Cellular interpretation of the long-range gradient of Four-jointed activity in the Drosophila wing
Figures
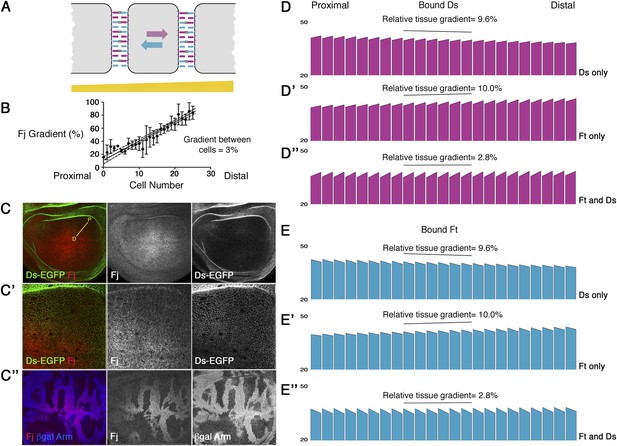
Computational modelling of the effect of a Fj gradient on Ft–Ds binding.
(A) Cartoon illustrating Ft–Ds binding and the predicted effect of a Fj gradient on the distribution of Ft–Ds heterodimers in a single cell. In the absence of any gradients (i.e., uniform Ft, Ds, and Fj expression across the tissue), Ft (blue) and Ds (purple) freely associate at junctions, with a certain proportion binding to form heterodimers (indicated by double black bars). We predict that by adding a Fj gradient (yellow bar) an asymmetric distribution of Ft–Ds binding across a cell will occur. If Fj inhibits Ds binding and promotes Ft binding more strongly to the right, Ft molecules in each cell prefer to bind to Ds in the next left-most cell (with which they have a stronger binding interaction), and so preferentially accumulate at left cell edges; similarly Ds molecules prefer to associate with Ft in the next right-most cell and in turn accumulate at right cell edges. Hence, the overall consequence of a Fj gradient and free movement of Ft and Ds within cells is the generation of cellular asymmetries of Ft and Ds distributions. (B) Graph illustrating the proximal-distal measurements of the Fj gradient across Drosophila third instar larval wing discs, showing a typical gradient of around 3% (dashed lines indicate 95% confidence intervals, error bars indicate standard deviation (SD), n = 5). Cell number increases from 0 to 30 moving from proximal to distal. (C) Confocal images of wing discs from animals with EGFP knocked-in to the endogenous ds locus, stained for Fj (red) and observed for native GFP fluorescence (green). A yellow line indicates where Fj gradient measurements were taken from proximal [P] to distal [D]. (C′) An example zoomed-in image showing the distribution of Fj and Ds in this region. (C″) fjd1 mutant clones (absence of blue ß-gal staining, cell junctions also labelled in blue) demonstrate that Fj is expressed throughout the tissue. Mutant clones were used to subtract background from the gradient measurements. (D and E) A computational model of Ft–Ds binding at cellular junctions. Each bar represents one cell and the scale indicates the amount of bound Ds or Ft in arbitrary units at junctions between cells (note scale is cut off at 20 units), also see Figure 1—figure supplement 1. Sloped top edges represent the difference between proximal and distal cell edges within one cell. A more graded slope within a cell indicates an increase in asymmetry. 23 cells are represented and the observed Fj gradient of 3% (B) has been used. Looking at both bound Ds (D) and bound Ft (E) shows Ds is preferentially localised distally and Ft proximally. If Fj acts on Ds only (D and E), weak asymmetry is seen within cells (3.8% increase) and a tissue gradient of higher to lower Ft–Ds binding is seen as Fj increases. If Fj acts on Ft only (D′ and E′) similar weak asymmetry (3.6% increase) is seen within cells, however, a tissue gradient of lower to higher Ft–Ds binding is observed as Fj increases. If Fj can act on both Ft and Ds (D″ and E″) stronger asymmetry is seen within cells (7.9% increase) and the tissue gradient is much reduced.

Representation of mathematical model.
(A) one-dimensional row of cells with proximal (P) and distal (D) membranes in cells 1 to i.
