholinergic.
Convergence of MBON-α3 with MBON-α1.
Two-color labeling of MBON-α3 and MBON-α1 by G0239 and R71C03-LexA as in Figure 20K. MBON-α1 was segmented from the pattern of R71C03-LexA. The video displays the projection patterns of both MBON types, then zooms to the SIP, where terminals of MBON-α3 and MBON-α1 are located, often within submicron distances of one another.
Tables
List of cell types in the mushroom body
Categories* | Putative transmitter† | Neurites in calyx or lobes‡ | Cell type names§ | Short names | Number of cells | Drivers# | Other names in literature¶ | Reference** | |
---|---|---|---|---|---|---|---|---|---|
MB-intrinsic neurons | Kenyon cells | C, L | γd | ∼75 | MB607B, MB419B | Aso 2009 | |||
C, L | γmain | ∼600 | MB131B | Aso 2009 | |||||
C, L | α′/β′ap | ∼210 | MB463B | α′/β′a, α′/β′p | Tanaka 2008 | ||||
C, L | α′/β′m | ∼140 | MB418B | Tanaka 2008 | |||||
C, L | α/βp | ∼90 | MB371B | Tanaka 2008, Lin 2007 | |||||
C, L | α/βs | ∼500 | MB185B, MB477B | Tanaka 2008, Lin 2007 | |||||
C, L | α/βc | ∼400 | MB594B | Tanaka 2008, Lin 2007 | |||||
modulatory neurons | 5HT amn | C, L | MB-DPM | 1 | DPM | Waddell 2000 | |||
GABA | C, L | MB-APL | 1 | VT43924 | Tanaka 2008 | ||||
MB-extrinsic neurons | MB output neurons (MBONs) | glutamate | L | MBON-γ5β′2a | MBON-01 | 1 | MB011B, MB210B (MB002B) | MB-M6 | Tanaka 2008 |
L | MBON-β2β′2a | MBON-02 | 1 | MB399B | |||||
L | MBON-β′2mp | MBON-03 | 1 | MB002B, MB011B, MB210B | MB-M4 | Tanaka 2008 | |||
L | MBON-β′2mp_bilateral | MBON-04 | 1 | MB011B | |||||
L | MBON-γ4>γ1γ2 | MBON-05 | 1 | MB434B, MB298B | |||||
L | MBON-β1>α | MBON-06 | 1 | MB434B, MB433B | MB-MV2 | Tanaka 2008 | |||
L | MBON-α1 | MBON-07 | 2 | MB310C, MB323B, MB319C | |||||
GABA | L | MBON-γ3 | MBON-08 | 1 | MB083C, MB110C | ||||
L | MBON-γ3β′1 | MBON-09 | 1 | MB083C, MB110C | |||||
L | MBON-β′1 | MBON-10 | 8†† | MB057B, MB078C | |||||
L | MBON-γ1pedc>α/β | MBON-11 | 1 | MB112C, MB085C, MB262B | MB-MVP2 | Tanaka 2008 | |||
acetylcholine | L | MBON-γ2α′1 | MBON-12 | 2 | MB077B, MB051B, MB090C | ||||
L | MBON-α′2 | MBON-13 | 1 | MB018B, MB091C | MB-V4 | Tanaka 2008 | |||
L | MBON-α3 | MBON-14 | 2 | MB082C, MB093C, G0239 | MB-V3 | Tanaka 2008, Chiang 2011 | |||
L | MBON-α′1 | MBON-15 | 2 | MB543B | |||||
L | MBON-α′3ap | MBON-16 | 1 | MB027B, MB549C | MB-V2α′ | Tanaka 2008, Sejourne 2011 | |||
L | MBON-α′3m | MBON-17 | 2 | MB027B | MB-V2α′ | Tanaka 2008, Sejourne 2011 | |||
L | MBON-α2sc | MBON-18 | 1 | MB549C, MB080C, MB050B | MB-V2α | Tanaka 2008, Sejourne 2011 | |||
L | MBON-α2p3p | MBON-19 | 2 | MB542B, (MB062B) | |||||
N.D. | L | MBON-γ1γ2 | MBON-20 | 1 | (VT999036) | ||||
L | MBON-γ4γ5 | MBON-21 | 1 | (VT999036) | |||||
C | MBON-calyx | MBON-22 | 1 | MB622B, MB242A | MB-CP1 | Tanaka 2008 | |||
modulatory neurons | dopamine | L | PAM-γ5 | PAM-01 | 8–21## | MB315C, MB335C | aSP13, MB-M1? | Tanaka 2008, Keleman 2012 | |
L | PAM-β′2a | PAM-02 | 6–9 | MB109B | |||||
L | PAM-β2β′2a | PAM-03 | >3 | MB301B, NP5272 | MB-M3, MB-M1? | Tanaka 2008 | |||
L | PAM-β2 | PAM-04 | 8–19 | MB209B | MB-M8 subset | Perisse 2013 | |||
L | PAM-β′2p | PAM-05 | 14–17 | MB056B | MB-M5? MB-AIM? | Tanaka 2008 | |||
L | PAM-β′2m | PAM-06 | 12–15 | MB032B | MB-M5? MB-AIM? | Tanaka 2008 | |||
L | PAM-γ4<γ1γ2 | PAM-07 | 13–17 | MB312B | subset of MB-AIM? | Tanaka 2008 | |||
L | PAM-γ4 | PAM-08 | MB312B | subset of MB-AIM? | Tanaka 2008 | ||||
