The context-dependent epigenetic and organogenesis programs determine 3D vs. 2D cellular fitness of MYC-driven murine liver cancer cells
Figures
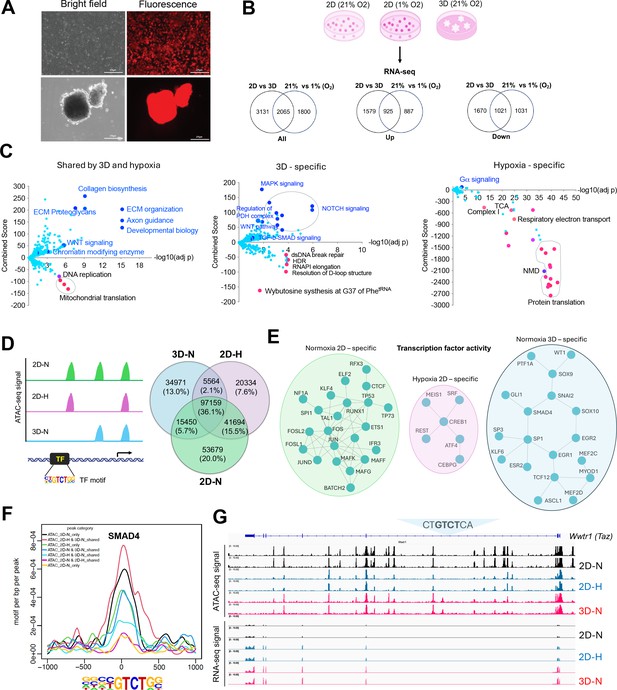
Transcriptomic and epigenetic reprogramming in hypoxic 2D and normoxic 3D conditions.
(A) The ABC-Myc-driven hepatoblastoma organoids serve as a good model for genome wide fitness screening. The ABC-Myc lineage was validated through expression of TdTomato. scale bar = 275µm (B) RNA-sequencing data from cells cultured in our conditions of interest are represented in Venn Diagrams of genes in 3D vs 2D normoxia and 2D-Hypoxia vs 2D-Normoxia (n=3 per group). The leftmost Venn diagram represents a total count of all genes identified, followed by the Up and down labeled diagrams reporting the upregulated and downregulated genes, respectively. The numbers in the Venn diagrams represent the number of significant genes expressed in the respective conditions. Created with BioRender. (C) The volcano plots, generated using Enrichr, show significant REACTOME pathways shared by cells in normoxic 3D and hypoxic 2D (left), specific to hypoxic 2D (middle) and normoxic 3D conditions (right; n=3 per group). The X- axis represents the significance of expression change for a gene in –log10 (adjusted p- value). The Y- axis represents the combined score for enrichment of a given pathway with positive values indicating enrichment and negative values indicating a decrease in the pathway. (D) ATAC-seq analysis for cells under normoxic 2D, hypoxic 2D, and normoxic 3D conditions (n=2 per group). The left cartoon indicates the changes of ATAC-seq signals under different culture conditions and predicted transcription factor binding motif. Right Venn diagram showing the common and unique ATAC-seq signals (numbers and percentages) under three conditions. (E) Protein-protein interaction network (STRING confidence threshold = 0.7) formed by transcription factors that are predicted to be highly active under normoxic 2D, hypoxic 2D, and normoxic 3D, respectively. (F) SMAD4 motif densities around ATAC-seq open chromatin regions (±1000 bp) by categories. Motif density was determined by HOMER (Hypergeometric Optimization of Motif EnRichment) program and normalized to that in a background sequence of equal length. X-axis indicates the distance to peak center (bp). (G) IGV snapshot shows the ATAC-seq signals and RNA-seq reads under normoxic 2D, hypoxic 2D, and normoxic 3D conditions. The ‘CTGTCTCA‘ SMAD4 binding motif lies within the ATAC-seq peak specifically appears in normoxic 3D conditions.
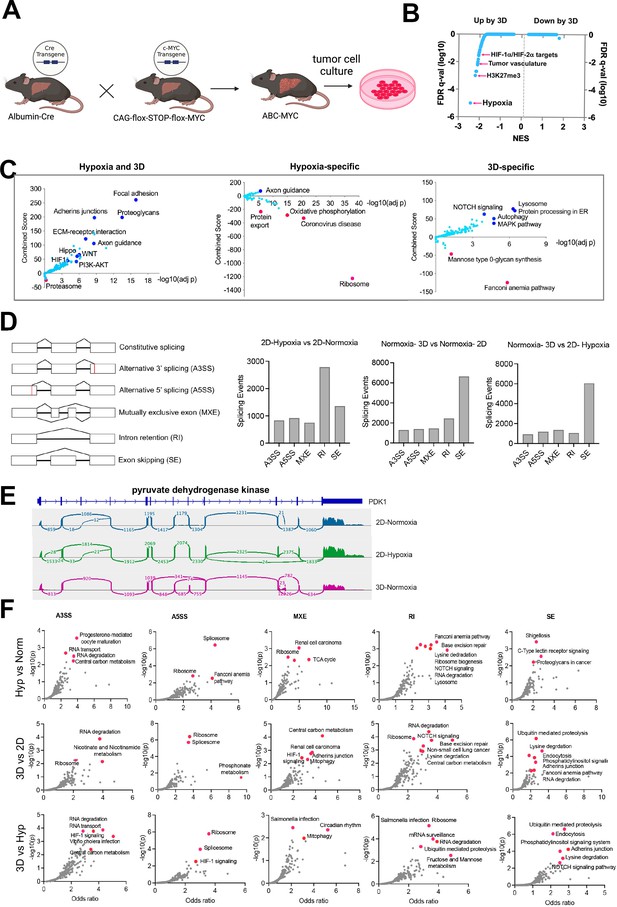
Cells undergo different transcriptional programing in hypoxia, normoxia, and 3D culture.
(A) Cartoon indicate the generation of ABC-Myc-driven hepatoblastoma model for in vitro tumor cell culture. Created with BioRender. (B) GSEA results for pathway enrichment in 3D vs 2D normoxia. Each dot represents one geneset. NES = normalized enrichment score. FDR = false discovery rate. (C) The volcano plots, generated using Enrichr, show significant KEGG pathways shared by cells in 3D and hypoxia (left), specific to hypoxic (middle) and 3D conditions (right). The X- axis represents the significance of expression change for a gene in –log10 (adjusted p- value). The Y- axis represents the combined score for enrichment of a given pathway with positive values indicating enrichment and negative values indicating a decrease in the pathway. (D) Five major alternative splicing events are illustrated with the boxes representing exons and the connecting lines representing introns. When comparing 2D hypoxia to 2D normoxia, there is high intron retention as indicated by the number of splicing events is over 2000. Normoxic 3D compared to 2D also shows an increase in intron retention along with upregulation of exon skipping. The graph representing 3D Normoxia and 2D Hypoxic shows both conditions upregulate exon skipping; however, increased intron retention is not seen. (E) The splicing events of pyruvate dehydrogenase kinase, a regulator of pyruvate dehydrogenase and known oncogenic driver, is shown in three conditions of interest: 2D normoxia, 2D hypoxia, and 3D normoxia. (F) Pathways for genes undergoing alternative splicing in each category. The Y- axis represents the significance of expression change for a gene in –log10 (adjusted p- value). The X- axis represents the Odds ratio.
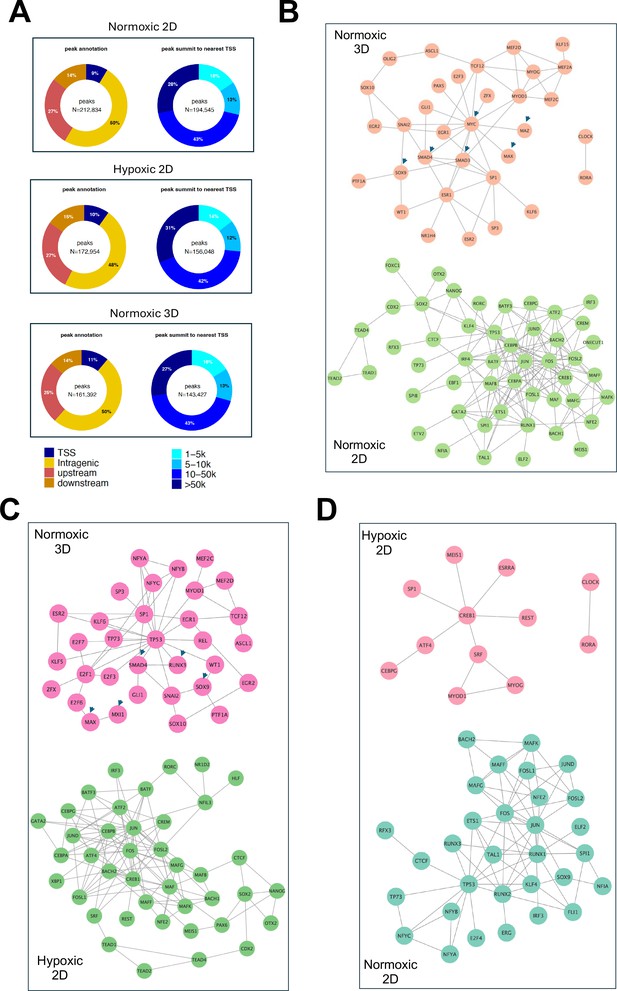
Epigenetic reprogramming under hypoxic 2D and normoxic 3D conditions.
