Circular RNA biogenesis can proceed through an exon-containing lariat precursor
Figures
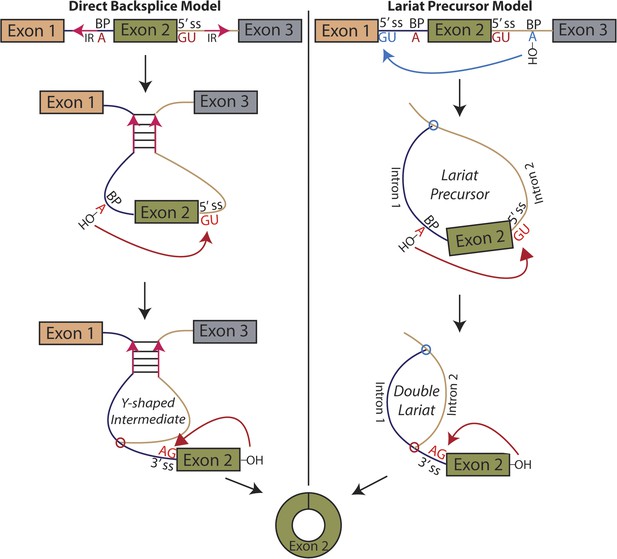
Models for the production of circular RNA.
(Left) Complementary sequences facilitate the biogenesis of circular RNA. (Right) Circular RNA biogenesis proceeds through a lariat precursor.
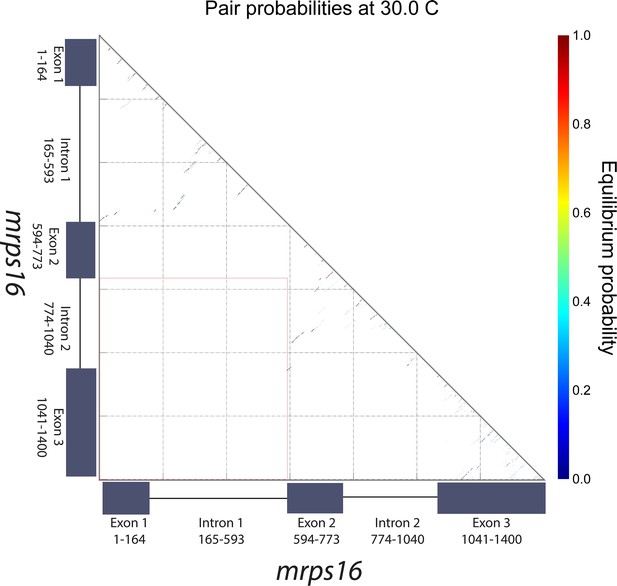
mrps16 pre-mRNA predicted pair probabilities.
Dot plot representation of the predicted minimum free energy (MFE) mrps16 pre-mRNA secondary structure. This structure was predicted using the NUPACK web server. The area highlighted by the orange box represents the region where interactions between flanking sequences would be predicted. The nature of the nearby long-range interactions between exon 2 and intron 2/exon 3 is illustrated in the MFE structure of the mrps16 pre-mRNA.
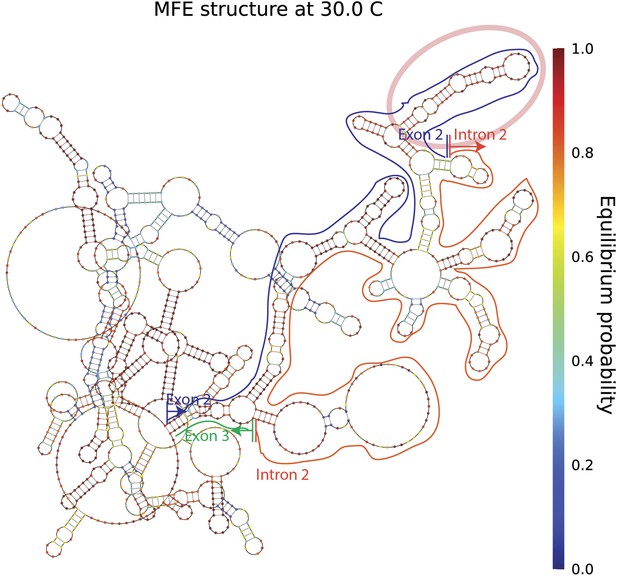
mrps16 pre-mRNA predicted MFE structure.
Long-range interactions between exon 2 and intron 2/exon 3 are highlighted above. The blue line traces the path of exon 2, the orange line traces the path of intron 2, and the green line traces the path of the first 17 nucleotides of exon 3. The vertical lines demarcate the start and end of each segment. Note, these predicted long-range interactions do not extend to regions beyond exon 2 and thus, no extensive base-pairing interactions are predicted to form between the segments of the gene that flank the exon (also depicted in the dot plot shown in Figure 1—figure supplement 1). The pink oval marks a highly structured region of exon 2, which is noted later in our discussion of exonic sequence deletions.