L | PAM-β1ped | PAM-09 | 1–3 | (MB194B) | subset of MB-MVP1 | Tanaka 2008 | |||
L | PAM-β1 | PAM-10 | 4–6 | MB063B | subset of MB-MVP1 and MB-M8 | Tanaka 2008, Perisse 2013 | |||
L | PAM-α1 | PAM-11 | >6 | MB043B, MB299B | subset of MB-MVP1, MB-VP1 | Tanaka 2008 | |||
L | PAM-γ3 | PAM-12 | 9–23 | MB441B | MB-M2 | Tanaka 2008 | |||
L | PAM-β′1 ap | PAM-13 | 13–14 | MB025B | subset of MB-AIM? | Tanaka 2008 | |||
L | PAM-β′1 m | PAM-14 | MB025B | subset of MB-AIM? | Tanaka 2008 | ||||
L | PPL1-γ1pedc | PPL1-01 | 1–2 | MB438B | MB-MP1, MP | Tanaka 2008, Krashes 2009 | |||
L | PPL1-γ1‡‡ | PPL1-02 | 1 | (TH-GAL4) | |||||
L | PPL1-γ2α′1 | PPL1-03 | 1 | MB296B, MB439B | MB-MV1 | Tanaka 2008 | |||
L | PPL1-α′3 | PPL1-04 | 1 | MB304B | Mao 2008 | ||||
L | PPL1-α′2α2 | PPL1-05 | 1 | MB058B | MB-V1 | Tanaka 2008 | |||
L | PPL1-α3 | PPL1-06 | 1 | MB065B, MB060B | Mao 2008 | ||||
C | PPL2ab§§ | >1 | (TH-GAL4) | Mao 2008 | |||||
C | PPL2ab | >1 | (TH-GAL4) | Mao 2008 | |||||
octopamine | C, L | OA-VPM3 | 1 | MB022B | Busch 2009 | ||||
L | OA-VPM4 | 1 | MB022B, MB021B, MB113C | Busch 2009 | |||||
C | OA-VPM5 | 1 | (TDC2-GAL4) | Busch 2009 | |||||
C | OA-VUM2a | 2 | (TDC2-GAL4, NP7088) | Busch 2009 | |||||
5HT | C | CSD | 1 | MB465C | Roy 2007 | ||||
SIFamide | C, L | SIFamide | 4 | MB013B | MB-C2? | Verleyen 2004, Tanaka 2008 | |||
GABA | C | MB-C1 | >2 | MB380B | Tanaka 2008 |
-
MB intrinsic and extrinsic cell types are listed. The number of cells in each cell type per brain hemisphere is given. We have made split-GAL4 lines that drive expression in most cell types and these are listed. Supplementary file 1 lists the cell types in which expression is observed with each split-GAL4 driver. For the remaining cell types, the GAL4 line used to visualize individual neuron(s) within each cell type is given; in nearly all cases, these lines have much broader expression than the split-GAL4 lines. MBON cell types were characterized at the single cell level (see text for details). For ten MBON cell types, the relevant split-GAL4 lines label only one cell per hemisphere. For other MBONs, the relevant split-GAL4 lines expressed in two or more cells per hemisphere and we used MCFO and determined single cell morphologies to classify cell types.
-
*
The MB neurons were categorized into two major groups of cell types: the MB-intrinsic neurons that have all processes in the MB and thus contribute to local signal processing inside the MB, whereas the MB-extrinsic neurons are any neurons that connect the MB with other neuropils irrespective of polarity and transmitter. We defined MBONs as neurons that have dendrites in the MB. The antennal lobe projection neurons are also MB-extrinsic neurons by definition, but not listed. The polarity of neurons was often obvious in high-resolution confocal images of membrane labeled cells (Figure 8A,B) in which presynaptic terminals and dendrites exhibit distinctive morphologies. However, in each case we confirmed the polarity using a reporter that localizes to presynapses by virtue of the synaptic vesicle protein Synaptotagmin (pJFRC51-3xUAS-Syt::smGFP-HA in su(Hw)attP1; Figure 8C). In addition to the 21 MBON cell types, one neuron (MBON-calyx, or MB-CP1) extends dendrites in the main calyx, the posterior part of the pedunculus and the ventral part of the lateral horn, and thus we classify this as an atypical MB output neuron (see Figure 3—figure supplement 1).
