Frequency-selective control of cortical and subcortical networks by central thalamus
Figures
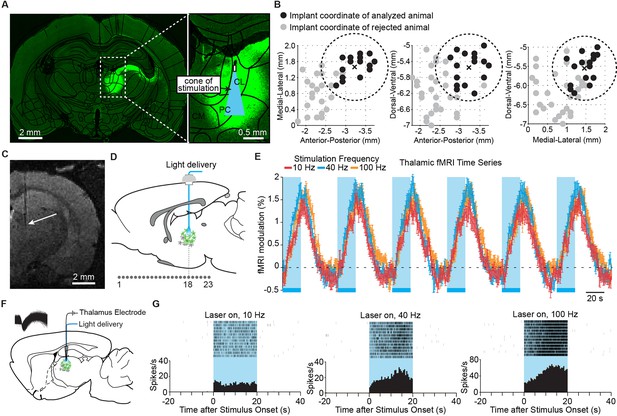
Targeted stimulation of central thalamus evokes positive BOLD changes and increases in neuronal firing at the site of stimulation.
(A) Representative wide-field fluorescence image shows robust ChR2-EYFP expression throughout central thalamus, overlaid with the estimated cone of excited tissue shown to scale. (B) Empirically observed locations of fiber optic implants in initial cohort of 47 rats, estimated using high-resolution structural MRI scans. Of these animals, 18 had implant locations that were accurately localized to the central thalamus (<0.85 mm from target site, shown as dashed circle and cross). Two were excluded based on lack of thalamic activation, leaving n = 16 rats for further analysis. Black dots indicate implant coordinates of 16 animals used for analysis. Gray dots indicate implant coordinates of 31 rejected animals. (C) Representative T2-weighted anatomical MRI scan used to estimate implant location, marked with arrow. (D) Schematic of 23 coronal slices acquired during ofMRI experiments. Slice numbers correspond to activation maps in Figure 2. (E) Average time series of significantly modulated voxels within the ipsilateral thalamus ROI (see Figure 2D) exhibit robust positive BOLD responses during repeated 20 s periods of stimulation at 10, 40, and 100 Hz, indicated by blue bars. Values are mean ± s.e.m. across animals (n = 16, 10, and 16 for each frequency, respectively). (F) Diagram of local in vivo optrode recordings during optical stimulation of central thalamus. Inset shows spike waveforms of a recorded neuron. (G) Representative peri-event time histogram of a recorded neuron showing the increase in firing rate within central thalamus during optical stimulation at each of the three frequencies tested. See also Figure 1—figure supplement 1 and Figure 1—source data 1. BOLD: Blood-oxygen-level-dependent; ROI: Regions of interest.
-
Figure 1—source data 1
Firing rates before, during, and after repeated 20 s stimulation periods for each of the five neurons recorded in central thalamus.
Exact p values comparing thalamic firing rate before and during stimulation are provided.
- https://doi.org/10.7554/eLife.09215.004
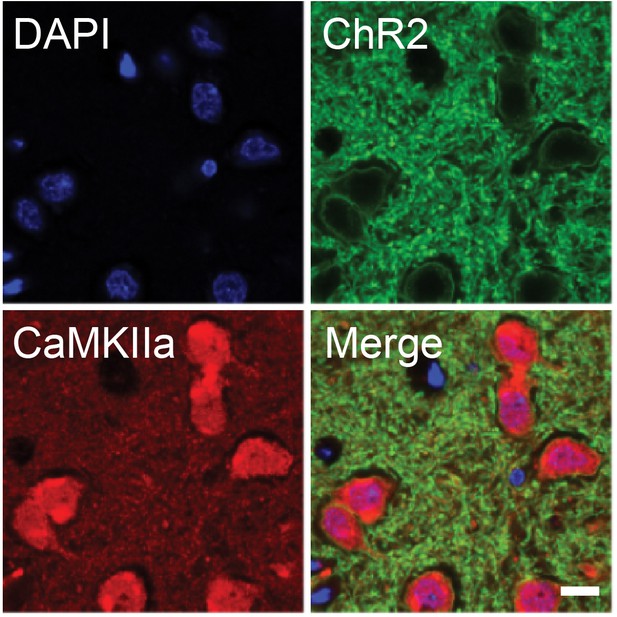
Specificity of ChR2 targeting for CaMKIIa-positive cells.