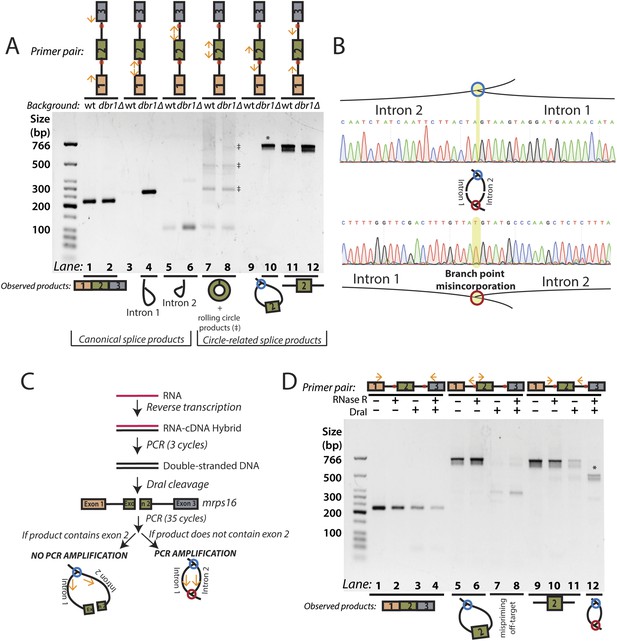
RT-PCR analysis of circular RNA biogenesis intermediates.
(A) reverse transcription-PCR (RT-PCR) of splice products and byproducts of mrps16 pre-mRNA in wild-type and dbr1∆ S. pombe. Primers used are indicated above each lane of the gel. Red dots represent the location of branch points. The observed products are shown below the gel. Laddering of bands in lanes 7 and 8 is due to rolling-circle reverse transcription of the 181 nt mrps16 circular RNA. (B) Sequencing trace for product in Figure 2A, lane 10 (Top) and Figure 2D lane 12 (Bottom) (each marked with * on respective gels). Due to the 2′–5′ linkage at the branch point, there is base misincorporation or nucleotide skipping at the branch point by the reverse transcriptase (highlighted in trace). Note, the top trace is mirrored for consistency with the orientation in the graphic representation. (C) Strategy to enrich for the byproduct of circular RNA backsplicing event. Since the circularized exon of mrps16 contains a DraI restriction site, we can deplete PCR products containing the exon. (D) RT-PCR results after RNase R and/or DraI treatment (treatment indicated above the gel).
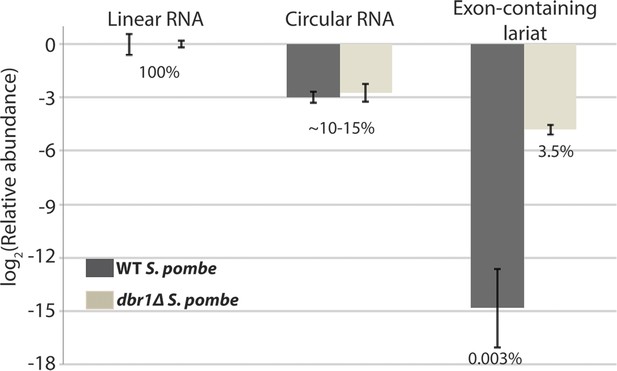
qPCR analysis of mrps16 splice isoforms.
The relative proportions of the species computed by subtracting mean Ct values from mean Ct values of the linear RNA. Error bars represent standard deviations from replicate experiments. Percentages are computed assuming perfect qPCR primer efficiency for all primers and are noted beneath the bars.