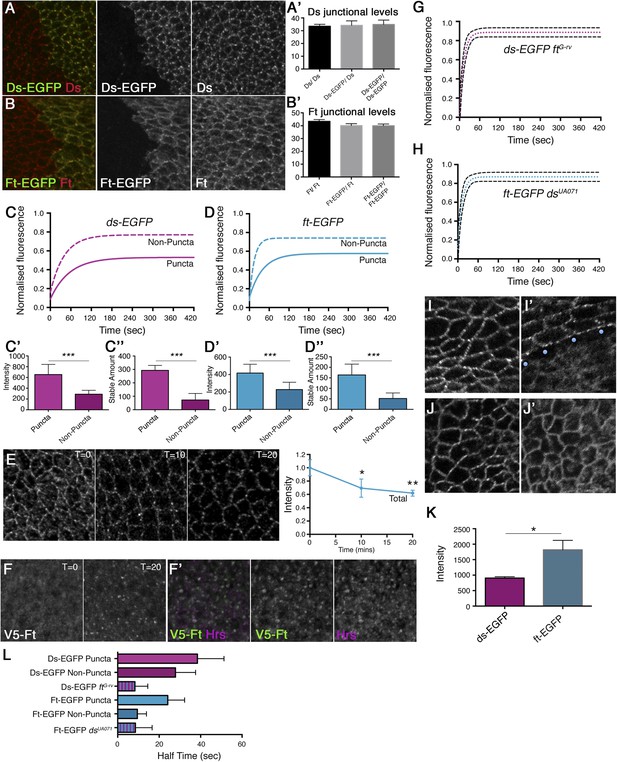
Ft and Ds show mutually dependent stable fractions at junctions.
Wing discs containing clones of (A) ds-EGFP/+ and (B) ft-EGFP/+ labelled for EGFP (green) and Ds or Ft, respectively (red), show that addition of an EGFP tag does not affect protein localisation. Quantification of (A′) Ds or (B′) Ft junctional protein confirms expression levels of tagged proteins are similar to wild-type. FRAP analysis of (C) Ds-EGFP and (D) Ft-EGFP puncta (bright regions, solid line) and non-puncta (dashed line) reveals a large stable fraction of protein in puncta (see Figure 2—figure supplement 1 for an example of a puncta and non-puncta region and Figure 2—figure supplement 2 for FRAP graphs with individual data points). Multiplying the intensity of FRAP regions prior to bleaching (C′ and D′) by the stable fraction plateau (C and D) reveals a final ‘stable amount’ of protein at junctions (C″ and D″) and a large significant difference between puncta and non-puncta regions (both p = 0.0001, error bars denote standard error (SEM) here and in all remaining figures). (E) Apical confocal sections of a V5-Ft antibody internalisation assay in pre-pupal wing, with time points of 0, 10, and 20 min, reveal persistent Ft puncta over time while quantification of total apical levels (right panel) shows protein internalisation is occurring (p = 0.01 at 10 min and p = 0.001 at 20 min) (F) Sub-apical confocal sections of pre-pupal wing show V5-Ft previously at the cell surface is seen in discrete intracellular puncta at t = 20, where it co-localises with early endosome marker Hrs (magenta) (F′), confirming antibody internalisation rather than antibody drop off. FRAP analysis of Ds-EGFP in ftG-rv clones (G) and Ft-EGFP in a dsUA071 background (H) resulted in more rapid and increased protein recovery. Dashed lines denote 95% confidence intervals. As recovery did not reach 100%, a small stable population could remain. Also see Figure 2—figure supplement 4 for FRAP graphs with individual data points. Re-bleaching experiments suggest that this is not an experimental artefact (see Figure 2—figure supplement 5). Live images of Ds-EGFP (I) and Ds-EGFP with a ftG-rv clone (I′; blue dots indicate first row of mutant cells) show a loss of puncta and diffuse distribution of Ds-EGFP when Ft is not present. Similar distribution of Ft-EGFP (J) is seen in a dsUA071 mutant (J′). (K) Comparison of Ds-EGFP and Ft-EGFP protein levels taken at the same confocal settings suggests there is almost twice as much Ft-EGFP (n = 4 wings) as Ds-EGFP (n = 2 wings) in puncta regions (p = 0.01). Intensity measurements were taken from manually selected puncta using 1 µm2 ROIs. Around 50 ROI measurements were taken per wing and average intensities were plotted and analysed using an unpaired t-test. (L) Bar chart representing time taken for 50% protein recovery to occur during FRAP experiments. Both Ft-EGFP and Ds-EGFP mobile fractions exhibit slower recovery in puncta compared to non-puncta and mutants.