Immunohistochemistry confirms the specific targeting of ChR2-EYFP to CaMKIIa-positive neurons in central thalamus. 35% of cells identified within the bulk injection area were EYFP-positive, and 97% of EYFP-positive cells co-expressed CaMKIIa (n = 2 rats, 831 cells). Scale bar, 10 µm.
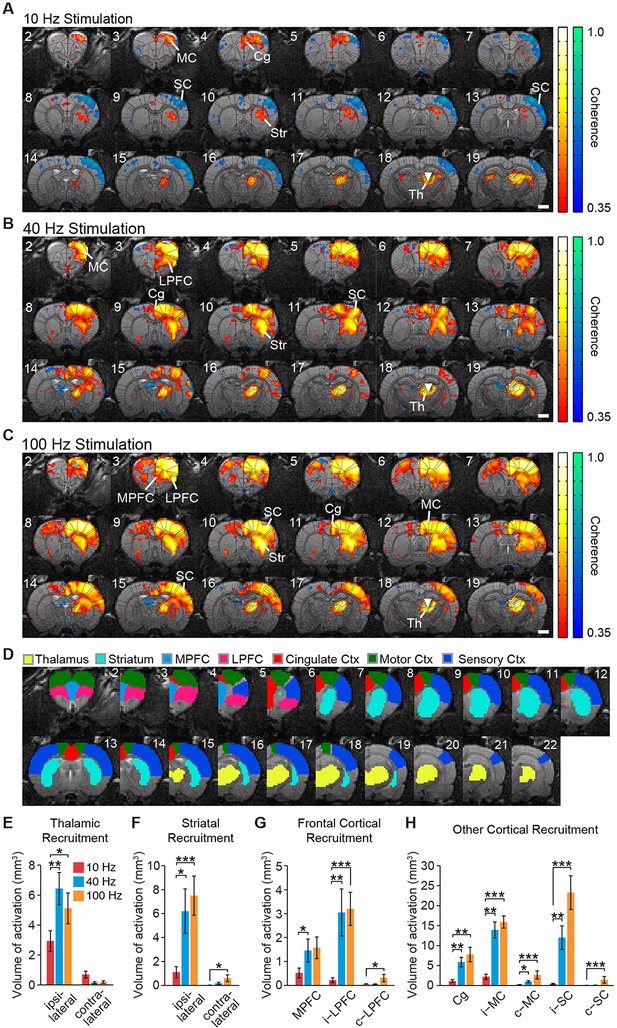
Spatial characterization of evoked fMRI signals.
(A-–C) Average coherence maps of brain-wide activity during stimulation of excitatory central thalamus relay neurons at 10, 40, and 100 Hz. Warm colors indicate positive BOLD responses, while cool colors indicate negative BOLD responses (see ‘Materials and methods’). (D) Regions of interest (ROIs) used for quantitative analysis of spatial ofMRI activation patterns. (E) The amount of active volume (positive signal with coherence > 0.35) in the ipsilateral thalamus is significantly greater during 40 and 100 Hz stimulations than 10 Hz stimulation. Thalamic recruitment is relatively limited on the contralateral side. (F) Activation of the ipsilateral striatum is significantly greater during 40 and 100 Hz stimulations than 10 Hz stimulation. Activation of the contralateral striatum is limited across frequencies, although there is an increase from 10 to 100 Hz. (G) Medial and lateral prefrontal cortex exhibit a significantly greater volume of activation during 40 and/or 100 Hz stimulation than 10 Hz stimulation. Activity in the contralateral hemisphere is limited across all tested frequencies, although there is an increase from 10 to 100 Hz. (H) Activation of cingulate, motor, and somatosensory cortex is each greater during 40 and 100 Hz stimulations than 10 Hz stimulation. The contralateral motor and sensory cortices are also activated to a greater extent during 40 and/or 100 Hz stimulation. Scale bars in panels A through C represent 2 mm. Asterisks in panels E through H indicate significant differences for 10 versus 40 Hz and 10 versus 100 Hz stimulations. *p < 0.05, **p < 0.005, ***p < 0.001, one-sided Wilcoxon signed-rank tests, corrected for multiple comparisons. Error bars represent mean ± s.e.m. across animals. n = 16, 10, and 16 animals for 10, 40, and 100 Hz, respectively. Abbreviations are as follows: i- (ipsilateral), c- (contralateral), Cg (cingulate cortex), MC (motor cortex), MPFC (medial prefrontal cortex), LPFC (lateral prefrontal cortex), SC (sensory cortex), Str (striatum), Th (thalamus). See also Figure 2—figure supplements 1/2/3 and Figure 2—source data 1.