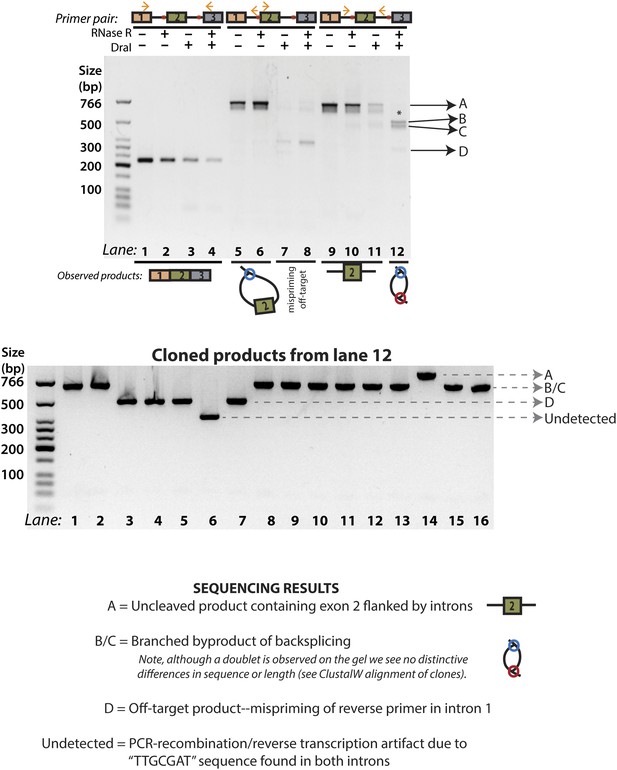
Figure 2D, Lane 12 cloning and sequencing results.
The products from Figure 2D, Lane 12 (reproduced above) were cloned, and 16 colonies were chosen for PCR analysis. Four distinct groups of products were observed, labeled A, B/C, D, and ‘undetected’. All products were sequenced and the results of each group of bands are described here. Products B/C correspond to the ‘doublet’ product observed in lane 12; the alignment of these sequences is shown in Figure 3—figure supplement 3. ‘Undetected’ refers to a PCR product that is not visible in the gel representing the original PCR reaction.
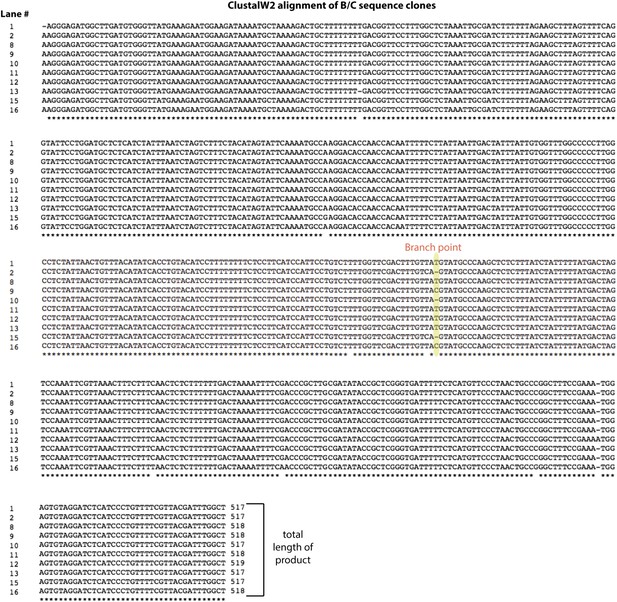
Alignment of backsplice byproduct PCR products.
The group of products labeled B/C in the lower gel in Figure 2—figure supplement 2 was sequenced and aligned using ClustalW2. The lane numbers to the left of the sequences correspond to the lower gel shown in Figure 2—figure supplement 2. The length of each product is shown at the end of each sequence.
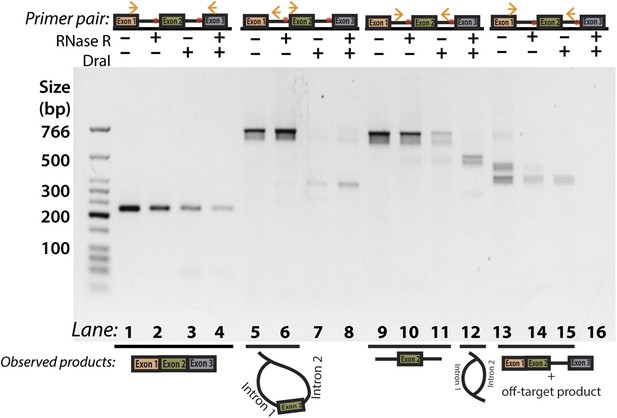
Additional DraI restriction digest PCR for Y-shaped intermediate product.
Panel 2D with additional lanes indicating attempted amplification of the Y-shaped intermediate. As in 2D, primers used are indicated above the gel and observed products are illustrated below. The off-target product is the higher molecular weight species (lane 13) and represents mispriming of the reverse primer to a downstream site in exon 3.