(A) The percentage of ATAC-seq peaks at different genomic regions (transcription start site, intragenic, upstream of 5’ and downstream of 3’; left panel), and the percentage of peaks with distances from the transcription start site (TSS). (B–D) Pairwise comparison of the protein-protein interaction networks (STRING confidence threshold = 0.7) formed by transcription factors that are predicted to be highly active under normoxic 2D, hypoxic 2D, and normoxic 3D, respectively.
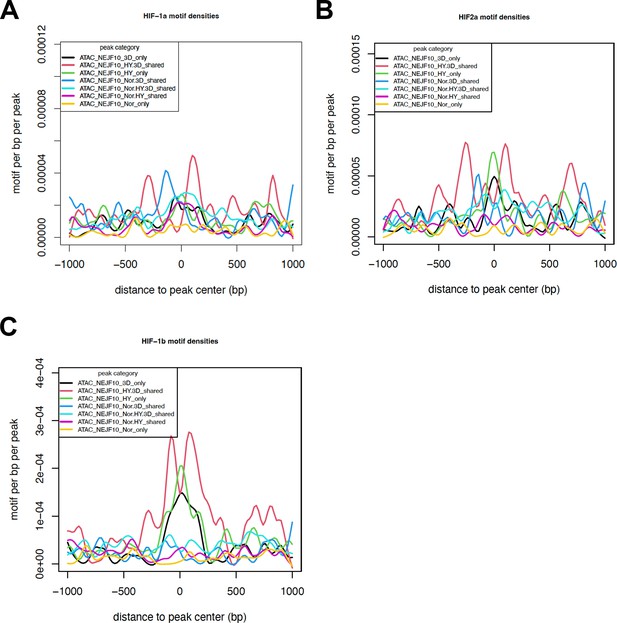
HIF motif densities surrounding the ATAC-seq peaks.
HIF-1a (A), HIF-2a (B), and HIF-1b (C) motif densities around ATAC-seq open chromatin regions (±1000 bp) by categories. Motif density was determined by HOMER (Hypergeometric Optimization of Motif EnRichment) program and normalized to that in a background sequence of equal length. X-axis indicates the distance to peak center (bp).
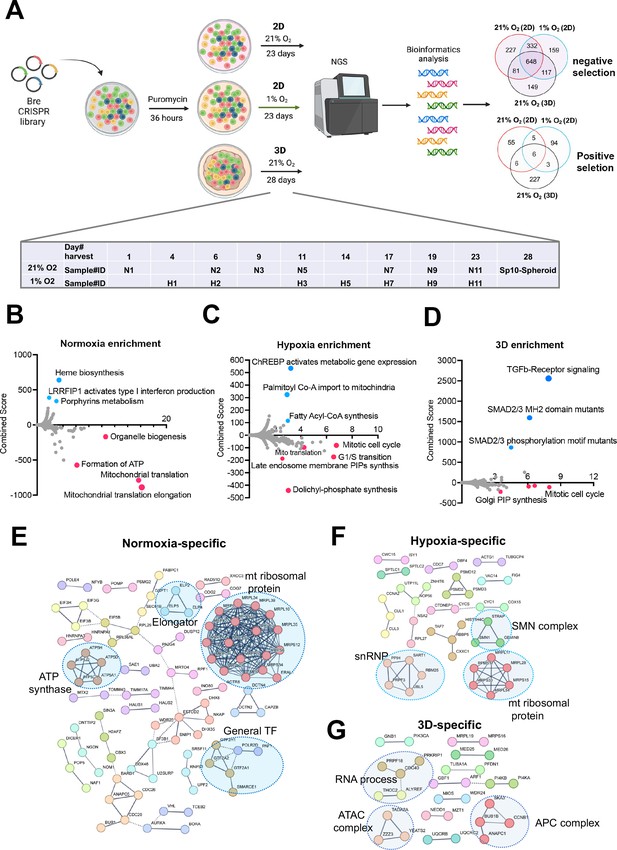
Identification of cell fitness genes in 2D hypoxia, 2D normoxia, and 3D normoxia.
(A) Scheme showing the procedure of genome- wide CRISPR screen of the ABC-Myc NEJF10 cell line treated with different growth environments. Venn analysis of essential genes (negative selection) and anti-proliferative genes (positive selection) reveals gene essentiality for cellular fitness identified in 2D normoxia, 2D hypoxia, and 3D Normoxia. The table is a report of when samples were harvested with N representing 2D normoxia, H representing 2D hypoxia, and Sp10- Spheroid representing 3D normoxia grown cells. Created with BioRender.(B, C, D) Pathway enrichment analysis reported as an enrichment score with negative values indicating negative selection, positive values indicating positive selection, and values of zero indicating no relationship of the respective condition. The colored points highlight significant upregulation (blue) or down regulation (red), whereas grey colored points were not significantly enriched. (E, F, G) Pathways within a protein-protein interaction network of genes essential in cellular fitness are selectively enriched in normoxic, hypoxic, and 3D specific environments. Related genes are clustered by color with some circled to indicate functional complexes.
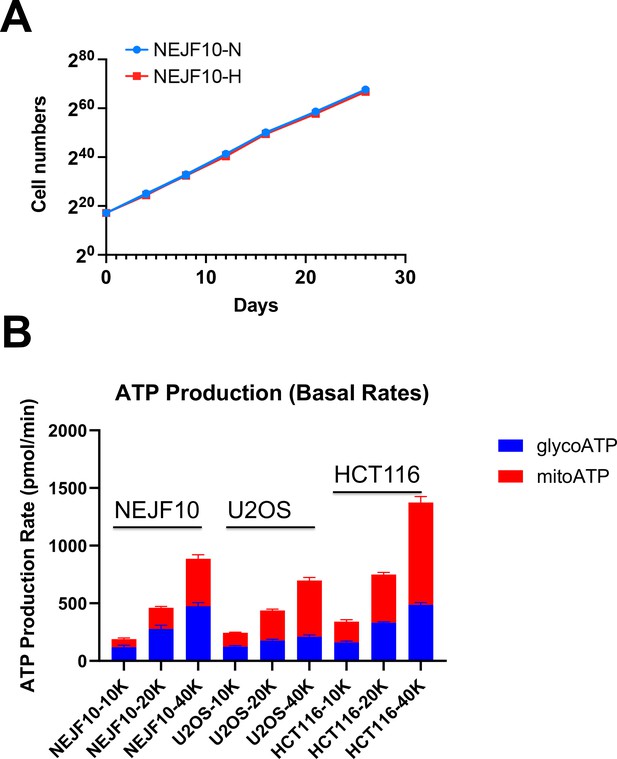
NEJF10 cells tolerate chronic hypoxia and more rely on ATP from glycolysis.
(A) Cell counts over time for NEJF10 cells cultured in 2D under 1% oxygen (hypoxia) and normoxia. (B) Seahorse measurement of ATP sources of NEJ10 cells seeded with different cell numbers (10 k, 20 k, 40 k), U2OS cells and HCT116 cells cultured in 2D normoxia. n=3. Error bars indicate mean ± SEM.