-
Figure 2—source data 1
Animal-specific activation volumes for the twelve regions of interest shown in Figure 2E–H.
Exact p values comparing the volume activated during 10 versus 40 Hz stimulation and 10 versus 100 Hz stimulation are provided.
- https://doi.org/10.7554/eLife.09215.007
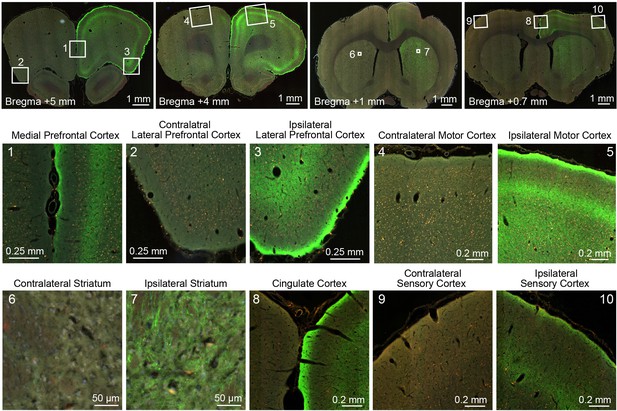
Representative fluorescence images of ChR2-EYFP at remote targets illustrate the massive projections to forebrain from transfected relay neurons in the right central thalamus.
The bottom two rows provide magnified images of cortical and striatal regions used for quantitative ofMRI analysis. The top row provides the whole-brain slices from which these magnified images come. EYFP-expressing axonal projections are primarily localized to the ipsilateral hemisphere and to superficial layers in cortex.
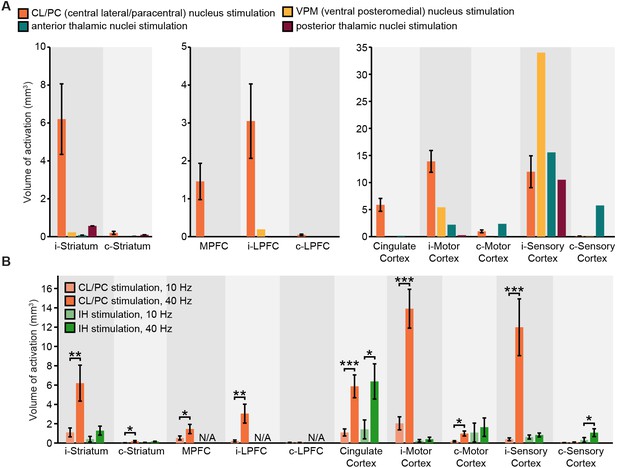
Widespread and frequency-dependent recruitment of forebrain with optogenetics is distinct to stimulation of intralaminar nuclei of central thalamus.
(A) Volumes of striatal and cortical activation (i.e. positive BOLD signals with coherence greater than 0.35) during 40 Hz stimulation of central thalamus, presented with activation volumes during stimulation of other thalamic nuclei. Central thalamus is the only target to result in significant recruitment of striatum and prefrontal and frontal cortical regions. (B) Comparison of frequency dependent effects of central thalamus stimulation with those of intermediate hippocampus (IH) stimulation. Unlike central thalamus stimulation, which recruits significantly more volume in striatum, motor cortex, and sensory cortex at 40 Hz than at 10 Hz (*p < 0.05, **p< 0.005, ***p < 0.001; one-sided Wilcoxon signed-rank test), recruitment of these regions during hippocampal stimulation does not exhibit a significant dependence on frequency. Note that activation data was not available (N/A) at MPFC or LPFC for hippocampus stimulation due to differences in field of view. Abbreviations are as follows: i- (ipsilateral), c- (contralateral), MPFC (medial prefrontal cortex), LPFC (lateral prefrontal cortex).
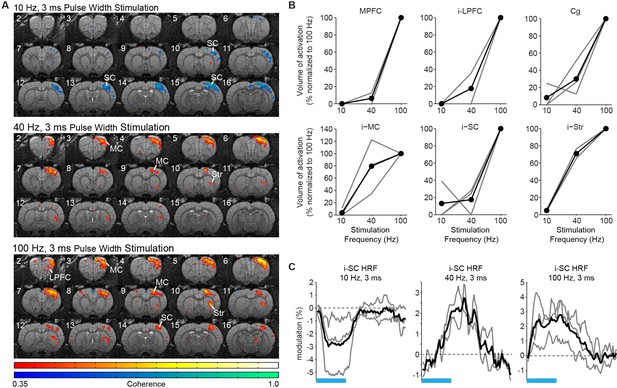
The frequency-dependent recruitment of forebrain by central thalamus and its control over cortical BOLD signal polarity are preserved when pulse width is held constant.