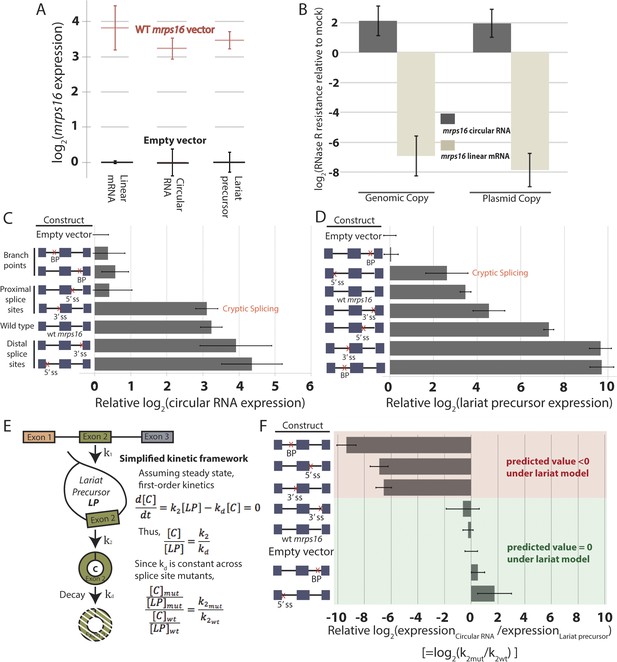
Quantitative analysis of mrps16 splice isoforms in dbr1∆ S. pombe reveals circular RNA can be produced through an exon-containing lariat precursor.
(A) qPCR quantification of splice isoforms produced by ectopically expressed mrps16 relative to background expression of each isoform. (B) qPCR quantification of RNase R resistance of the endogenously and ectopically expressed mrps16 circular and linear RNA isoforms. Positive values represent enrichment, while negative values represent depletion after RNase R treatment. (C–D) qPCR quantification of ectopically expressed mrps16 circular RNA (C) and lariat precursor RNA (D) for splice site and branch point mutants relative to background. Cryptic splicing of the 3′ ss in intron 1 generates a circular RNA whose junction differs by 5 nt, and cryptic splicing of the 5′ ss in intron 1 generates a larger lariat precursor using a 5′ ss positioned 74 nt upstream of the original splice site. (E) Simplified kinetic framework for the production and decay of circular RNA. Under this model, the values presented in (F) represent log2(k2mut/k2wt). (F) Ratio of circular to lariat precursor RNA for splice site and branch point mutants relative to background ratios. Red (predicted value < 0) and green (predicted value = 0) coloring represents predictions under the lariat model. These predictions neglect any effects due to cryptic splicing. In all cases, error bars represent standard deviations from replicate experiments.
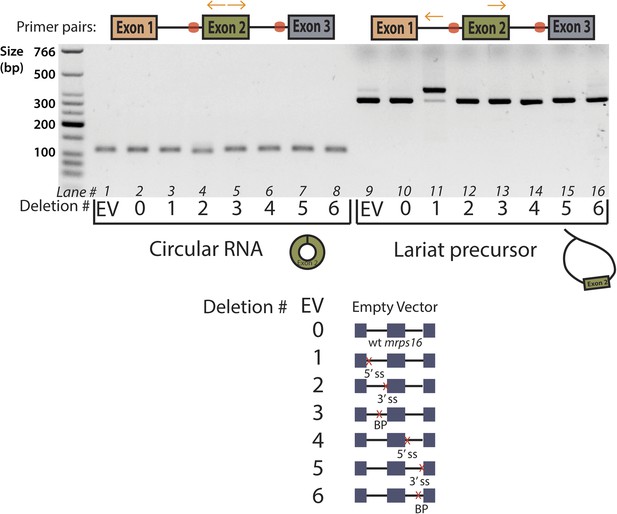
Agarose gel electrophoresis of mrps16 qPCR products.
Primers used are indicated above the gel, and the splice site mutants tested are indicated directly below the gel using a number key. Cryptic splicing of a large lariat precursor using a 5′ ss in exon 1 is responsible for the size shift observed in lane 11.
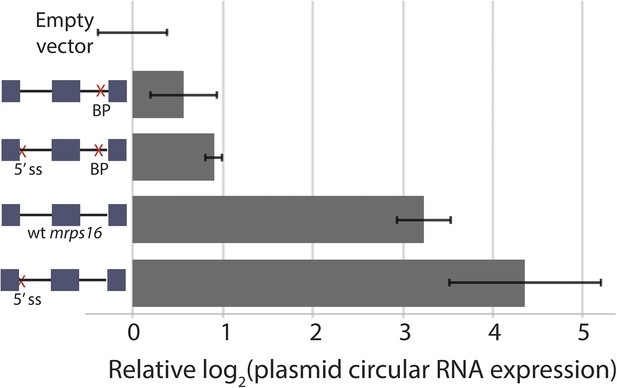
Effect of 5′ ss and BP double deletion on mrps16 circularization.
qPCR quantification of ectopically expressed mrps16 circular RNA. Splice site and branch point mutations are noted as in 3C. Values for EV, wt mrps16, and 5′ ss deletion are repeated here for reference. In all cases, error bars represent standard deviations from replicate experiments.