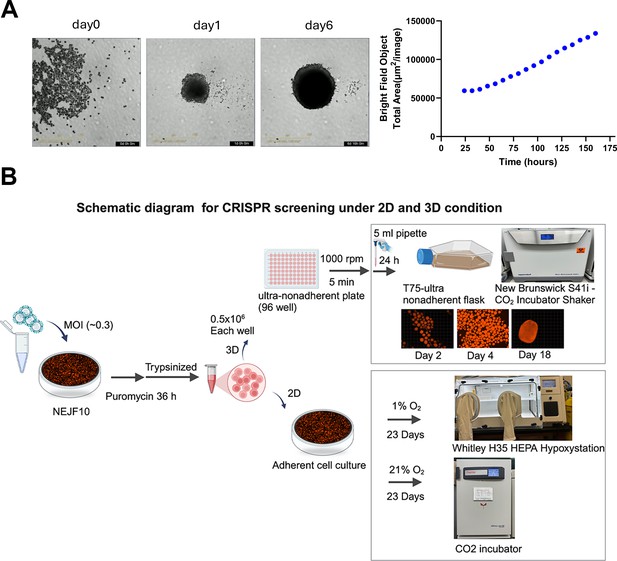
NEJF10 spheroid growth and CRISPR procedure.
(A) Representative images at different time points for 3D growth of NEJF10 seeded with 3000 cells per well in 96 well plate (left). The 3D growth curve monitored by IncuCyte.(right). (B) The schematic showing the CRISPR procedure for NEJF10 cells cultured under different conditions in T75 flask. Created with BioRender.
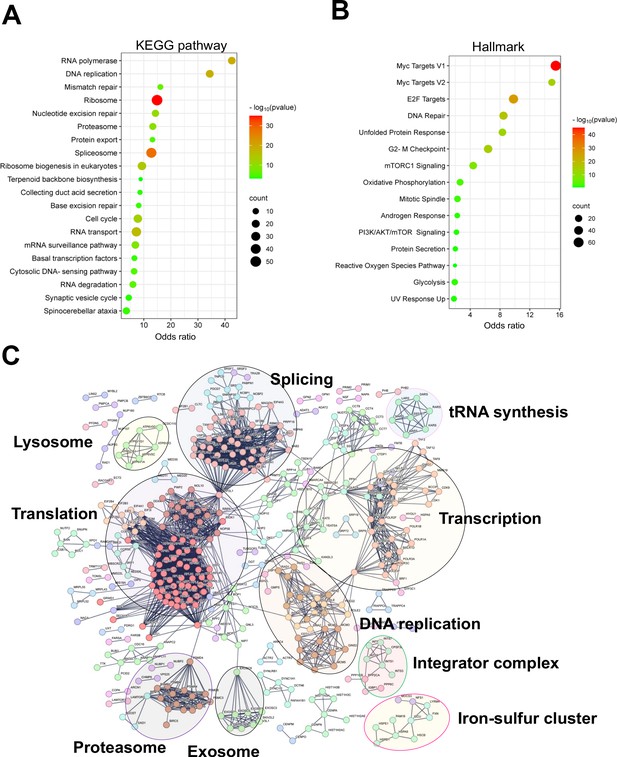
Shared essential genes under normoxia 3D, normoxia 2D, and hypoxia 2D.
(A) Bubble blot showing KEGG pathway enrichment for the shared essential genes under normoxia 3D, normoxia 2D, and hypoxia 2D. (B) Bubble blot showing hallmark pathway enrichment for the shared essential genes under normoxia 3D, normoxia 2D, and hypoxia 2D. (C) STRING interaction network analysis for the shared essential genes under normoxia 3D, normoxia 2D, and hypoxia 2D.
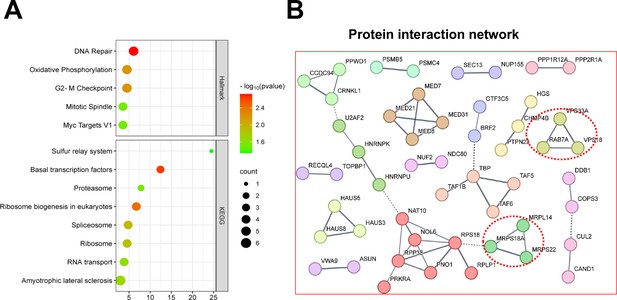
Shared essential genes under normoxia 3D and hypoxia 2D.
(A) Bubble blot showing hallmark (top) KEGG (bottom) pathway enrichment for the shared essential genes under normoxia 3D and hypoxia 2D. (B) STRING interaction network analysis for the shared essential genes under normoxia 3D and hypoxia 2D.
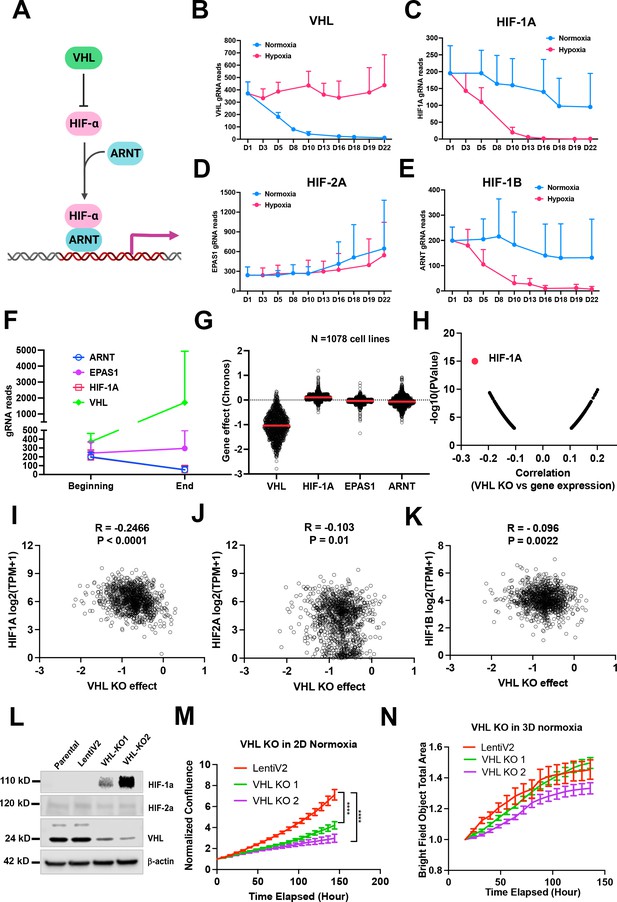
Fitness incompatibility of VHL-HIF1a pathway in normoxia vs hypoxia or 3D.
(A) Scheme representing the VHL-HIF pathway. The HIF-1 and HIF-2a family of transcription factors are degraded by VHL, an E3 ubiquitin ligase, in normoxia. In the presence of hypoxia or loss function of VHL, HIFa is present and dimerizes with nuclear HIF1b or ARNT to drive gene transcription. (B–E) The gRNA reads for Vhl (B), Hif-1a (C), Hif-2a or Epas1 (D), Hif-1b or Arnt (E) at different time points in 2D normoxia and 2D hypoxia. Error bar data = mean +/-SD (n=4 for each time point). (F) The gRNA reads for Vhl, Hif-1a, Hif-2a or Epas1, Hif-1b or Arnt at the beginning and end time points in 3D normoxia. (G) CRISPR KO effect of VHL, HIF-1A, HIF-2A (EPAS1), HIF-1B (ARNT) in 1078 human cell lines. Data were extracted from DepMAP database (http://www.depmap.org/). (H–K) Spearman correlation of VHL CRISPR KO effect vs HIF-1A, HIF2A, HIF1B gene expression in 1078 human cell lines. (L) Western blot analysis of VHL CRISPR knockout in HepG2 cells using two gRNAs with indicated antibodies. (M) Cell growth of wild-type and VHL KO HepG2 over time monitored by Incucyte live cell microscopy in real-time in 2D normoxic condition. ****p<0.0001. p value is calculated by student t test for the last time point data. Error bar data = mean +/-SD. (N) Cell growth of wild-type and VHL KO HepG2 over time monitored by Incucyte in real-time in 3D normoxic condition. Error bar data = mean +/-SD.
-
Figure 3—source data 1
The source data contains original raw data of western blots for Figure 3L, labelled.
- https://cdn.elifesciences.org/articles/101299/elife-101299-fig3-data1-v1.zip
-
Figure 3—source data 2
The source data contains original raw data of western blots for Figure 3L.
- https://cdn.elifesciences.org/articles/101299/elife-101299-fig3-data2-v1.zip
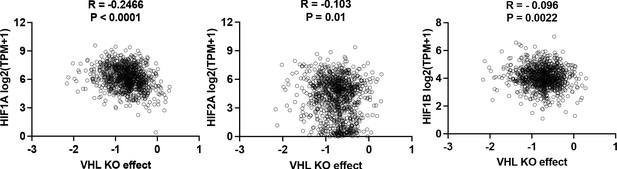
Spearman correlation analysis of VHL knockout effect vs HIF.