(A) Representative coherence map of brain-wide activity during stimulation of excitatory central thalamus relay neurons at 10, 40, and 100 Hz using a constant pulse width of 3 ms. Warm colors indicate positive BOLD responses, while cool colors indicate negative BOLD responses (see ‘Materials and methods’). (B) Quantification of positive BOLD responses in cortex and striatum (n = 3 animals). Gray lines indicate animal-specific results, normalized to 100 Hz stimulation. Black lines indicate the average across animals. All six regions exhibit an increase in recruitment with frequency, consistent with the study’s main results when pulse width was varied to keep the duty cycle and total amount of light delivery constant. Regions of interest are the same as those used in Figure 2. (C) Hemodynamic response functions evoked in somatosensory cortex during 10, 40, and 100 Hz stimulation of central thalamus using a constant pulse width of 3 ms. Consistent with the study’s main results, a negative BOLD signal is evoked at 10 Hz, while slow and fast positive BOLD responses are evoked at 40 and 100 Hz, respectively. Blood-oxygen-level-dependent.
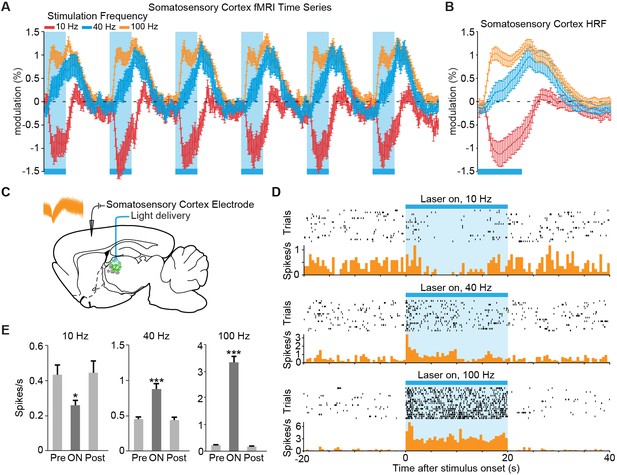
The sign of evoked cortical activity depends on the frequency of central thalamic stimulation.
(A,B) 10 Hz stimulation of central thalamus evokes a strong negative BOLD signal throughout ipsilateral somatosensory cortex, while 40 and 100 Hz stimulations evoke positive responses. Time series come from the sensory cortex ROI defined in Figure 2D. Hemodynamic response function (HRF) shows the average response to a single 20 s period of stimulation, indicated by blue bar. Error bars represent mean ± s.e.m. across animals. n = 16, 10, and 16 for 10, 40, and 100 Hz, respectively. (C) Diagram of in vivo recordings at somatosensory cortex during stimulation of excitatory central thalamus relay neurons. Inset shows spike waveforms of a recorded neuron. (D,E) Representative peri-event time histogram of a recorded neuron, and corresponding quantification of firing rate during the 20 s periods before, during, and after stimulation. Neural firing rate decreased within the somatosensory cortex during 10 Hz central thalamus stimulation, but increased during 40 and 100 Hz stimulations (n = 17 trials each, *p < 0.05, ***p < 0.001 pre vs. ON, two-tailed Wilcoxon signed-rank test; see Table 1 for further analysis). Values are mean ± s.e.m. See also Figure 2—figure supplement 3C and Figure 3—figure supplement 1.
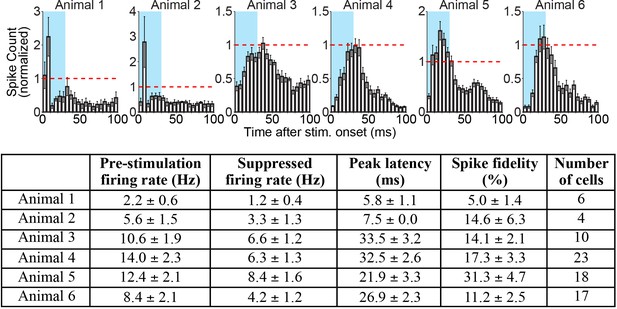
Cortical spikes that occur during periods of inhibition driven by 10 Hz central thalamus stimulation exhibit a non-uniform distribution over time.