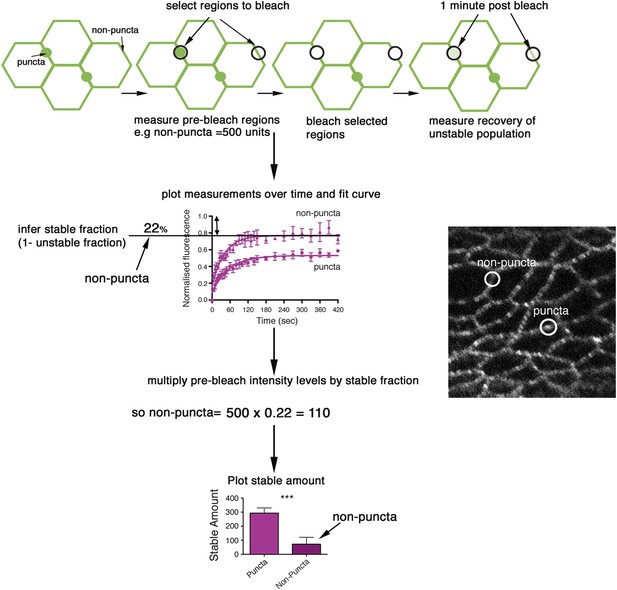
Description of FRAP method.
A cartoon flowchart describing the steps involved in a FRAP experiment. Initially, puncta and non-puncta regions are selected and their intensity measured (pre-bleach intensity). The regions are bleached for a few seconds by increasing the laser power. Bleached regions are imaged over time and recovery of the unstable population is measured for around 5 min. Measurements are plotted and a one-phase exponential curve is fitted to the data. The plateau of this curve gives us the unstable fraction and to get the stable fraction we minus the unstable fraction from 1, for example, 1–0.78 = 0.22. This figure is multiplied by the pre-bleach intensity to give the stable amount. So if, for example, 78% unbleached protein has recovered, 22% of the bleached protein in the region of interest is then the stable fraction. We multiply this by the original pre-bleach intensity. So, a starting intensity of 500 units is multiplied by 0.22 to get a final stable amount of 110 units. However, if a mutant has a starting intensity of 250 and the same stable fraction of 22% we would say the stable amount of the mutant is 55. The mutant has therefore much less stable protein at junctions despite having a similar stable fraction. An example of puncta and non-puncta regions in ds-EGFP is also shown.
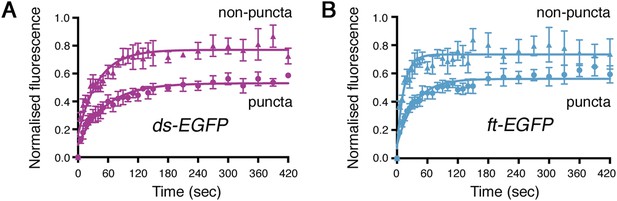
Individual data points for FRAP experiments.
FRAP graphs containing individual data points with SEM for each experiment. (A) ds-EGFP homozygous puncta and non-puncta. (B) ft-EGFP homozygous puncta and non-puncta.
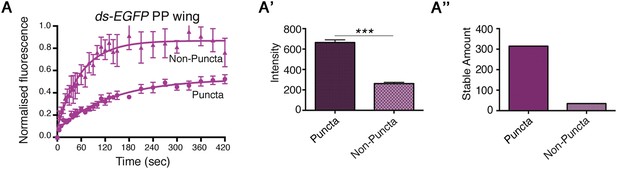
FRAP on Ds-EGFP in the pre-pupal wing.
(A) FRAP on distal pre-pupal wings demonstrates a difference between puncta and non-puncta in the same wing region as the internalisation assay, confirming the presence of stable and unstable protein populations. (A′) Intensity of puncta and non-puncta regions in the pre-pupal wing. (A″) Stable amount of puncta and non-puncta in the pre-pupal wing confirms a large difference in stability between regions.
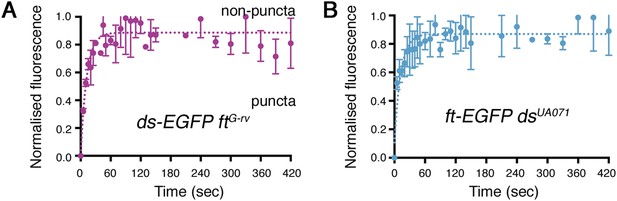
Individual data points for FRAP experiments.
