MagIC-Cryo-EM, structural determination on magnetic beads for scarce macromolecules in heterogeneous samples
Figures
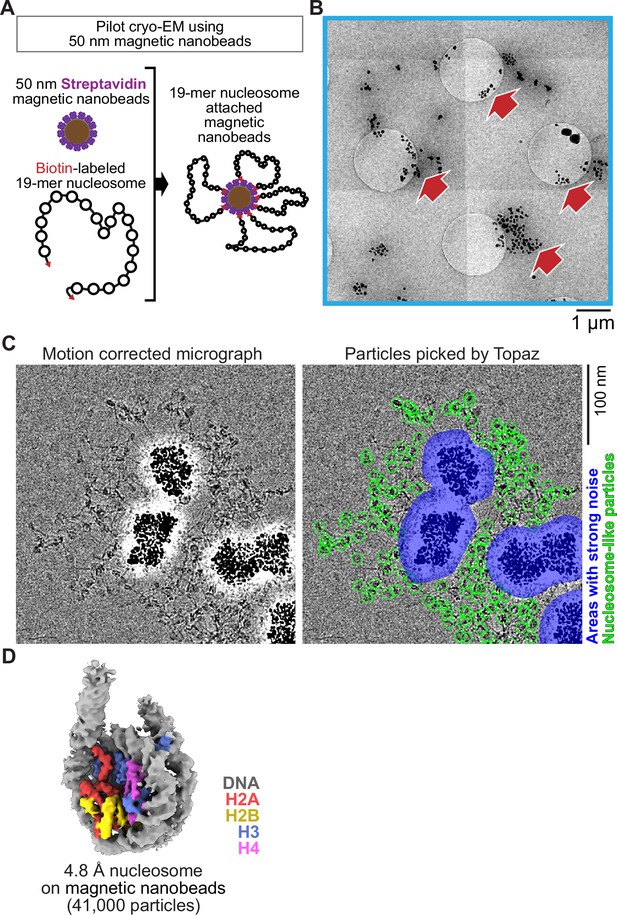
Single particle cryo-EM analysis of poly-nucleosomes attached to magnetic beads.
(A) Schematic of a pilot cryo-EM experiment on magnetic beads. Biotin-labeled 19-mer nucleosome arrays attached to 50 nm streptavidin-coated magnetic nanobeads were loaded onto the cryo-EM grid. (B) Representative medium magnification micrographs. The magnetic beads are seen as black dots (red arrows). (C) Left; a representative highmagnification micrograph. The micrograph was motion-corrected and low-pass filtered to 5 Å resolution. Right; green circles indicate the nucleosome-like particles selected by Topaz, and the blue areas indicate the halo-like scattering. (D) The 3D structure of the nucleosome bound on magnetic beads.
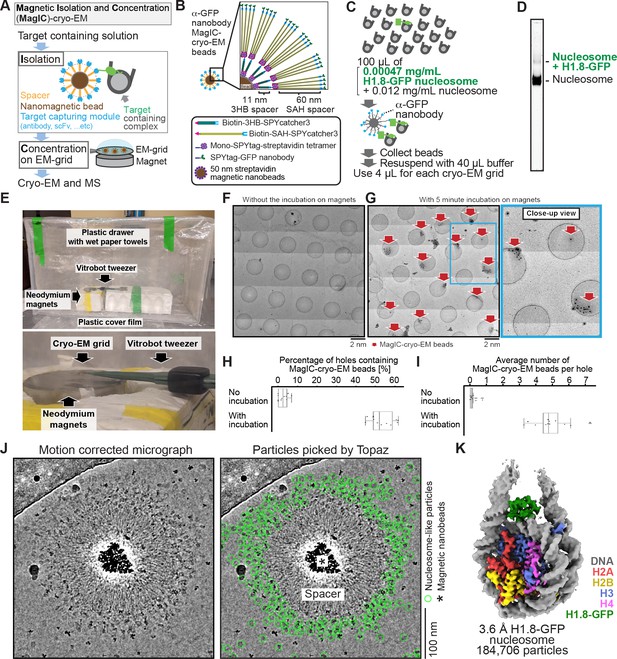
MagIC-Cryo-EM structural determination of low-quantity and low-purity targets.