(Top figure) Average peristimulus time histograms (PSTHs) of spike events in somatosensory cortex during 10 Hz central thalamus stimulation for six animals. Analysis was restricted to the 5 s time bin with the greatest number of neurons inhibited for each animal. PSTHs were calculated by aligning spike counts to the onset of individual 30 ms light pulses, summing over the 50 pulses delivered during the 5 s stimulation period, averaging across trials, and binning at 5 ms intervals for each inhibited neuron. Histograms were normalized by the corresponding spike count value during the 20 s pre-stimulation baseline period (represented by the dashed red line), and averaged across neurons for each animal. Blue rectangles represent the 30 ms light pulse. Note that spike events are reduced relative to baseline for the majority of the 100 ms inter-stimulus period, but spike events that do occur have a non-uniform distribution that peaks 6–34 ms after stimulus onset. These patterns suggest that some thalamic stimuli induce spike events in cortex, despite the net suppression of activity relative to pre-stimulation levels. Animals presented include two used for ChR2-electrophysiology experiments in Table 1 and four used for combined ChR2-eNpHR electrophysiology experiments in Figure 5. (Bottom table) Summary of PSTH peak latencies and spike fidelity for inhibited neurons in somatosensory cortex. Peak latency was defined as the 5 ms bin with highest spike count for each neuron’s PSTH. Spike fidelity represents the percentage of light pulses in the given 5 s bin of inhibition that evoke at least one spike during the 30 ms pulse. Values represent mean +/- s.t.e. across cells in the figure and table.
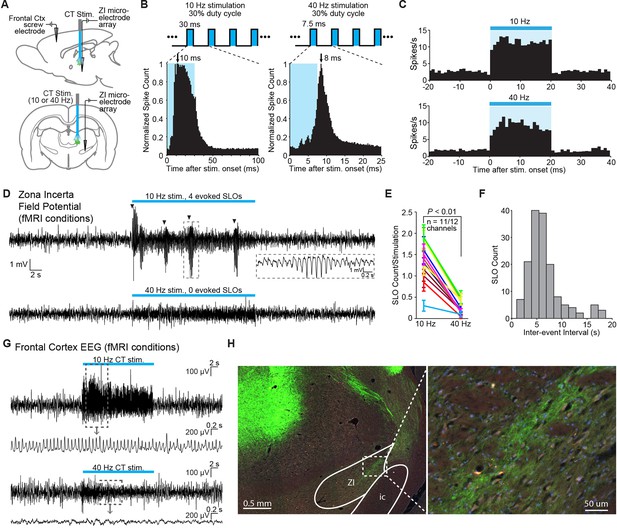
Frequency-dependent spindle-like oscillations are evoked in zona incerta (ZI).
(A) Diagram of in vivo recordings at ZI and simultaneous EEG recordings in frontal cortex during optical stimulation of central thalamus in anesthetized animals. (B) Representative peri-event time histograms of spiking activity from recorded ZI neurons aligned to the onset of individual light pulses, summed over all pulses and trials. Peak spike latencies are approximately 10 and 8 ms for 10 Hz (left) and 40 Hz (right) stimulations, suggesting that recordings are performed at least one synapse away from the stimulated population in central thalamus. Schematics at top illustrate the 30% duty cycle pulse trains which lasted 20 s for each frequency. (C) Representative peri-event time histograms over the 20 s period of stimulation show increases in ZI firing during 10 and 40 Hz stimulations. Among the 28 isolated single-units in ZI (n = 2 animals), most exhibited a significant increase in firing rate during stimulation (n = 26 and 22 out of 28 neurons, respectively; p < 0.05, one-tailed Wilcoxon signed-rank test with 20 trials for each cell). (D) Representative field potential recordings from the same channel and trial number during 10 Hz (top) and 40 Hz (bottom) stimulation of central thalamus. Four amplitude-modulated, spindle-like oscillations (SLOs) are evoked during 10 Hz stimulation (marked by black triangles), while none are evoked during 40 Hz stimulation. Inset shows a zoomed-in SLO. (E) The number of SLOs was greater during 10 Hz stimulation than 40 Hz stimulation across 11 of 12 available channels (n = 2 animals, 20 trials each, p < 0.01, one-tailed Wilcoxon rank sum test). (F) When more than one SLO was evoked within the same 20 s period of 10 Hz stimulation, the distribution of inter-event intervals was centered at 6.6 ± 0.2 s (s.e.m.). (G) Representative EEG recordings collected in frontal cortex during central thalamus stimulation and simultaneous ZI recordings. 10 Hz stimulation evokes a spike-wave response, which is associated with loss of consciousness and perceptual awareness, while 40 Hz stimulation evokes a low voltage fast response indicative of arousal. (H) ChR2-positive processes were observed in ZI, providing a basis for its recruitment during stimulation of central thalamus. i.c.: internal capsule. See also Figure 4—source data 1, 2. EEG: Electroencephalography.