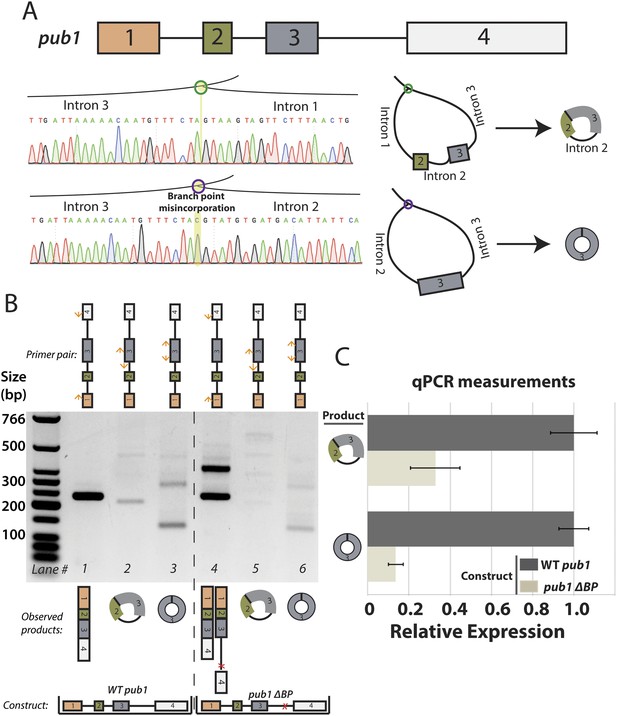
Analysis of S. pombe pub1 circular RNA splicing.
(A) Architecture of the pub1 gene along with sequencing traces for exon-skipped lariats. Traces were derived from Sanger sequencing of RT-PCR products from dbr1∆ S. pombe. (B) RT-PCR for pub1 circular and linear RNA. Isoforms of the pub1 (wt and ∆BP) are indicated below the gel. (C) qPCR quantification of circular RNA isoforms. Expressed form of pub1 is indicated on the bars, and circular isoform tested is shown to the left. Experiments shown in (B) and (C) were both performed in a pub1∆ background with pub1 expressed ectopically on a plasmid. Error bars represent standard deviations from technical replicates.
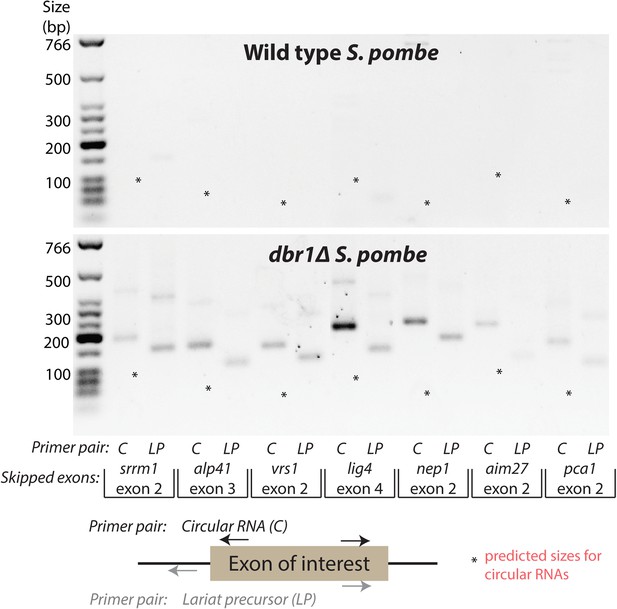
Production of exon-containing lariats is not sufficient to generate circular RNA.
RT-PCR analysis of a number of genes with validated exon-skipping events in S. pombe. The primers pairs used are indicated below the gel. Primer pair ‘C’ should amplify the circular RNA or the lariat precursor if the circle is absent, whereas primer pair ‘LP’ should specifically amplify the lariat precursor. For each gene, asterisks indicate the expected product size for the circular RNA. In each case, only exon-containing lariats from the exon-skipping events could be detected.