Spearman correlation analysis of VHL knockout effect vs expression of HIF-1A, HIF-2A and HIF-1B in 2D normoxia. Data were extracted from DepMap (depmap.org).
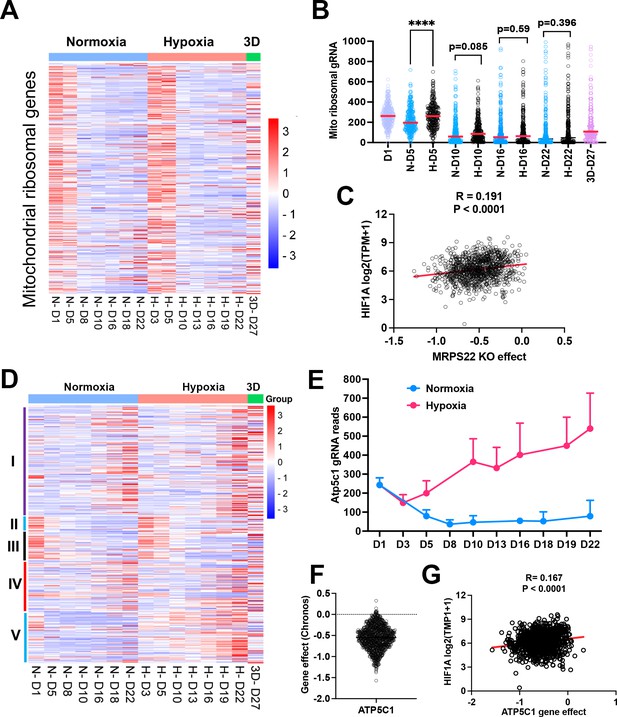
The effect of mitochondrial compartments on cell fitness.
(A) Heatmap showing the gRNA reads for mitochondrial ribosomal genes at different time points in 2D normoxia, 2D hypoxia and 3D normoxia. Scale bar indicates Z score. (B) Comparison of gRNA reads for mitochondrial ribosomal genes at different time points in 2D normoxia, 2D hypoxia and 3D normoxia. ****p<0.0001. p value is calculated by student t test for the last time point data. Data = mean +/-SD. (C) Spearman correlation of MRPS22 CRISPR KO effect vs HIF-1A gene expression in 1078 human cell lines. (D) Heatmap showing the gRNA reads for mitochondrial electron transport genes at different time points in 2D normoxia, 2D hypoxia, and 3D normoxia. Scale bar indicates Z socre. (E) The gRNA reads for Atp5c1 at different time points in 2D normoxia and 2D hypoxia. Error bar data = mean +/-SD. (F) CRIPSR KO effect of ATP5C1 in 1078 human cell lines. Data were extracted from DepMAP database (https://depmap.org/portal/home/). (G) Spearman correlation of ATP5C1 CRISPR KO effect vs HIF-1A gene expression in 1078 human cell lines.
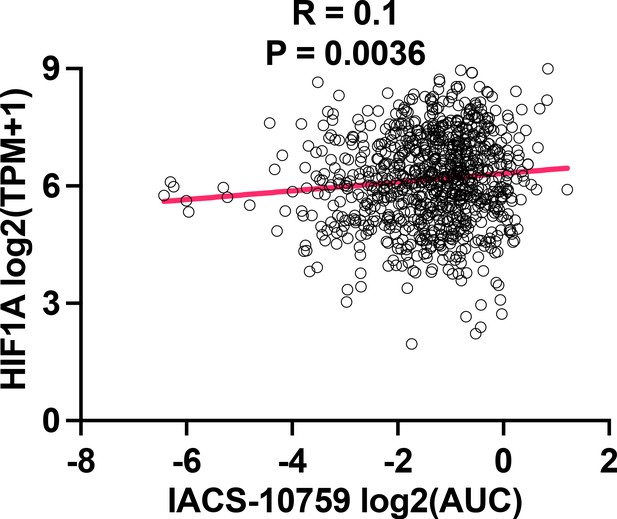
Spearman correlation analysis of IACS-10759 effect vs HIF1A expression.
Spearman correlation analysis of IACS-10759 effect vs expression of HIF-1A in 2D normoxia. Data were extracted from DepMap (depmap.org).
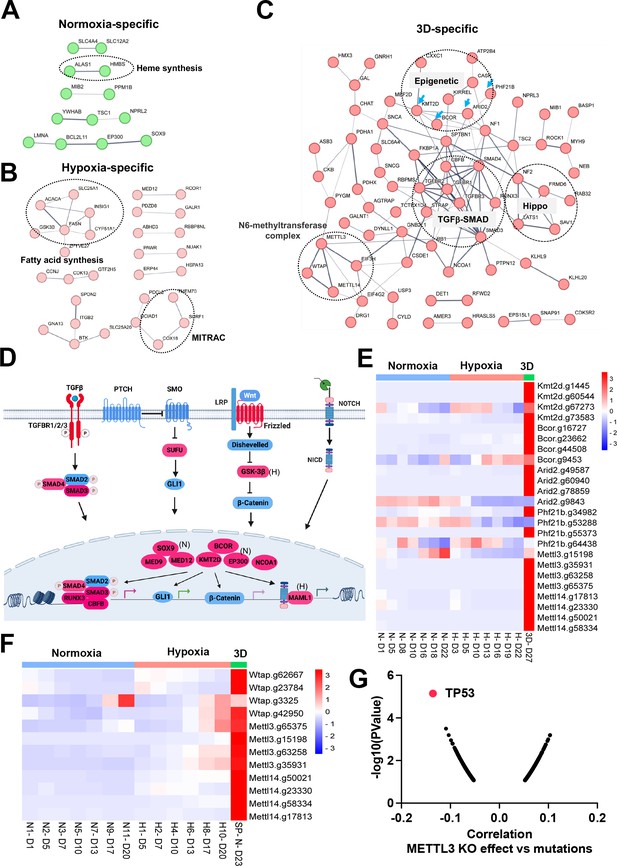
Organogenesis signaling and epigenetic modifiers constrain 3D cell proliferation.
(A–C) Positive selection of pathways within protein-protein interaction networks enriched specifically in 2D normoxia, 2D hypoxia, and 3D normoxia. (D) The graphic illustrates organogenesis signaling pathways positively selected in 3D. Please note that we included hits from normoxia (Sox9, Ep300) and hypoxia (Maml1 and Gsk-3b) in the related pathways. Created with BioRender. (E) The heatmap for the normalized gRNA reads for epigenetic genes in 2D hypoxia and normoxia and 3D normoxia. Red color indicates positive selection. Scale bar indicates Z score. (F) The heatmap for the normalized gRNA reads for WMM complex of NEJF6 cells in 2D hypoxia and normoxia and 3D normoxia. Scale bar indicates Z score. (G) Spearman correlation of METTL3 CRISPR KO effect vs TP53 mutations in human cancer cells lines by analyzing DepMAP data (https://depmap.org/portal/home/).
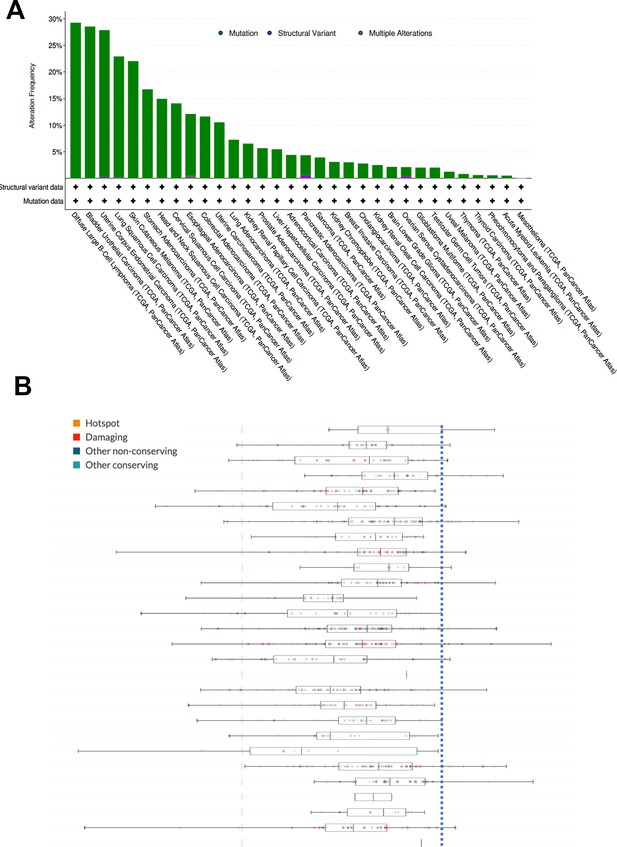
Mutations in KMT2D in human cancers and its knockout effect in human cancer cell lines.