-
†
Four of the MBON cell types have been previously described as ChAT immunoreactive (Sejourne et al., 2011; Placais et al., 2013), and we observed consistent results. Likewise, our results with anti-dVGluT staining are consistent with conclusions based on the expression pattern of an enhancer trap line in the dVGluT locus, OK371 (Johard et al., 2008). N.D.; not determined.
-
‡
Neurite distribution inside the MB is indicated: L, lobes; C, calyx.
-
§
As described in the text, MB-extrinsic neurons project to specific compartments in the MB lobes with minimal overlap. This allowed us to adopt a nomenclature for MB-extrinsic neurons based on their projection pattern in the MB: for MBONs, we use MBON plus the name of MB lobe compartments in which their dendrites arborize (e.g., MBON-α1 for the MB output neurons that have dendrites in the α1 compartment); for DANs, we use the name of cluster to which they belong, either PPL1 or PAM, followed by the name of the MB compartments in which their axon terminals are found (e.g., PAM-α1 for the dopaminergic neurons that terminate in α1). For the MBONs with axon terminals in the MB lobes, the ‘>’ symbol followed by the compartments, or the lobes, in which the axon terminals innervate is appended to the dendritic compartment (e.g., MBON-β1>α for the MB output neuron with dendrites in β1 and presynaptic terminals in the α lobe). For the DAN type with dendrites in the MB lobes, the ‘<’ symbol followed by the compartments in which its dendritic arbors elaborate is appended to the compartment with axon terminals (e.g., PAM-γ4<γ1γ2 for the PAM neuron that terminates in γ4 and has dendrites in γ1 and γ2). We also provide a short name for each MBON and DAN cell type of the format MBON-01 to MBON-22, PAM-01 to PAM-14, and PPL1-01 to PPL1-06.
-
#
Bold font indicates lines highly recommended for behavioral experiments based on specificity and intensity. The best line for behavioral experiments depends on the UAS effectors. See Supplementary file 1 for more information.
-
¶
Some of the cell types we define here were previously named or included in a mixture of cell types with a single name. Our judgment of the most likely correspondence is given. Some assignments are uncertain, as indicated by a question mark.
-
**
See reference list in the main text.
-
††
Unlike other MBON cell types, the number of MBON-β′1 cells in MB057B varied between 7 and 9 (8.0 on average, SEM = 0.17, n = 12) across individuals. Also, the distribution of dendrites in the β′1 compartment differed among 24 single cells obtained with MCFO. Some extend dendrites in both ap and m layers, but others extend dendrites only in one of the layers. Three of 24 single cells examined exhibit very sparse dendritic arbors in the β′1 compartment.
-
‡‡
A sixth cell type in the PPL1 cluster that sparsely innervates the γ1 MB compartment has been observed in stochastic labeling experiments using the TH-GAL4 driver (PPL1-γ1) (Igor Siwanowicz, pers. comm.), but we were not able to generate a split-GAL4 for this cell type.
-
§§
Two cell types of DANs in PPL2ab cluster have been identified (Mao and Davis, 2009).
-
##
Numbers of cell for each PAM cluster DAN cell type were estimated by counting labeled cells in split-GAL4 (minimal estimate) or PA-GFP experiments (maximal estimate). See Figure 5 for detail.
Additional files
-
Supplementary file 1
List of split-GAL4 driver lines for the neurons in the mushroom body. For each driver line, the source of the enhancer fragments used to make the p65ADZp and ZpGAL4DBD constructs are given. Most enhancer fragments are from the collection of GAL4 lines described in Jenett et al. (2012); see ‘Materials and methods’ for the details of the TH and TDC2 constructs. All ZpGAL4DBD constructs are inserted into attP2; the insertion sites of the p65ADZp constructs are indicated by the letter at the end of the driver name as follows: A, su(Hw)attP8; B, attP40; C, VK00027. The cell types in which expression is seen with each driver are indicated in the matrix; the level of expression in each cell type is indicated by the gray scale (see ‘Materials and methods’). Note that observed expression depends to some extent on the reporter construct used (see Figure 2—figure supplement 1 for an example). The data in this matrix were based on imaging with pJFRC225-5xUAS-IVS-myr::smGFP-FLAG in VK00005. The VK00005 insertion site supports high-expression levels (Pfeiffer et al., 2012); many of the split-GAL4 drivers show more cell type specificity using reporters in attP18 (see the accompanying paper for examples) (Aso et al., 2014). We did not observe expression outside the central nervous system in any of these lines except MB062C; see ‘Materials and methods’ and Figure 2—figure supplement 7 for a description of the assay used.
- https://doi.org/10.7554/eLife.04577.045