FRAP graphs containing individual data points with SEM for each experiment. (A) ds-EGFP ftG-rv clones (no puncta observed). (B) ft-EGFP dsUA071 (no puncta observed).
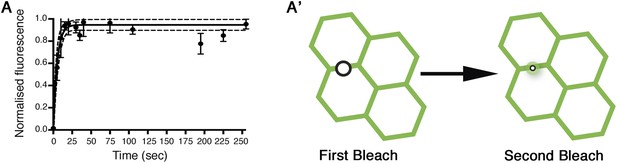
Control re-bleach FRAP experiments.
(A) Re-bleach experiment performed on ft-EGFP dsUA071. The re-bleach experiment confirms there are no obvious bleaching artefacts in our FRAP experiments. A FRAP region is selected and bleached as normal (see ‘Materials and methods’), recovery is allowed for 2 min prior to a second bleaching event which is performed in a smaller region within the first bleached region (A′). Recovery is measured and reaches around 100%. If there were any bleaching artefacts (such as photodamage-induced stabilisation of a protein population), recovery after the second bleach would not reach 100%.
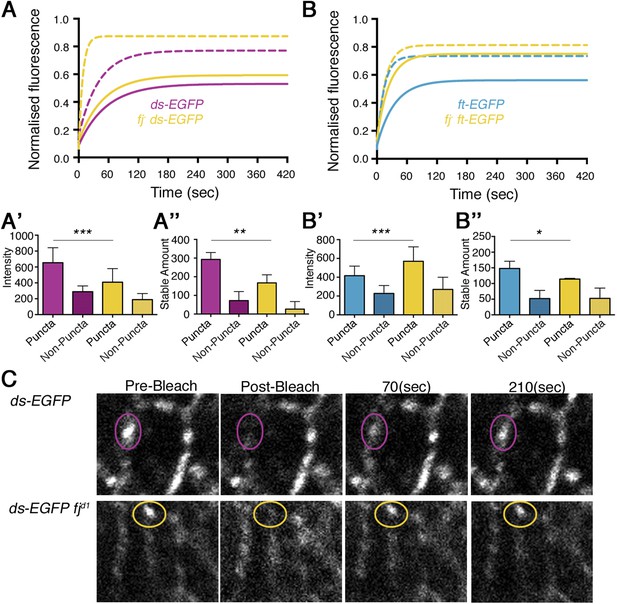
Ft and Ds stability upon loss of Fj.
Stable fraction of (A) Ds-EGFP and (B) Ft-EGFP in a fjd1 background (yellow lines). Solid lines represent puncta and dashed lines represent non-puncta. ‘Wild-type’ values are plotted for comparison. Intensity of Ds-EGFP decreases (A′) and Ft-EGFP increases (B′), in a fjd1 background, whereas stable amount of protein in puncta falls in both (A″ (p = 0.004) and B″ (p = 0.04)). The reduction in Ds-EGFP levels at junctions in the absence of Fj is consistent with less Ds being bound to Ft in this situation and excess unbound Ds being removed from junctions. The reason for an increase in Ft levels is unknown, as presumably less Ft is bound to Ds, but suggests that unbound Ft is not removed from junctions. See Figure 3—figure supplement 1 for FRAP curves with individual data points. (C) Live images of wing discs taken during a FRAP experiment show the recovery of puncta over time in ds-EGFP and ds-EGFP in a fjd1 background. Protein recovery after 70(sec) is increased in a fjd1 background and overall protein levels appear reduced.
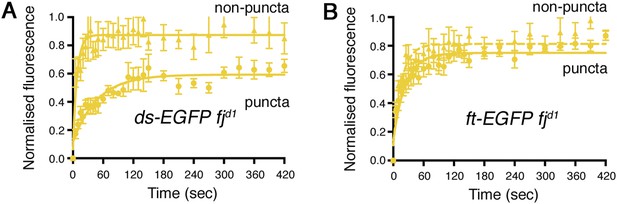
Individual data points for FRAP experiments.
FRAP graphs containing individual data points with SEM for each experiment. (A) ds-EGFP fjd1 puncta and non-puncta. (B) ft-EGFP fjd1 puncta and non-puncta.