(A) Genetic mutations of KMT2D across different types of human cancer. Data were extracted from cBioportal (https://www.cbioportal.org). (B) Knockout effect of KMT2D across different types of human cancer cell lines in 2D normoxia. Data were extracted from DepMap. Red color indicates mutations. The blue dotted line indicates CRISPR effect as ‘0’.
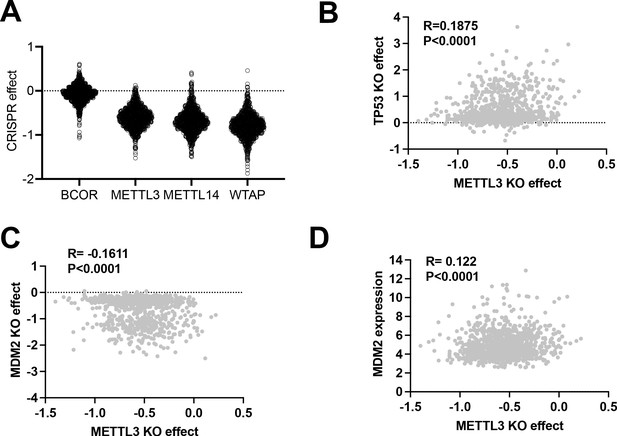
Gene knockout effect in human cancer cell lines under 2D normoxia.
(A) CRISPR knockout effect of BCOR, METTL3, METTL14, and WTAP across different types of human cancer cell lines in 2D normoxia. Data were extracted from DepMap. (B) Spearman correlation of knockout effect for TP53 vs METTL3 across different types of human cancer cell lines in 2D normoxia. Data were extracted from DepMap. (C) Spearman correlation of knockout effect for MDM2 vs METTL3 across different types of human cancer cell lines in 2D normoxia. Data were extracted from DepMap. (D) Spearman correlation of METTL3 knockout effect vs MDM2 expression across different types of human cancer cell lines in 2D normoxia. Data were extracted from DepMap.
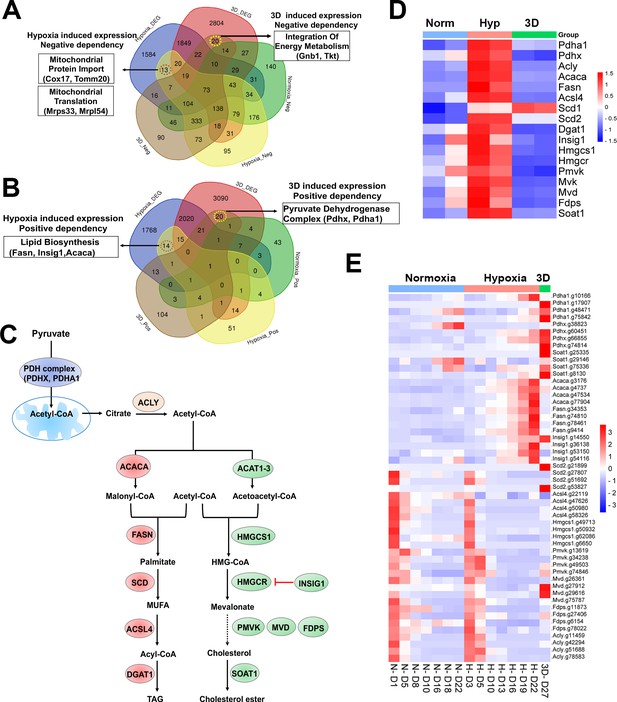
Selective fitness of lipid metabolisms.
(A) Venn diagram showing 13 and 20 genes with negative dependency in specifically hypoxia and 3D induced conditions, respectively, are selectively induced in hypoxia or 3D. (B) Venn diagram showing 14 and 20 genes with positive dependency in specifically hypoxia and 3D induced conditions, respectively, are selectively induced in hypoxia or 3D. (C) A diagram showing lipid biosynthesis pathway of unsaturated fatty acids and cholesterol. (D) The heatmap showing the gene expression involved in lipid biosynthesis. Scale bar indicates Z score. (E) The heatmap showing the normalized gRNA reads for genes involved in lipid biosynthesis in 2D normoxia and hypoxia, 3D normoxia. Scale bar indicates Z score.
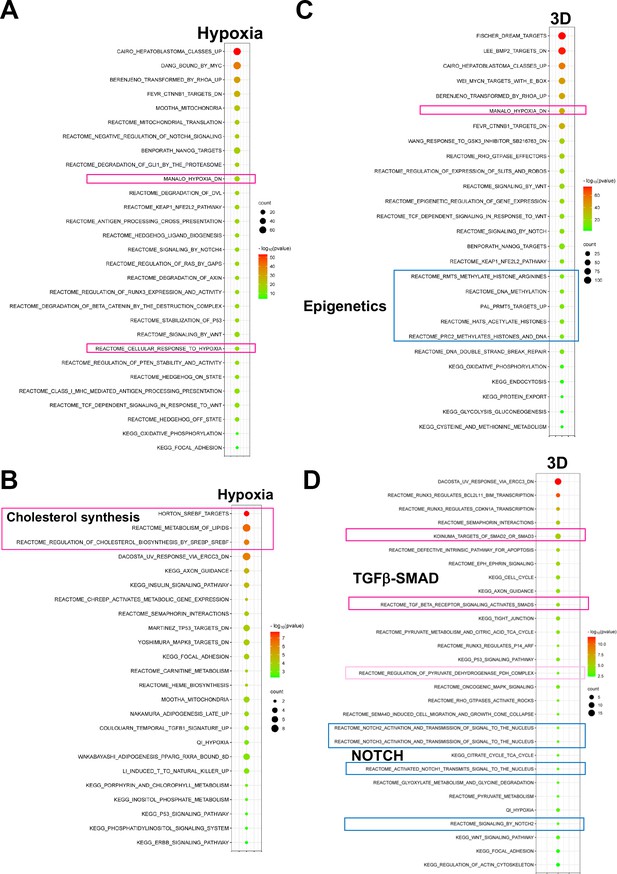
Pathways enrichment for fitness genes whose expression is altered in hypoxia and 3D.
(A) Bubble blot showing pathway enrichment for the essential genes under hypoxia 2D whose expression is also altered in hypoxia 2D. However, this analysis does not exclude the genes that may also be altered under 3D culture. (B) Bubble blot showing pathway enrichment for the positive selection genes under hypoxia 2D whose expression is also altered in hypoxia 2D. However, this analysis does not exclude the genes that may also be altered under 3D culture. (C) Bubble blot showing pathway enrichment for the essential genes under 3D whose expression is also altered in 3D. However, this analysis does not exclude the genes that may also be altered under hypoxia 2D culture. (D) Bubble blot showing pathway enrichment for the positive selection genes under 3D whose expression is also altered in 3D. However, this analysis does not exclude the genes that may also be altered under hypoxia 2D culture.
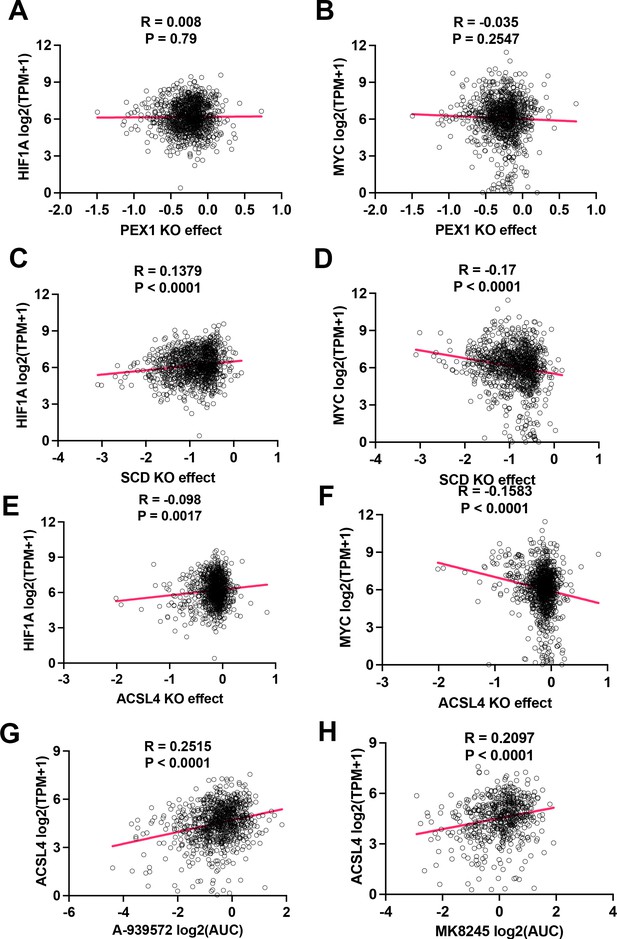
Inhibition effect of lipid metabolic genes of human homologs.