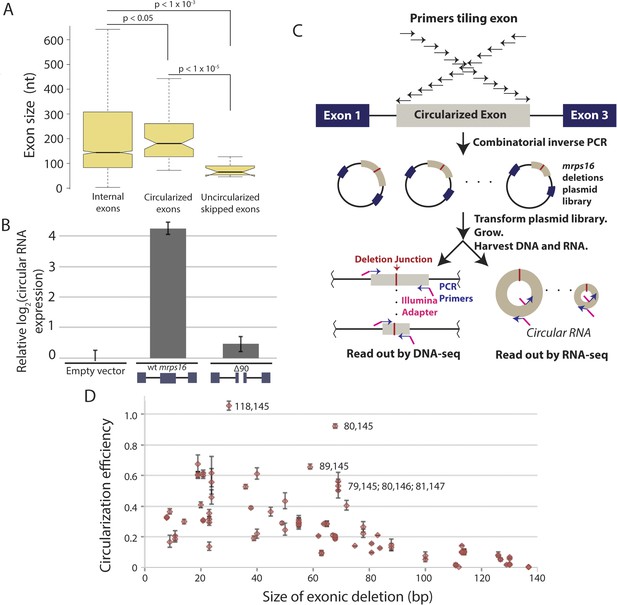
Exon size predicts circular RNA production.
(A) Notched box plots depicting the sizes of internal exons (n = 2831), single exon circular RNAs predicted from RNA-seq (n = 86), and skipped uncircularized exons (n = 11) in S. pombe. The notches represent the 95% confidence interval of the median (specifically, ± 1.57 × IQR/√n, where IQR is interquartile range, and n is sample size). p-values, denoted above the plots, were calculated using a Wilcoxon rank sum test. Note only single exon circular RNAs were included in this analysis. (B) qPCR analysis of circular RNA production from an mrps16 isoform lacking 90 nucleotides from the center of its second exon (mrps16 ∆90). Total circular RNA was measured using outward-facing primers outside the bounds of the deletion and normalized to total circular RNA produced by the endogenous locus. Error bars represent standard deviations from replicate experiments. (C) Schematic outlining the production of mrps16 exon plasmid deletion library and quantitative RNA-seq (qRNA-seq) method for determining isoform-specific circular RNA expression. (D) Circularization efficiency (CE) vs exon length as determined by qRNA-seq experiment. Outliers are noted on the plot using a parenthetical notation (x, y), where x and y represent the first and last nucleotide of deletion, respectively, with 1 representing the first nucleotide of the exon. Error bars represent two standard deviations from the mean (see ‘Materials and methods’ for derivation). Experiments shown in (B) and (D) were performed in wild-type S. pombe.
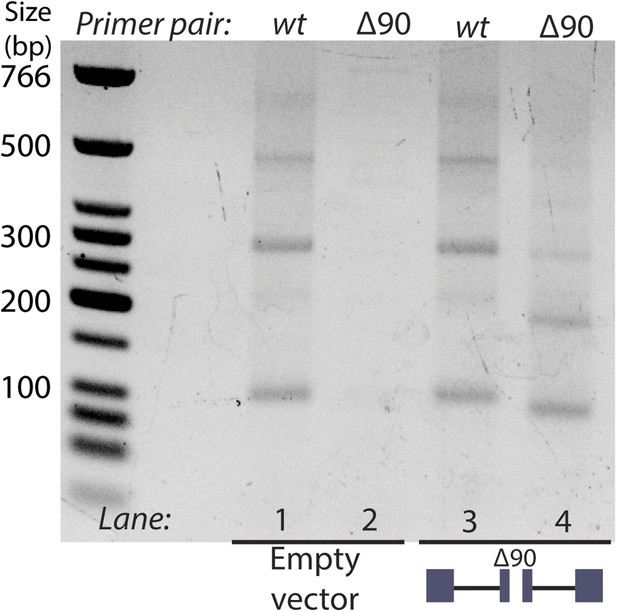
RT-PCR analysis of circular RNA produced by endogenous mrps16 and mrps16 ∆90.
Primer pairs are indicated above the gel. Primer pair wt is a set of primers specific to the wt mrps16 circular RNA, while primer pair ∆90 is specific to the deleted form of the exon. Expressed form of mrps16 is shown below the gel and is expressed in addition to the endogenous copy.
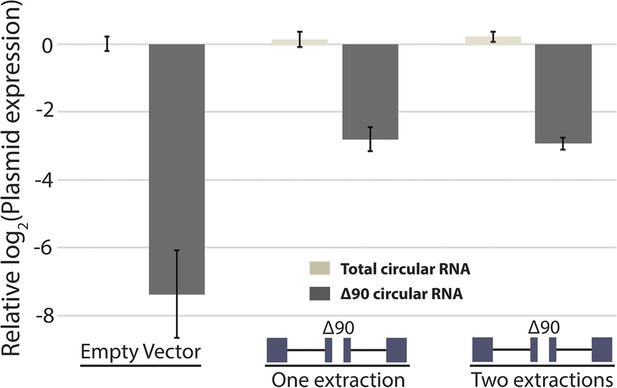
Measurement of RNA extraction size bias by qPCR.
qPCR analysis of circular RNA production from mrps16 ∆90. Total circular RNA was measured using outward-facing primers outside the bounds of the deletion, while ∆90 circular RNA was measured using primers specific to the deletion isoform (see Figure 5—figure supplement 1). All bars are normalized to total circular RNA produced by endogenous circular RNA (first data point; empty vector). Error bars represent standard deviations from technical replicates.