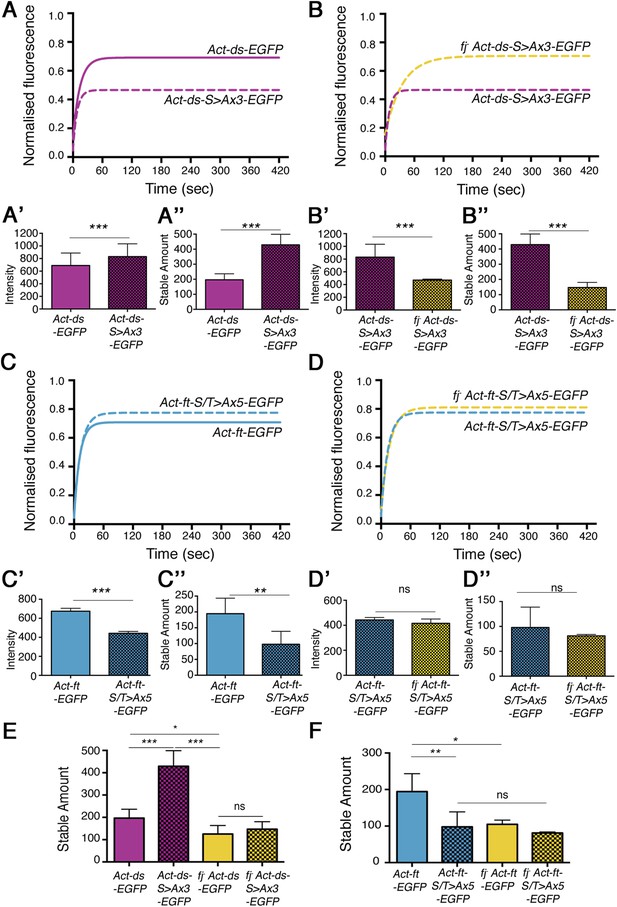
Effects of mutating the phosphorylation sites in Ds and Ft on in vivo stability.
(A) Comparison of Act-ds-EGFP with Act-ds-EGFP phosphomutant (Act-ds-S>Ax3-EGFP). FRAP is performed on junctions as no puncta are visible. Ds-S>Ax3-EGFP has a larger remaining stable fraction after bleaching (A), is more highly expressed at junctions (A′) and has a significantly larger stable amount at junctions (A″ p = 0.0004) than ‘wild-type’. (B) When Fj is removed from the phosphomutant background (fj− Act-ds-S>Ax3-EGFP) intensity levels drop (B′) and the stable amount at junctions returns to below ‘wild-type’ levels (B″ and E). (C) Ft-EGFP phospho-mutant (Act-ft-S/T>Ax5-EGFP) has a smaller stable fraction than Ft-EGFP (Act-ft-EGFP), shows reduced levels at junctions (C′), and has a significantly reduced stable amount at junctions (C″, p = 0.006). (D, D′, D″) In a fjd1 mutant background, the phosphomutant protein (fj- Act-ft-S/T>Ax5-EGFP) does not become any less stable suggesting any loss of stability in (C) is caused primarily by a loss of Ft phosphorylation. See Figure 4—figure supplement 1 for FRAP graphs with individual data points. (E–F) An overview of stable amounts in (E) Act-ds-EGFP and (F) Act-ft-EGFP shows that despite there being a significant increase in stable Ds when its phosphorylation sites are mutated, the removal of Fj results in a loss of stable Ds (E; p = 0.02) and also Ft (F; p = 0.02). Thus the phosphorylation state of Ds only appears to significantly affect Ft–Ds binding if Ft is already phosphorylated by Fj.