(A) Spearman correlation analysis of PEX1 knockout effect vs expression of HIF-1A in 2D normoxia. Data were extracted from DepMap (depmap.org). (B) Spearman correlation analysis of PEX1 knockout effect vs expression of MYC in 2D normoxia. Data were extracted from DepMap (depmap.org). (C) Spearman correlation analysis of SCD knockout effect vs expression of HIF-1A in 2D normoxia. Data were extracted from DepMap (depmap.org). (D) Spearman correlation analysis of SCD knockout effect vs expression of MYC in 2D normoxia. Data were extracted from DepMap (depmap.org). (E) Spearman correlation analysis of ACSL4 knockout effect vs expression of HIF-1A in 2D normoxia. Data were extracted from DepMap (depmap.org). (F) Spearman correlation analysis of ACSL4 knockout effect vs expression of MYC in 2D normoxia. Data were extracted from DepMap (depmap.org). (G) Spearman correlation analysis of A-939572 effect vs expression of ACSL4 in 2D normoxia. Data were extracted from DepMap (depmap.org). (H) Spearman correlation analysis of MK8245 effect vs expression of ACSL4 in 2D normoxia. Data were extracted from DepMap (depmap.org).
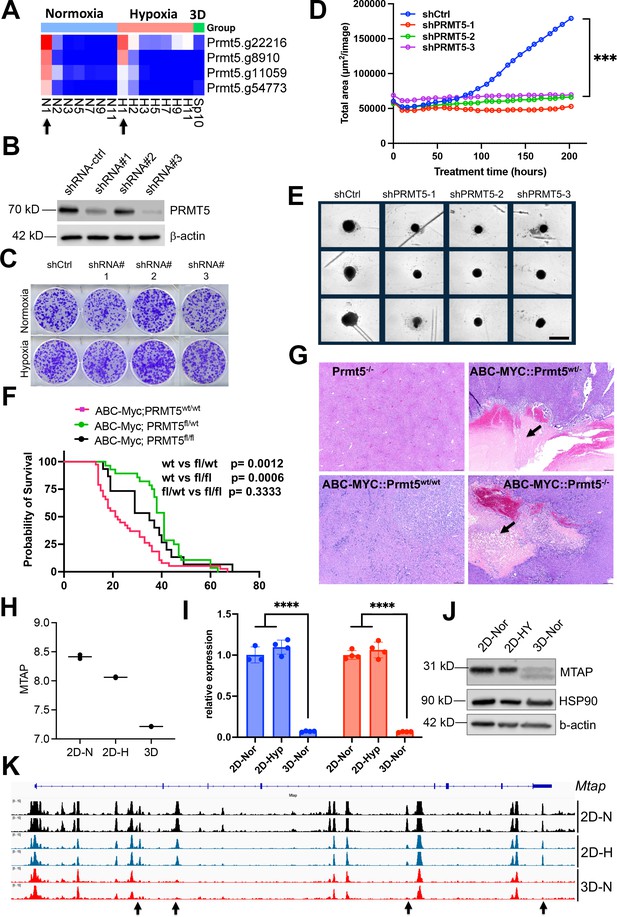
Synthetic lethality of partial Prtm5 loss with 3D.
(A) The heatmap showing the normalized gRNA reads for Prmt5 in 2D normoxia and hypoxia, 3D normoxia. Arrows indicate the first samples harvested for analysis. (B) Western blot analysis with indicated antibodies after Prtm5 knockdown in NEJF10 cells. (C) Colony formation of NEJF10 cells after 5 days of Prmt5 knockdown in 2D normoxia and hypoxia. Cells were stained with crystal violet. (D) Incucyte monitoring of cell proliferation grown in 3D after Prtm5 knockdown in NEJF10 cells. ***p<0.001 for comparison of shCtrl with each of shPrmt5. p value is calculated by student t test by comparing the last reading. N=3 per group. Data = mean +/- SD. (E) Snapshot of NEJF10 cell with or without Prmt5 knockdown in 3D. Scale bar = 400µm. (F) Kaplan-Meier survival for mice of ABC-Myc::Prmt5+/+, ABC-Myc::Prmt5+/-, and ABC-Myc::Prmt5-/-. p values are calculated by log-rank test. (G) Hematoxylin and eosin stain for tumor tissues obtained from mice of Prtm5-/-, ABC-Myc::Prmt5+/+, ABC-Myc::Prmt5+/-, and ABC-Myc::Prmt5-/-. Arrows indicate necrosis in tumor areas. Scale bar = 500 μm. (H) Quantification of normalized Mtap mRNA under 2D normoxia, 2D hypoxia, and 3D normoxia from RNA-seq results. n=2 for each group. (I) Real-time quantitative PCR to determine the expression of Mtap in NEJF10 cells cultured under 3D normoxia, 2D normoxia, and hypoxia for 3 days. Blue and orange indicate results obtained from two different pairs of PCR primers against Mtap. n=3 for each group.****p<0.0001 student t test. (J) Western blot analysis with indicated antibodies of NEJF10 whole cell lysates cultured from 3D normoxia, 2D normoxia, and hypoxia for 3 days. (K) IGV snapshot showing the ATAC-seq result for Mtap gene. The black arrow indicates the enhancers in Mtap gene are selectively lost in 3D.
-
Figure 7—source data 1
The source data contains original raw data of western blots for Figure 7B, labelled.
- https://cdn.elifesciences.org/articles/101299/elife-101299-fig7-data1-v1.zip
-
Figure 7—source data 2
The source data contains original raw data of western blots for Figure 7B.
- https://cdn.elifesciences.org/articles/101299/elife-101299-fig7-data2-v1.zip
-
Figure 7—source data 3
The source data contains original raw data of western blots for Figure 7J, labelled.
- https://cdn.elifesciences.org/articles/101299/elife-101299-fig7-data3-v1.zip
-
Figure 7—source data 4
The source data contains original raw data of western blots for Figure 7J.
- https://cdn.elifesciences.org/articles/101299/elife-101299-fig7-data4-v1.zip
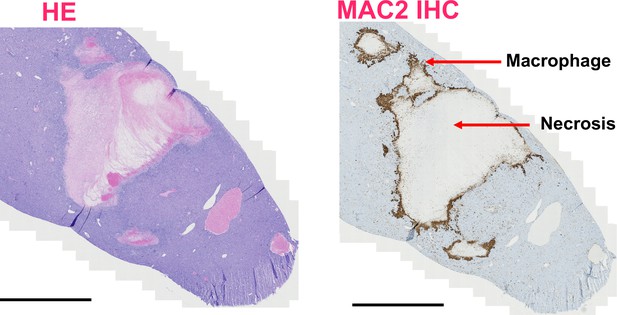
Necrosis and inflammation of liver tumor with Prmt5 knockout.
(A) H&E staining of ABC-MYC liver tumors with Prmt5 knockout. (B) MAC2 immunohistochemistry staining of ABC-MYC liver tumors with Prmt5 knockout. Scale bar = 2000µm.
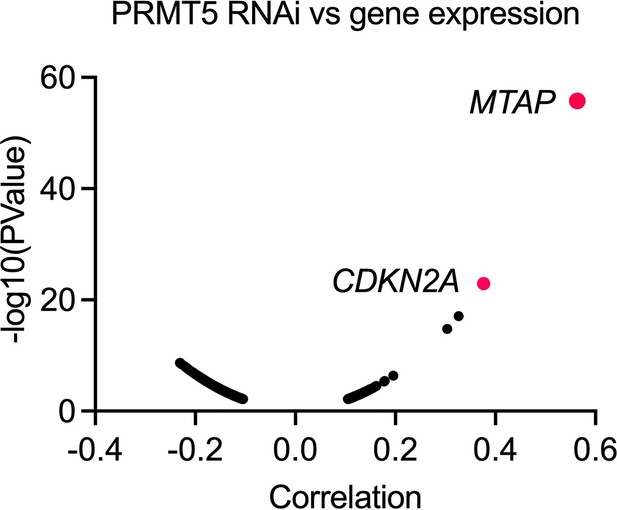
PRMT5 knockdown effect vs MTAP expression.
