The known unknowns of the Hsp90 chaperone
Figures
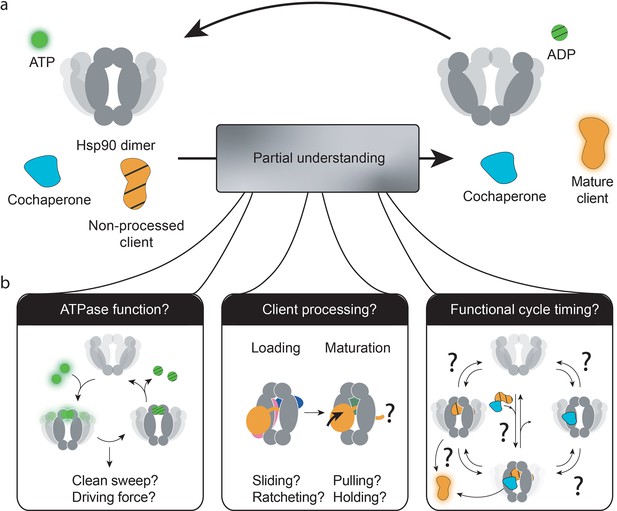
Three central questions about heat-shock protein 90’s (Hsp90’s) functional mechanism.
(a) The current combined knowledge describes a general functional cycle of eukaryotic Hsp90, where Hsp90 hydrolyses ATP at a slow rate, interacts with cochaperones and clients, and (by only partially understood mechanisms) matures clients to their functional form. (b) Several open questions remain, as illustrated: What is the role of Hsp90’s ATPase activity? How does Hsp90, alone or in cooperation with cochaperones, process its vast client base? Which is the sequence of interactions by which the Hsp90 system operates to produce functional clients? And which are reversible processes as compared to unidirectionally driven ones? Which are fast or rate-limiting steps?.
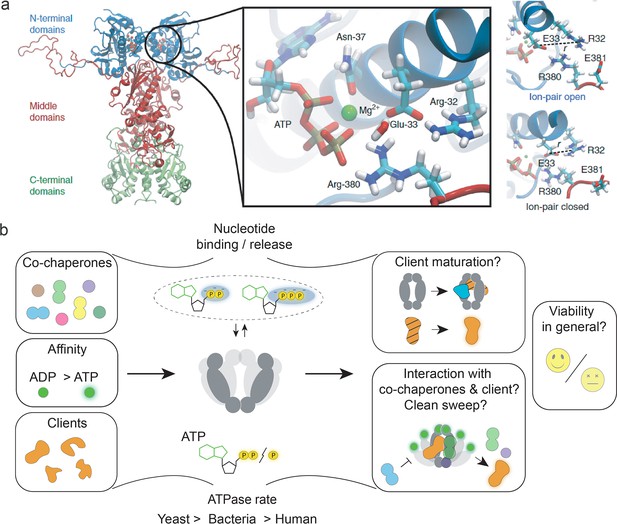
The role of heat-shock protein 90’s (Hsp90’s) ATPase function.
(a) Structure of the yeast Hsp90 dimer (PDB ID: 2CG9, Ali et al., 2006). The insert obtained from an molecular dynamics (MD) simulation shows the active site with bound ATP. MD simulations indicate a role of ion pair opening in the ATP hydrolysis reaction. Panel A is reproduced from Figures 1 and 3 from Mader et al., 2020. (b) Visual summary of Hsp90’s ATPase rate modulation by interactors, ATPase rate differences among different species, and partially understood aspects of Hsp90’s ATPase function, such as interactor dissociation. The notion that ATP hydrolysis is essential for yeast cell viability was challenged by recent evidence. Nucleotide binding and release may be sufficient to support yeast cell viability.
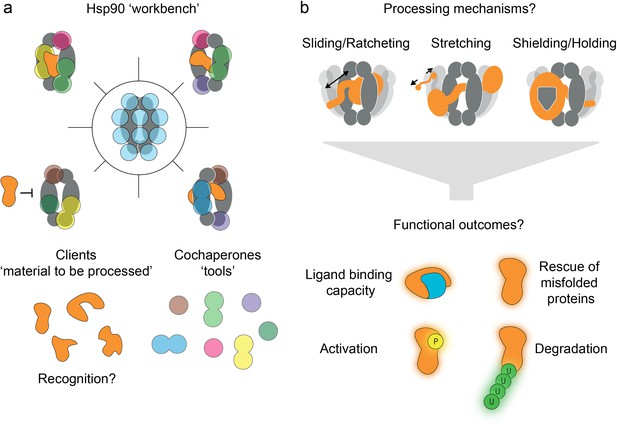
Convergent model of heat-shock protein 90’s (Hsp90’s) functional mechanism.
(a) Hsp90 can be regarded as a workbench, bringing clients and cochaperones (coloured tools) into close proximity via interaction sites spread over the entire protein (blue spots). However, many details of recognition of the numerous clients by Hsp90 and its cochaperones remain elusive. (b) Various client processing modes are being discussed based on growing experimental evidence, such as sliding, ratcheting, stretching, shielding, and holding. These processing modes seem to be client-specific, and they may offer distinct functional outcomes (e.g. misfolding rescue, or targeting for or protection from degradation).
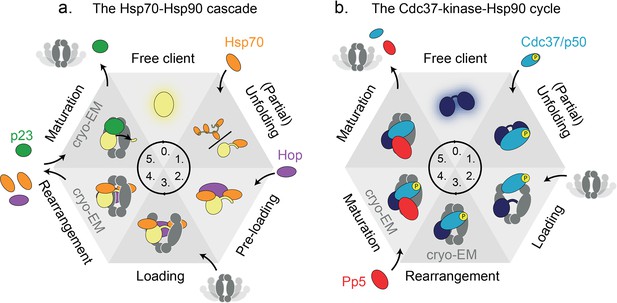
Heat-shock protein 90 (Hsp90’s) functional cycle timing illustrated by two literature examples.
Schematic representation of the two discussed functional cycles of eukaryotic Hsp90, illustrated using six states each. Cryo-EM structures of two of the states exist, as indicated. Many transient interactions and intermediate complexes limit the current understanding of the order and timing of events, as well as their directionality or reversibility. (a) The Hsp70-Hsp90 cascade and (b) the Cdc37-kinase-Hsp90 cycle. Both cycles have in common that the client is (partially) unfolded prior to recruitment to Hsp90 in an intermediate loading complex. Next, by unknown mechanisms, clients are processed by Hsp90 through at least one intermediate maturation state. It is not completely understood, among other details, when ATP binding or hydrolysis by Hsp90 occurs, and how clients are released from these complexes.
Tables
Special features of heat-shock protein 90 (Hsp90) compared to other well-known ATPase proteins (model ATPases): (i) the motor protein kinesin, (ii) the helicase DnaB, (iii) the protease ClpXP.
-
*
ATPase in the presence of substrate.