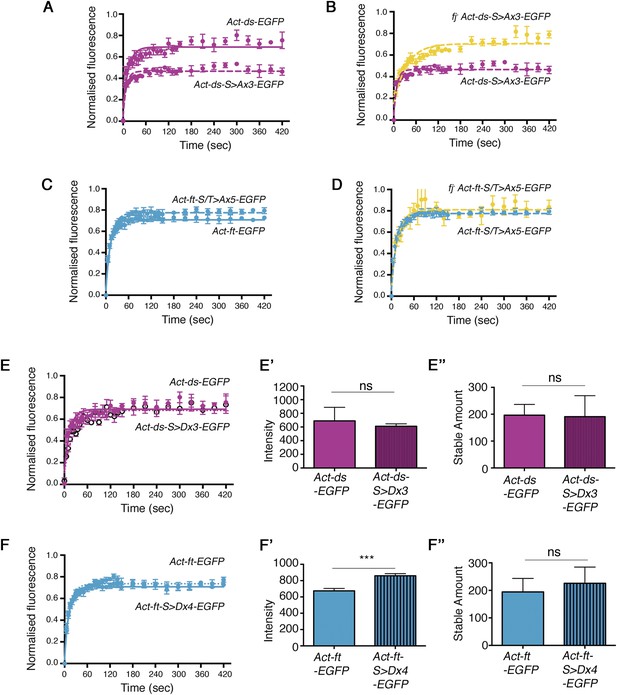
Individual data points for FRAP experiments.
FRAP graphs containing individual data points with SEM for each experiment. (A) Act-ds-EGFP and Act-ds-S>Ax3-EGFP (no puncta available). (B) Act-ds-S>Ax3-EGFP and fj-Act-ds-EGFP S>Ax3-EGFP (no puncta observed). (C) Act-ft-EGFP and Act-ft-S/T>Ax5-EGFP (no puncta observed). (D) Act-ft-S/T>Ax5-EGFP and fj- Act-ft-S/T>Ax5-EGFP (no puncta observed). (E) Comparison of Act-ds-EGFP and Act-ds-EGFP phosphomimetic (Act-ds-S>Dx3-EGFP). The expected result upon expression of a ds phosphomimetic might be a reduction in stable fraction and stable amount. However, the Act-ds-S>Dx3-EGFP stable fraction is the same as Act-ds-EGFP (E), intensity levels are similar (E′) and stable amounts are not significantly different (E″) suggesting that mutating the phosphorylation sites has not been successful or does not have any additional effect (see text). (F) Comparison of Act-ft-EGFP and Act-ft-EGFP phosphomimetic (Act-ft-S/T>Dx4-EGFP). Mimicking the phosphorylation sites in Act-ft-EGFP might be expected to increase both the stable fraction and stable amount of protein when compared to ‘wild-type’ Act-ft-EGFP. However, the stable fraction remains the same (F), and although intensity levels of the phosphomimetic are higher (F″), the stable amount is not significantly increased (F″).
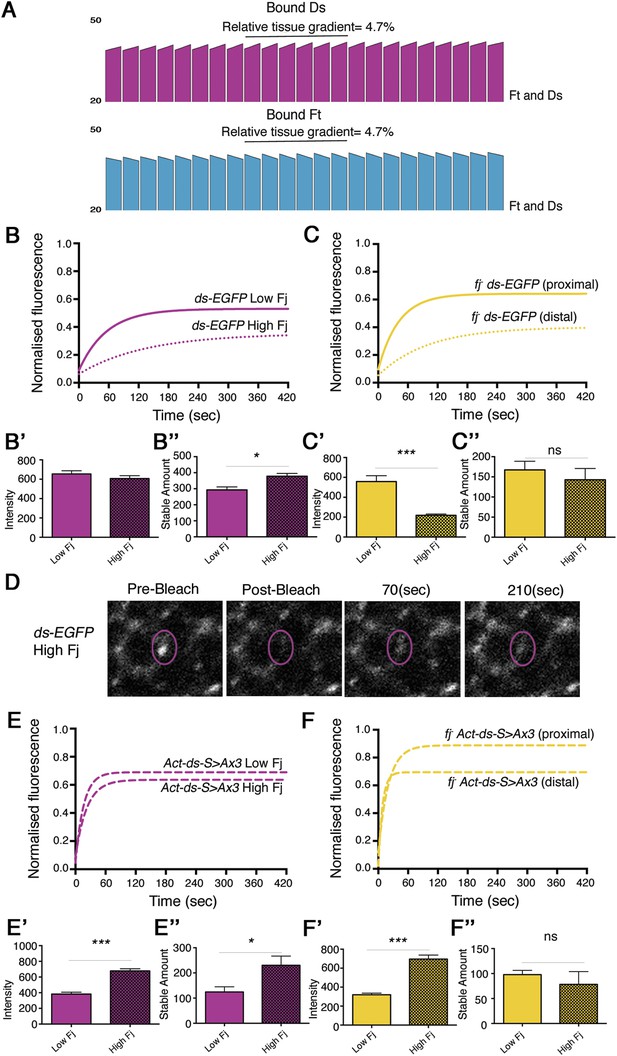
Ft–Ds binding varies across the wing in response to the Fj expression gradient.