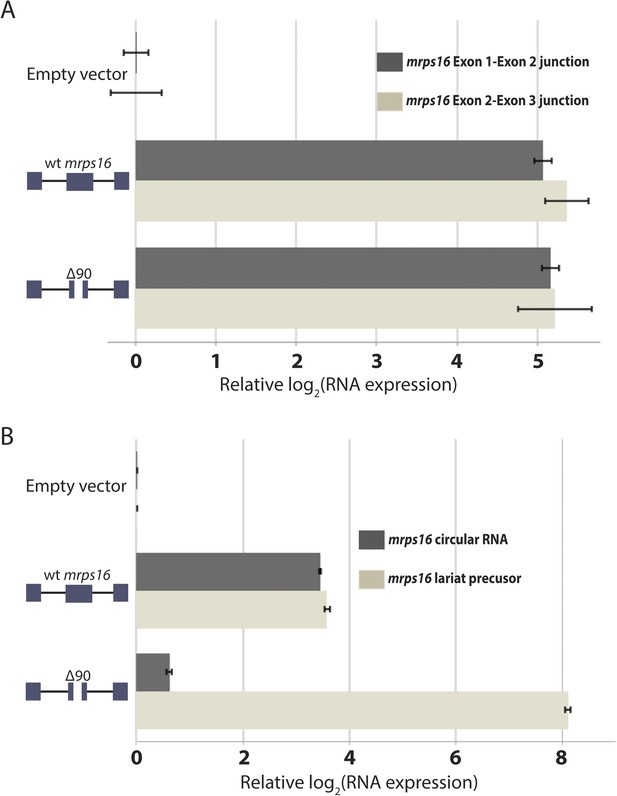
qPCR analysis of splice isoforms in mrps16 ∆90.
(A) qPCR quantification of mrps16 linear junctions, measured for both wt mrps16 and mrps16 ∆90 in wild-type S. pombe. (B) qPCR quantification of mrps16 circular RNA and lariat precursor in dbr1∆ S. pombe. Error bars represent standard deviations from technical replicates.
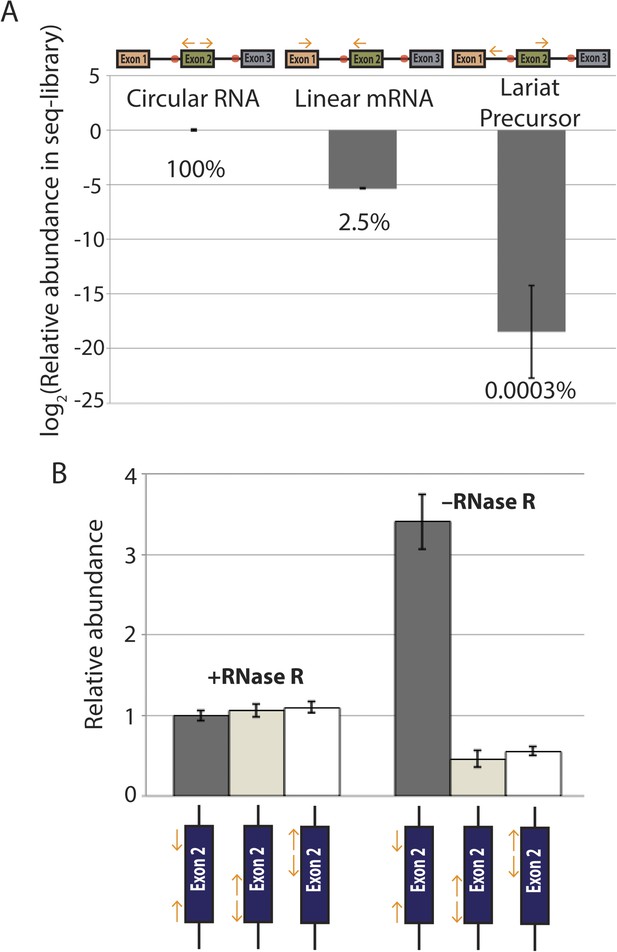
qPCR analysis of splice isoform representation in exonic deletion library.