(A) Schematic depicting the principle steps of MagIC-cryo-EM. (B) Graphical representation of the MagIC-cryo-EM beads with 3HB and SAH spacers and GFP nanobody target capture module. (C) Schematic of MagIC-cryo-EM for in vitro reconstituted H1.8-GFP-bound nucleosomes isolated from an excess of H1.8-free nucleosomes. (D) Native PAGE analysis of H1.8-GFP-bound nucleosomes and unbound nucleosomes in the input. DNA staining by SYTO-60 is shown. (E) A handmade humidity chamber used for the 5 min incubation of the cryo-EM grids on the magnet. The humidity chamber was assembled using a plastic drawer. Wet tissues are attached to the side walls of the chamber, which is sealed with a plastic cover to maintain high humidity. Two pieces of neodymium magnets are stacked. A graphene grid is held by a non-magnetic vitrobot tweezer and placed on the magnets. 4 µL of sample is applied on the grid and incubated for 5 min. (F) Micrograph montage of the grids without using magnetic concentration. The GFP-nanobody-MagIC-cryo-EM beads (4 µL of 12.5 pM beads) were applied on the graphene-coated Quantifoil R 1.2/1.3 grid and vitrified without incubation on a magnet. (G) Micrograph montage of the grids without using magnetic concentration. The GFP-nanobody-MagIC-cryo-EM beads (4 µL of 12.5 pM beads) were applied on the graphene-coated Quantifoil R 1.2/1.3 grid and vitrified with 5 min incubation on two pieces of 40x20 mm N52 neodymium disc magnets. (H) Quantitative analysis of the percentage of holes containing MagIC-cryo-EM beads. Each data point represents the percentage of holes containing MagIC-cryo-EM beads on each square mesh. (I) Quantitative analysis of the average number of MagIC-cryo-EM beads per hole. Each data point represents the average number of MagIC-cryo-EM beads per hole on each square mesh. The edges of the boxes and the midline indicates the 25th, 50th, and 75th percentiles. Whiskers indicate the maximum and lowest values in the dataset, excluding outliers. For the quantification, 11 square meshes with 470 holes without magnetic concentration and 11 square meshes with 508 holes with 5 min incubation on magnets were used. (J) Representative motion corrected micrographs of in vitro reconstituted H1.8-GFP nucleosomes captured by MagIC-cryo-EM beads. The micrographs were low-pass filtered to 10 Å resolution. Green circles indicate the nucleosome-like particles picked by Topaz. (K) 3D structure of the in vitro reconstituted H1.8-GFP-bound nucleosome determined through MagIC-cryo-EM. The pipeline for structural analysis is shown in Figure 2—figure supplement 2.
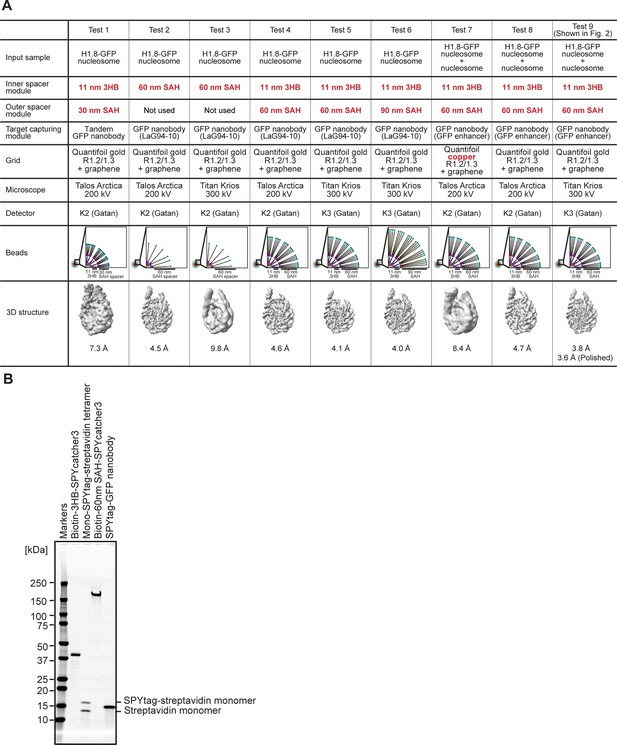
Optimization of the MagIC-cryo-EM beads.