(A) Output of the revised computational model in which Fj acts more strongly on Ft than Ds, and in which unphosphorylated Ft binds with equal affinity to both phosphorylated and unphosphorylated Ds. When Fj can act on both Ft and Ds with a Ft bias, an intermediate outcome between acting on Ft only and acting on Ft and Ds without a bias is seen and a cellular asymmetry of 4.1% is observed. A tissue gradient of 4.7% in the model when acting on both Ft and Ds, compared to 10% when Fj acts on Ft alone (Figure 1D′,E′) suggests that Ds phosphorylation is required to counter the strong graded effects of Ft phosphorylation. (B) FRAP analysis of endogenously expressed Ds-EGFP reveals a larger stable fraction in regions of high Fj (distal) despite showing slightly less overall fluorescence (B′). Overall, a significantly increased stable amount is seen in high Fj regions (B″ p = 0.01, 23% difference). (C) FRAP analysis in a fj mutant shows that this difference is lost when taking pre-bleach intensity levels into account (C′, C″). See Figure 5—figure supplement 1 for FRAP graphs with individual data points. (D) Images taken during a FRAP experiment in a region of high Fj demonstrate the low level of recovery over time when compared to Ds-EGFP recovery in a region of low Fj (as seen in Figure 3C). (E) FRAP analysis of junctionally localised Ds-EGFP expressed from the Act-ds-S>Ax3-EGFP transgene show overall reduced stable fractions, however, increased distal intensity levels of Ds-EGFP (E′) mean the overall stable amount is significantly increased in regions of high Fj (E″, p = 0.01, 53% difference). In a fjd1 mutant (F) any observed difference in stable amount across the tissue is lost (F″) when taking pre-bleach intensity levels into account (F′) (proximal equates to low Fj, distal equates to high Fj). This is again consistent with Fj acting primarily on Ft. See Figure 5—figure supplement 1 for FRAP curves with individual data points.
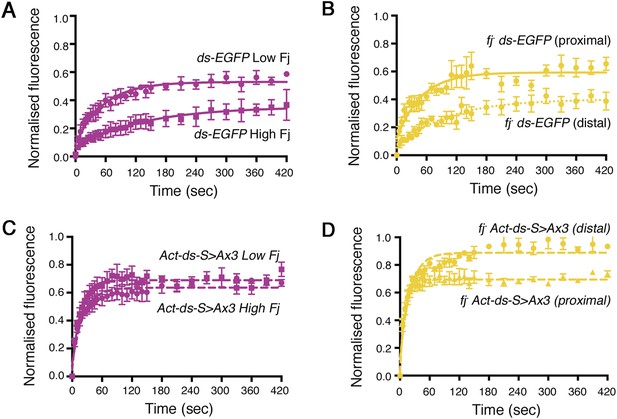
Individual data points for FRAP experiments.
FRAP graphs containing individual data points with SEM for each experiment. (A) ds-EGFP homozygous puncta at low (proximal) and high (distal) Fj regions. (B) ds-EGFP homozygous puncta at proximal and distal regions in a fj mutant. (C) Act-ds-S>Ax3-EGFP puncta at low (proximal) and high (distal) Fj regions. (D) Act-ds-S>Ax3-EGFP puncta at proximal and distal Fj regions in a fj mutant.