(A) qPCR quantification of circular, linear, and lariat precursor abundance in exonic deletion library cDNA. Primers indicated above plot and relative contribution of each species to the library are shown as percentages below each bar. (B) Quantification of circular RNA biogenesis from a wt mrps16 plasmid with three different primer pairs, each of which was used to construct a sequencing library. In the absence of RNase R, inward-facing primers lead to much higher levels of PCR amplification due to amplification from linear RNA. In contrast, with RNase R, the three primer pairs give essentially identical Ct values, indicating that the linear RNA is not largely contributing. Note, we chose not to perform this experiment with our actual exonic deletion library, as variations in Ct values between the libraries will likely only reflect variability in the deletion isoforms detected and the expression levels of those isoforms, rather than contributions of various splice isoforms under the given treatment.
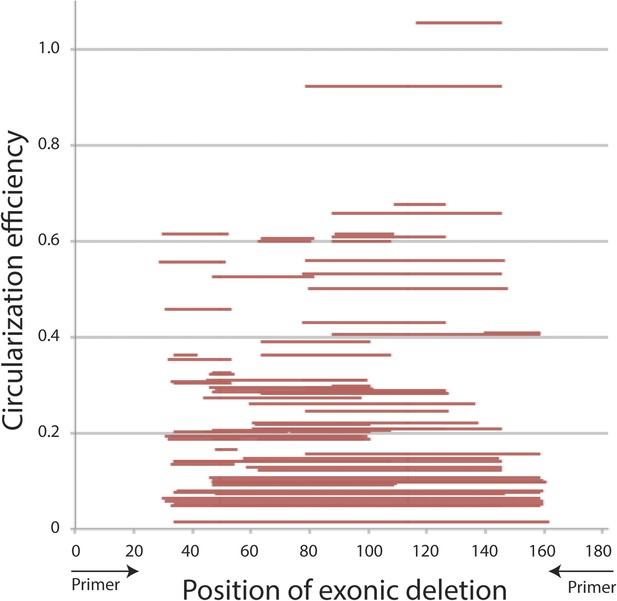
Primary sequence analysis of mrps16 exonic deletion library.
CE is plotted with respect to the position of each exonic deletion, depicted as horizontal bars extending from the start to end of the deletion.
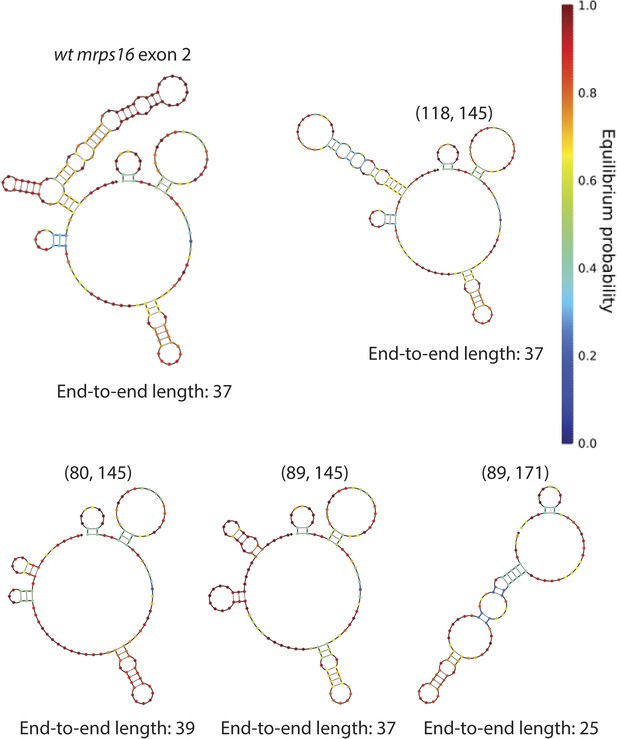
mrps16 exon 2 predicted structures.
Structures represent the predicted MFE secondary structures at 30°C. Deletion isoform is indicated above each structure along with its end-to-end length. These structures were predicted using the NUPACK web server. Parenthetical notation as in Figure 5D.
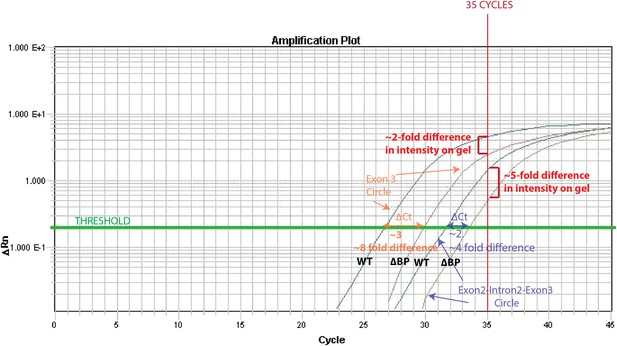
Additional files
-
Supplementary file 1
List of primers used for cloning in this study.
- https://doi.org/10.7554/eLife.07540.023