Spearman correlation of knockdown effect for PRMT5 vs MTAP expression across different types of human cancer cell lines in 2D normoxia. Data were extracted from DepMap.
Tables
Reagent type (species) or resource | Designation | Source or reference | Identifiers | Additional information |
---|---|---|---|---|
Strain, strain background (Mouse, B6, both male and female) | Albumin-Cre | THE JACKSON LABORATORY | RRID:IMSR_JAX:003574 | |
Strain, strain background (Mouse, B6, both male and female) | Rosa26StopFL-MYC | THE JACKSON LABORATORY | RRID:IMSR_JAX:020458 | |
Strain, strain background (Mouse, B6/129 mix both male and female) | Prmt5 flox | THE JACKSON LABORATORY | RRID:IMSR_JAX:034414 | |
Cell line (Murine) | NEJF10 | This paper | N/A | Cell line maintained in Jun Yang lab |
Cell line (Murine) | NEJF6 | This paper | N/A | Cell line maintained in Jun Yang lab |
Cell line (Murine) | NEJF10-shCtrl | This paper | N/A | Cell line maintained in Jun Yang lab |
Cell line (Murine) | NEJF10-shPRMT5-1 | This paper | N/A | Cell line maintained in Jun Yang lab |
Cell line (Murine) | NEJF10-shPRMT5-2 | This paper | N/A | Cell line maintained in Jun Yang lab |
Cell line (Murine) | NEJF10-shPRMT5-3 | This paper | N/A | Cell line maintained in Jun Yang lab |
Cell line (Homo sapiens) | U2OS | ATCC | HTB96 | |
Cell line (Homo sapiens) | HCT116 | ATCC | CCL-247 | |
Cell line (Homo sapiens) | HepG2 | ATCC | HB-8065 | |
Cell line (Homo sapiens) | HepG2-LeveV2 | This paper | N/A | Cell line maintained in Jun Yang lab |
Cell line (Homo sapiens) | HepG2-VHL-KO-1 | This paper | N/A | Cell line maintained in Jun Yang lab |
Cell line (Homo sapiens) | HepG2-VHL-KO-2 | This paper | N/A | Cell line maintained in Jun Yang lab |
Antibody | Anti-HIF1α (Rabbit, polyclonal) | Cayman | Cat# 10006421, RRID:AB_409037 | WB (1:200) |
Antibody | Anti-HIF2α (Rabbit, polyclonal) | Novus | Cat# NB100-122, RRID:AB_10002593 | WB (1:500) |
Antibody | Anti-VHL (Rabbit, polyclonal) | Cell Signaling Technology | Cat# 68547 S, RRID:AB_2716279 | WB (1:1000) |
Antibody | Anti-PRMT5 (Rabbit, polyclonal) | ABclonal | Cat# A1520, RRID:AB_2762092 | WB (1:1000) |
Antibody | Anti-MTAP (Rabbit, polyclonal) | Cell Signaling Technology | Cat# 4158 S, RRID:AB_1904054 | WB (1:1000) |
Antibody | Anti-HSP90 (Mouse, monoclonal) | Santa Cruz | Cat# sc13119, RRID:AB_675659 | WB (1:1000) |
Antibody | Anti-ACTIN (Mouse, monoclonal) | Sigma | Cat# A1978, RRID:AB_4766 | WB (1:5000) |
Antibody | Anti-MAC2 (Rat, monoclonal) | Accurate/CEDARLANE | Cat# CL-8942AP, RRID:AB_10060357 | IHC (1:1000) |
Recombinant DNA reagent | LeveV2-vector | Addgene | 52961 | |
Recombinant DNA reagent | VHL-gRNA-1 | GenScript | SC1678 | |
Recombinant DNA reagent | VHL-gRNA-2 | GenScript | SC1678 | |
Recombinant DNA reagent | shCtrl | VectorBuilder | VB010000-0009mxc | |
Recombinant DNA reagent | shPRMT5-1 | VectorBuilder | VB900070-3948cva | |
Recombinant DNA reagent | shPRMT5-2 | VectorBuilder | VB900070-3942srh | |
Recombinant DNA reagent | shPRMT5-3 | VectorBuilder | VB900080-7913zan | |
Sequence-based reagent | AlbCre genotyping primer1 | Integrated DNA Technologies | 5’-TGCAAACATCACATGCACAC-3’ | |
Sequence-based reagent | AlbCre genotyping primer2 | Integrated DNA Technologies | 5’-GAAGCAGAAGCTTAGGAAGAT GG –3’ | |
Sequence-based reagent | AlbCre genotyping primer3 | Integrated DNA Technologies | 5’-TTGGCCCCTTACCATAACTG –3’ | |
Sequence-based reagent | Rosa26StopFLMYC WT genotyping primer1 | Integrated DNA Technologies | 5’-CCAAAGTCGCTCTGAGTTGTTATC-3’ | |
Sequence-based reagent | Rosa26StopFLMYC genotyping primer2 WT | Integrated DNA Technologies | 5’- GAGCGGGAGAAATGGATATG –3’ | |
Sequence-based reagent | Rosa26StopFLMYC MYC genotyping primer1 | Integrated DNA Technologies | 5’- CCAAGAGGGTCAAGTTGGA –3’ | |
Sequence-based reagent | Rosa26StopFLMYC MYC genotyping primer2 | Integrated DNA Technologies | 5’-GCAATATGGTGGAAAATAAC-3’ | |
Sequence-based reagent | PRMT5 flox genotyping primer1 | Integrated DNA Technologies | 5’- GATACCAGGACCCACACTGG-3’ | |
Sequence-based reagent | PRMT5 flox genotyping primer2 | Integrated DNA Technologies | 5’-CTTAGGGGCTTCATGCATCT-3’ | |
Sequence-based reagent | PR-PCR 18 s Forward | Integrated DNA Technologies | 5’-GCTTAATTTGACTCAACACGGGA-3’ | |
Sequence-based reagent | PR-PCR 18 s Reverse | Integrated DNA Technologies | 5’-AGCTATCAATCTGTCAATCCTGTC-3’ | |
Sequence-based reagent | PR-PCR MTAP Forward-1 | Integrated DNA Technologies | 5’- ACGGCGGTGAAGATTGGAATA-3’ | |
Sequence-based reagent | PR-PCR MTAP Reverse-1 | Integrated DNA Technologies | 5’- ATGGCTTGCCGAATGGAGTAT –3’ | |
Sequence-based reagent | PR-PCR MTAP Forward-2 | Integrated DNA Technologies | 5’- AAGCCATCCGATGCCTTAATTT-3’ | |
Sequence-based reagent | PR-PCR MTAP Reverse-2 | Integrated DNA Technologies | 5’- TTGCCTGGTAGTTGACTTTTGAA –3’ | |
Commercial assay or kit | short tandem repeat (STR) profiling using PowerPlex 16 HS System | Promega | DC2320 | |
Commercial assay or kit | Mycoplasma PCR Detection Kit | Sigma-Aldrich | MP0035 | |
Commercial assay or kit | SuperSignal West Pico PLUS Chemiluminescent Substrate | Thermo Fisher Scientific | 34580 | |
Commercial assay or kit | NEBNext HiFi 2 x PCR Master Mix | NEB | M0541L | |
Commercial assay or kit | Mini-Elute PCR Purification Kit | QIAGEN | 28004 | |
Commercial assay or kit | PowerUp SYBR Green master mix | Applied Biosystems | 4367659 | |
Commercial assay or kit | SuperScript IV Reverse Transcriptase | Thermo Fisher Scientific | 18091050 | |
Commercial assay or kit | Kapa biosystems mouse genotyping extraction kit | Kapa biosystems | KK7352 | |
Commercial assay or kit | TDE1,TAGMENT DNA ENZYME,24 RXN | Illumina | 15027865 | |
Commercial assay or kit | RNeasy Plus Mini Kit (250) | QIAGEN | 74136 | |
Commercial assay or kit | Seahorse XF Real-Time ATP Rate Assay Kit | Agilent | 103592–100 | |
Commercial assay or kit | Seahorse XF Cell Mito Stress Test Kit | Agilent | 103015–100 | |
Commercial assay or kit | NucleoBond Xtra EF kits | Takara Bio USA | 740424–50 | |
Chemical compound, drug | Dulbecco’s Modified Eagle Medium | Corning | 15–013-CV | |
Chemical compound, drug | McCoy’s 5 A Medium | Thermo Fischer Scientific | 16600082 | |
Chemical compound, drug | L-Glutamine | Corning | A2916801 | |
Chemical compound, drug | Fetal bovine serum | Sigma-Aldrich | A5256701 | |
Chemical compound, drug | Penicillin/Streptomycin | Thermo Fischer Scientific | 15140122 | |
Chemical compound, drug | XF calibrant | Agilent | 100840–000 | |