-
Figure 4—source data 1
Firing rates before, during, and after repeated 20 s central thalamus stimulation periods for each of the 28 neurons recorded in zona incerta.
Exact p values comparing zona incerta firing rate before and during central thalamus stimulation are provided.
- https://doi.org/10.7554/eLife.09215.019
-
Figure 4—source data 2
Quantification of spindle-like oscillation (SLO) occurrences in the zona incerta during 10 and 40 Hz stimulation of central thalamus.
Exact p values comparing SLO counts during 10 versus 40 Hz stimulation are provided.
- https://doi.org/10.7554/eLife.09215.020
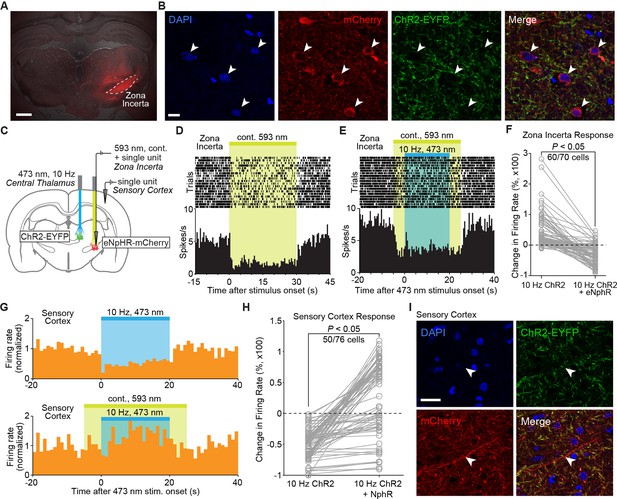
Cortical inhibition driven by 10 Hz central thalamus stimulation depends on normal incertal processing.
(A) Wide-field fluorescence image shows robust eNpHR-mCherry expression spatially localized to the right zona incerta. Scale bar, 1 mm. (B) Confocal images show eNpHR-mCherry localized to somatic membrane of neurons in zona incerta. Scale bar, 10 µm. Two hundred and nine out of 882 DAPI-stained cells co-expressed mCherry in ZI (24%, n = 2 animals). (C) Schematic of cortical electrophysiology recordings during 10 Hz stimulation of central thalamus and continuous (cont.) inhibition of zona incerta using ChR2 and eNpHR, respectively. (D) Peri-event time histogram of a representative neuron in zona incerta whose firing rate is suppressed during activation of eNpHR with 593 nm light. (E) Peri-event time histogram of a representative neuron in zona incerta whose firing rate remains suppressed throughout the period of 10 Hz central thalamus stimulation during eNpHR activation (compare to Figure 4C). (F) Activation of eNpHR in zona incerta significantly reduces the change in incertal firing rate evoked by 10 Hz central thalamus stimulation in 60 of 70 neurons (p < 0.05, one-sided Wilcoxon rank sum test). Changes in firing rate are normalized to pre-stimulation levels. (G) Peri-event time histograms from a representative cortical neuron show that the inhibitory response evoked by 10 Hz central thalamus stimulation is reversed by simultaneously suppressing activity in zona incerta. Firing rates are normalized to the average pre-stimulation values. (H) Quantification of evoked changes in cortical firing rate during 10 Hz central thalamus stimulation with and without concurrent eNpHR activation. 50 out of 76 cells exhibit reduced inhibition when central thalamus stimulation is paired with eNpHR activation (p < 0.05, Wilcoxon rank sum test over 1 s bins). Changes in firing rate are normalized to pre-stimulation levels. (I) Confocal images show mCherry-positive axonal projections from zona incerta in somatosensory cortex. Scale bar, 20 µm. See also Figure 5—source data 1,2.
-
Figure 5—source data 1
Evoked changes in incertal firing rate during 10 Hz central thalamus stimulation with and without concurrent eNpHR activation.
Changes are normalized to pre-stimulation baseline levels. Exact p values are provided.