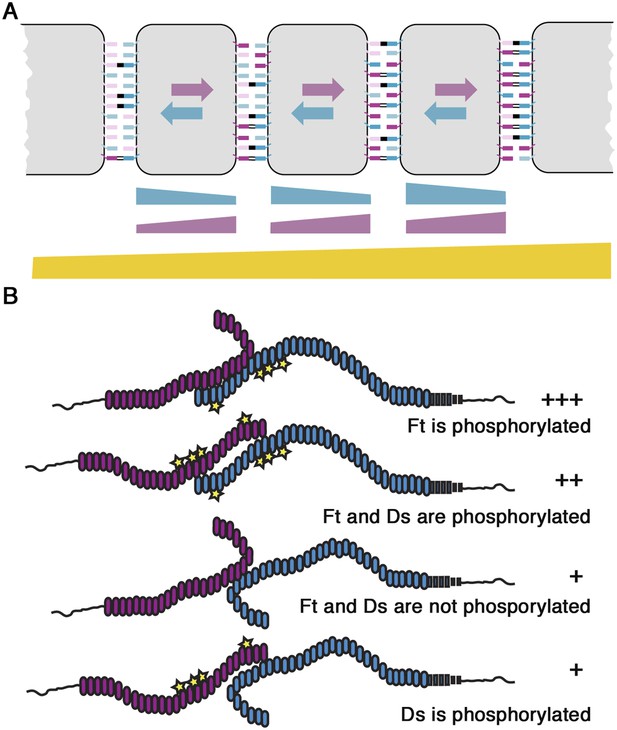
Models of Ft–Ds interactions.
(A) Cartoon illustrating our model of Ft–Ds binding across a tissue according to a Fj gradient. Ft-P (dark blue) and Ds-P (dark purple) increase, and Ft (light blue) and Ds (light purple) decrease as you move up the Fj gradient. Weak binding (no line) between heterodimers can occur between all populations, intermediate binding (double lines) can occur when Ft-P is available and the strongest bond (solid line) occurs only between Ft-P and Ds. As there is a bias towards Ft phosphorylation increasing binding, more intermediate binding occurs in regions of high Fj even though less favourable Ds (light purple) is available. In regions of low Fj, although there is more favourable Ds available, Ft-P is less abundant meaning fewer intermediate bonds are able to form. As Ft-P allows for stronger binding in cells with higher Fj, Ds in the next left-most cell preferentially accumulates to the right cell edge meaning the Fj gradient produces cellular asymmetry. A gradient of tissue binding is also produced with cells in the left-most cell producing fewer intermediate bonds than those in the right-most cell. (B) Conformational changes caused by the phosphorylation of Ft and Ds could provide an explanation for the differences in binding affinity caused by apparently similar phosphorylation events. The ‘open’ conformation of Ft and the ‘closed’ conformation of Ds may bind preferably such that if Ft is phosphorylated and Ds is not, binding is strongest (+++). If Ft and Ds are both phosphorylated, binding strength is intermediate (++). ‘Closed’ conformation of non-phosphorylated Ft produces the weakest binding and is not affected by Ds (+).
Tables
Comparison of rate and stabilisation data
Genotype | Stable amount | SEM of stable amount | Half time | Confidence interval |
---|---|---|---|---|
Ds-EGFP Homozygous Puncta | 292.9 | ±18.5 | 38.5 | 30.8 to 51.3 |
Ds-EGFP Homozygous Non-Puncta | 72.1 | ±21.8 | 27.9 | 22.13 to 37.6 |
Ds-EGFP ftG-rv Homozygous | n/a | n/a | 8.3 | 5.8 to 14.5 |
Ds-EGFP fjd1 Homozygous Puncta | 167.2 | ±21.6 | 33.89 | 25.5 to 50.5 |
Ds-EGFP fjd1 Homozygous Non-Puncta | 26.4 | ±20.5 | 5.6 | 3.8 to 10.3 |
Ft-EGFP Homozygous Puncta | 148.0 | ±9.5 | 24.2 | 19.28 to 32.3 |
Ft-EGFP Homozygous Non-Puncta | 52.0 | ±10.6 | 9.5 | 7.2 to 13.9 |
Ft-EGFP dsUA071 Homozygous | n/a | n/a | 8.5 | 5.7 to 16.6 |
Ft-EGFP fjd1 Homozygous Puncta | 114.1 | ±1.4 | 16.8 | 13.3 to 22.8 |
Ft-EGFP fjd1 Homozygous Non-Puncta | 52.6 | ±19.0 | 11.4 | 8.7 to 16.6 |
Ds-EGFP Homozygous Puncta Distal (High Fj) | 378.0 | ±17.6 | 93.0 | 59.1 to 217.6 |
Ds-EGFP fjd1 Homozygous Puncta Distal | 142.7 | ±28 | 69.7 | 51.3 to 108.6 |
Additional files
-
Supplementary file 1
Table contains numbers of wings, plateau data, and 95% confidence of plateau data for each FRAP experiment.
- https://doi.org/10.7554/eLife.05789.019