Chemical compound, drug | XF DMEM medium | Agilent | 103575–100 | |
Chemical compound, drug | Glucose | Agilent | 103577–100 | |
Chemical compound, drug | pyruvate | Agilent | 103578–100 | |
Chemical compound, drug | L-glutamine | Agilent | 103579–100 | |
Chemical compound, drug | Tris HCl | BioWorld | 40120297–1 | |
Chemical compound, drug | Dithiothreitol | Thermo Fischer Scientific | A39255 | |
Chemical compound, drug | Bromophenol blue | Thermo Fischer Scientific | A18469.18 | |
Chemical compound, drug | Sodium dodecyl sulfate | Thermo Fischer Scientific | BP166-500 | |
Chemical compound, drug | Glycerol | Sigma | G5516-500ml | |
Chemical compound, drug | TWEEN 20 | Bio-Rad | 1706531 | |
Chemical compound, drug | Gibco PBS, pH 7.4 | Thermo Fischer Scientific | 10-010-072 | |
Chemical compound, drug | Formaldehyde | Thermo Fischer Scientific | F79-1 | |
Chemical compound, drug | Concanavalin A-coated beads | Bangs laboratories | BP531 | |
Chemical compound, drug | KCl | Sigma-Aldrich | 58221–500 ml | |
Chemical compound, drug | NP-40 | Thermo Fisher Scientific | 28324 | |
Chemical compound, drug | Bovine Serum Albumin | Thermo Fisher Scientific | BP1605-100 | |
Chemical compound, drug | Opti-MEM | ThermoFisher Scientific | 31985062 | |
Chemical compound, drug | PEIpro transfection reagent | Polyplus | 101000033 | |
Chemical compound, drug | HEPES | GIBCO | 15630–080 | |
Chemical compound, drug | Proteinase K | Roche | 3115879001 | |
Software, algorithm | Prism 9.0 | Prism 9.0 | Prism 9.0 | |
Software, algorithm | Image J | Image J | https://imagej.nih.gov/ij/ | |
Software, algorithm | Biorender | Biorender.com | https://www.biorender.com/ | |
Software, algorithm | Adobe Illustrator 2024 | Adobe | https://www.adobe.com/it/products/illustrator.html | |
Software, algorithm | SJCRH’s Strongarm pipeline | Center for Applied Bioinformatics, St Jude Children’s Research Hospital | N/A | |
Software, algorithm | HOMER suite v4.8.3 | http://homer.ucsd.edu/homer/ | http://homer.ucsd.edu/homer/ | |
Software, algorithm | Picard (version 1.65) | https://broadinstitute.github.io/picard/ | https://broadinstitute.github.io/picard/ | |
Software, algorithm | MACS2 (version 2.2.7.1) | https://pypi.org/project/MACS2/ | https://pypi.org/project/MACS2/ | |
Software, algorithm | SICER (version 1.1) | https://github.com/dariober/SICERpy (Beraldi, 2017) | https://github.com/dariober/SICERpy | |
Software, algorithm | BEDtools version 2.25.0 | https://github.com/arq5x/bedtools2 (Quinlan, 2025) | https://github.com/arq5x/bedtools2 | |
Software, algorithm | bedGraphToBigWig | https://hgdownload.soe.ucsc.edu/admin/exe/linux.x86_64.v369/ | https://hgdownload.soe.ucsc.edu/admin/exe/linux.x86_64.v369/ | |
Software, algorithm | Integrated Genomics Viewer (IGV 2.3.82) | https://igv.org/doc/desktop/ | https://igv.org/doc/desktop/ | |
Software, algorithm | EdgeR (version 3.16.5) | https://bioconductor.org/packages/edgeR | https://bioconductor.org/packages/edgeR | |
Software, algorithm | GSEA | https://www.gsea-msigdb.org/gsea/index.jsp | https://www.gsea-msigdb.org/gsea/index.jsp | |
Software, algorithm | Draw Venn Diagram | https://bioinformatics.psb.ugent.be/webtools/Venn/ | https://bioinformatics.psb.ugent.be/webtools/Venn/ | |
Software, algorithm | Heatmap | https://www.bioinformatics.com.cn/en?keywords=heatmap | https://www.bioinformatics.com.cn/en?keywords=heatmap | |
Software, algorithm | STRING | https://string-db.org/ | https://string-db.org/ | |
Software, algorithm | Enrichr | https://maayanlab.cloud/Enrichr/ | https://maayanlab.cloud/Enrichr/ | |
Software, algorithm | DepMAP | https://depmap.org/portal/ | https://depmap.org/portal/ | |
Software, algorithm | cBioportal | https://www.cbioportal.org/ | https://www.cbioportal.org/ | |
Software, algorithm | Seahorse software | https://www.agilent.com/en/product/cell-analysis/real-time-cell-metabolic-analysis/xf-software/agilent-seahorse-analytics-787485 | https://www.agilent.com/en/product/cell-analysis/real-time-cell-metabolic-analysis/xf-software/agilent-seahorse-analytics-787485 | |
Software, algorithm | CRISPR algorithm | https://bitbucket.org/liulab/mageck-vispr/src/master/ | https://bitbucket.org/liulab/mageck-vispr/src/master/ | |
Software, algorithm | Incucyte software | https://shop.sartorius.com/us/p/incucyte-spheroid-analysis-software-module/9600-0019 | https://shop.sartorius.com/us/p/incucyte-spheroid-analysis-software-module/9600-0019 | |
Other (Deposited data) | RNA-seq | This paper | GSE240980 | |
Other (Deposited data) | ATAC-seq | This paper | GSE262074 |
Additional files
-
Supplementary file 1
Differentially expressed genes for NEJF10 in hypoxia vs normoxia 2D culture.
- https://cdn.elifesciences.org/articles/101299/elife-101299-supp1-v1.xlsx
-
Supplementary file 2
Differentially expressed genes for NEJF10 in 3D vs 2D culture in normoxia.
- https://cdn.elifesciences.org/articles/101299/elife-101299-supp2-v1.xlsx
-
Supplementary file 3
Analysis of five major splicing events (2D Normoxia vs 2D Hypoxia).
- https://cdn.elifesciences.org/articles/101299/elife-101299-supp3-v1.xlsx
-
Supplementary file 4
Analysis of five major splicing events (2D Normoxia vs 3D Normoxia).
- https://cdn.elifesciences.org/articles/101299/elife-101299-supp4-v1.xlsx
-
Supplementary file 5
Analysis of five major splicing events (3D Normoxia vs 2D Hypoxia).
- https://cdn.elifesciences.org/articles/101299/elife-101299-supp5-v1.xlsx
-
Supplementary file 6
KEGG pathway analysis for the spliced genes (2D Hypoxia vs 2D Normoxia).
- https://cdn.elifesciences.org/articles/101299/elife-101299-supp6-v1.xlsx
-
Supplementary file 7
KEGG pathway analysis for the spliced genes (3D Normoxia vs 2D Normoxia).
- https://cdn.elifesciences.org/articles/101299/elife-101299-supp7-v1.xlsx
-
Supplementary file 8
KEGG pathway analysis for the spliced genes (3D Normoxia vs 2D Hypoxia).
- https://cdn.elifesciences.org/articles/101299/elife-101299-supp8-v1.xlsx
-
Supplementary file 9
NEJF10 CRISPR results with Venn analysis.
- https://cdn.elifesciences.org/articles/101299/elife-101299-supp9-v1.xlsx
-
Supplementary file 10
NEJF6 CRISPR results.
- https://cdn.elifesciences.org/articles/101299/elife-101299-supp10-v1.xlsx
-
Supplementary file 11
Venn analysis for the differentially expressed genes and fitness genes cultured under different conditions.
- https://cdn.elifesciences.org/articles/101299/elife-101299-supp11-v1.xlsx
-
MDAR checklist
- https://cdn.elifesciences.org/articles/101299/elife-101299-mdarchecklist1-v1.pdf