- https://doi.org/10.7554/eLife.09215.022
-
Figure 5—source data 2
Evoked changes in cortical firing rate during 10 Hz central thalamus stimulation with and without concurrent eNpHR activation in zona incerta.
Values represent the 1 s bin that exhibited the greatest number of cells with reduced inhibition for each animal. Changes are normalized to pre-stimulation baseline levels. Exact p values are provided.
- https://doi.org/10.7554/eLife.09215.023
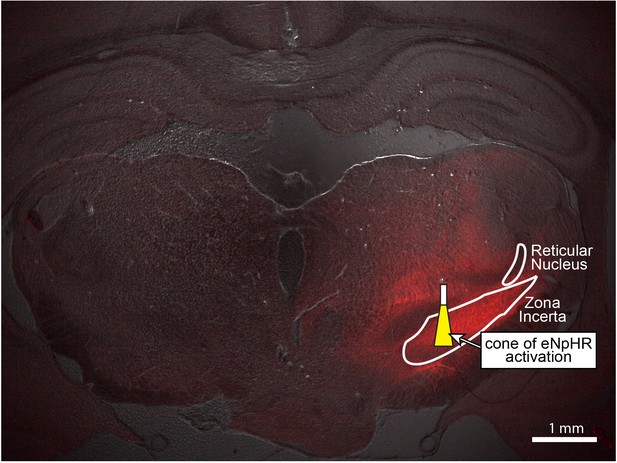
Wide-field fluorescence image of eNpHR expression in zona incerta, overlaid with the estimated cone of activated eNpHR (i.e. inhibited neurons) shown to scale.
Penetration depth and volume were calculated to be 0.64 mm and 0.024 mm3, respectively, using the methods described in (Aravanis et al., 2007) and a threshold light intensity of 5 mW/mm2 (Mattis et al., 2012). The optical fiber had a diameter of 105 μm, NA of 0.22, and half-angle of divergence of 9.3°. Penetration depth and activation volume correspond to an optical power of 3 mW exiting the fiber optic’s tip. Stimulation coordinate corresponds to -3.96 mm AP, +2.4 mm ML, and -6.7 mm DV. The thalamic reticular nucleus, another region of dense GABAergic neurons, is shown for reference.
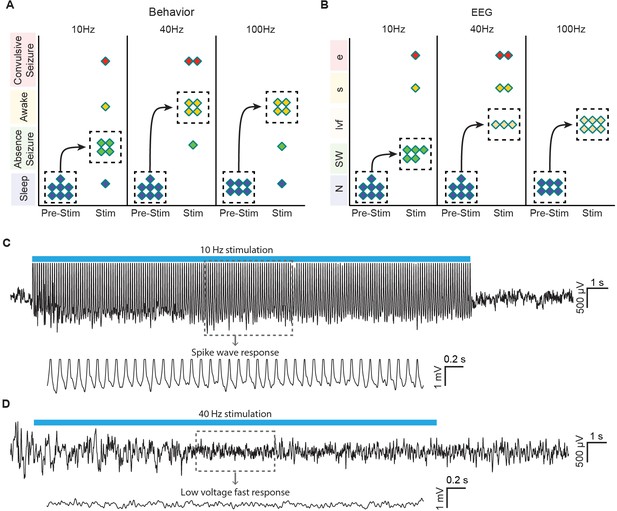
Optogenetic stimulation of central thalamus in asleep animals modulates brain state in a frequency-dependent manner.
(A) Low-frequency stimulation (10 Hz) in a majority of animals (n = 4/7) evokes behavioral absence seizures, while high-frequency stimulations (40 and 100 Hz) cause a majority of animals to awaken (n = 4/7 and 4/6, respectively). Dashed boxes indicate the most common response for each frequency, with arrows indicating the corresponding transition from sleep. (B) Low-frequency stimulation typically evokes spike-wave responses in EEG (n = 5/7), consistent with the behavioral reading of absence seizures. The most frequent EEG response during high-frequency stimulations is low voltage fast (n = 3/7 and 6/6), indicative of arousal. N, normal. SW, spike-wave. lvf, low voltage fast. s, spiking. e, evolving seizure. (C,D) Representative traces of EEG responses classified as spike-wave and low voltage fast. Insets show 4 s magnification. Importantly, these EEG patterns match those recorded under anesthetized conditions (Figure 4G), further linking the responses visualized with ofMRI to the reported behavioral responses. See also Figure 6—figure supplement 1. EEG: Electroencephalography.
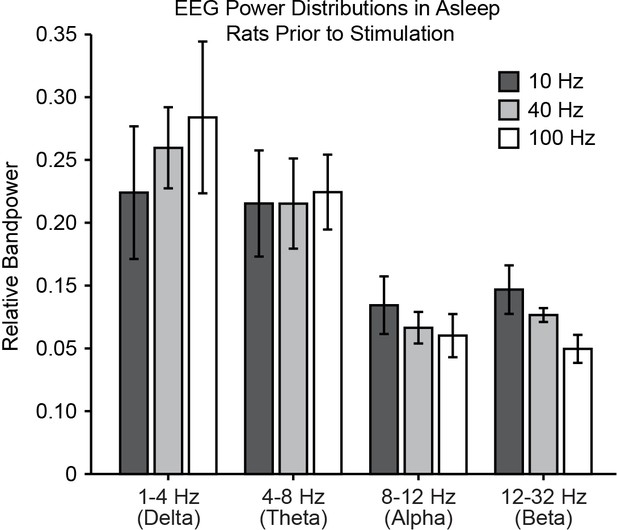
Pre-stimulus activity is consistent across frequencies of stimulation in asleep rats, as quantified with EEG bandpower in delta, theta, alpha, and beta bands. EEG: Electroencephalography.
https://doi.org/10.7554/eLife.09215.026
Videos
Spatiotemporal dynamics of ofMRI activity during 10 Hz stimulation of excitatory relay neurons of the central thalamus.
Highlighted voxels are restricted to those significantly synchronized to the block stimulation paradigm, as determined by frequency domain analysis. Color coding reflects the instantaneous relative percent modulation of each voxel’s hemodynamic response function, thresholded over ± (0.2 to 1.5)%. Laser status indicates the 20 s period of stimulation (2–22 s). Abbreviations are as follows: SC (sensory cortex), Th (thalamus).
Spatiotemporal dynamics of ofMRI activity during 40 Hz stimulation of excitatory relay neurons of the central thalamus.
Highlighted voxels are restricted to those significantly synchronized to the block stimulation paradigm, as determined by frequency domain analysis. Color coding reflects the instantaneous relative percent modulation of each voxel’s hemodynamic response function, thresholded over ± (0.2 to 1.5)%. Laser status indicates the 20 s period of stimulation (2–22 s). Abbreviations are as follows: Cg (cingulate cortex), LPFC (lateral prefrontal cortex), MC (motor cortex), MPFC (medial prefrontal cortex), SC (sensory cortex), Str (striatum), Th (thalamus).
Spatiotemporal dynamics of ofMRI activity during 100 Hz stimulation of excitatory relay neurons of the central thalamus.
Highlighted voxels are restricted to those significantly synchronized to the block stimulation paradigm, as determined by frequency domain analysis. Color coding reflects the instantaneous relative percent modulation of each voxel’s hemodynamic response function, thresholded over ± (0.2 to 1.5)%. Laser status indicates the 20 s period of stimulation (2–22 s). Abbreviations are as follows: Cg (cingulate cortex), LPFC (lateral prefrontal cortex), MC (motor cortex), MPFC (medial prefrontal cortex), SC (sensory cortex), Str (striatum), Th (thalamus).
Tables
Electrophysiology results from sensory cortex single-unit recordings. See also Table 1—source data 1.
Stimulation frequency | Effect on sensory cortex firing rate | Percentage of neurons with significant change in firing rate (n = 11) | |||
---|---|---|---|---|---|
0–5 s after stim. onset | 5–10 s after stim. onset | 10–15 s after stim. onset | 15–20 s after stim. onset | ||
10 Hz | Increase | 0% | 0% | 0% | 0% |
Decrease | 0% | 91% | 82% | 9% | |
40 Hz | Increase | 100% | 91% | 36% | 55% |
Decrease | 0% | 0% | 0% | 0% | |
100 Hz | Increase | 100% | 82% | 82% | 82% |
Decrease | 0% | 0% | 0% | 0% |
-
Table 1—source data 1
Firing rates before, during, and after repeated 20 s stimulation periods for each of the 11 neurons recorded in somatosensory cortex.
Exact p values comparing the somatosensory cortex firing rate before and during stimulation are provided. The 20 s stimulation period was divided into four consecutive 5 s blocks to evaluate the change in firing rate over time.
- https://doi.org/10.7554/eLife.09215.017
Additional files
-
Source code 1
Matlab script (countSLOs.m) for identification and quantification of spindle-like oscillations.
- https://doi.org/10.7554/eLife.09215.027