(A) Spacer modules attached to the 50 nm magnetic beads were optimized to cover intense halo-like noises formed by the beads. The in vitro reconstituted H1.8-GFP-bound nucleosomes were used for MagIC-cryo-EM optimization with various versions of the spacer modules. The cartoons depict the beads and spacer length of each experiment. The critical parameters are colored with red. The bottom 3D maps are the cryo-EM structures determined in each experiment. For the sub-5 Å resolution structure determinations using the 300 kV microscope, layers of the of 11 nm 3HB spacer and 60 nm SAH spacer are required on the paramagnetic nanobeads (Test 1, 3, and 5). For the sub-5 Å resolution structure determinations using the 200 kV microscope, the inner layers with the 11 nm 3HB spacer and mono-SPYtag avidin tetramer can be omitted because the noise signals are weaker in the 200 kV microscope then that in 300 kV microscope (Test 2 and 3). (B) Purified proteins for assembling the MagIC-cryo-EM beads. SDS-PAGE analysis was done by applying samples to 4–20% Criterion TGX Precast Midi Protein Gel (Bio-Rad 5671095) and ran at 200 V for 40 min. GelCode Blue stained gel is shown.
-
Figure 2—figure supplement 1—source data 1
The full image of gel shown in Figure 2—figure supplement 1B.
- https://cdn.elifesciences.org/articles/103486/elife-103486-fig2-figsupp1-data1-v1.pdf
-
Figure 2—figure supplement 1—source data 2
The raw image of gel shown in Figure 2—figure supplement 1.
- https://cdn.elifesciences.org/articles/103486/elife-103486-fig2-figsupp1-data2-v1.zip
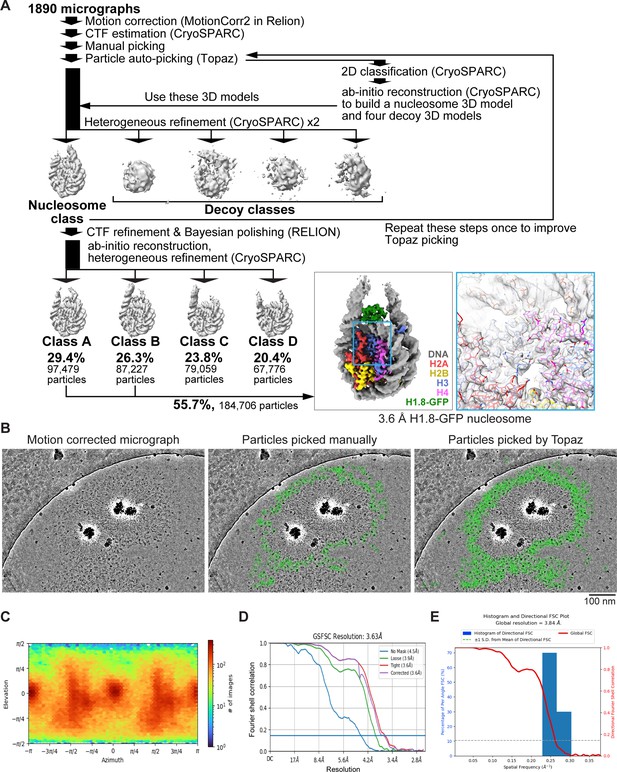
MagIC-cryo-EM single particle analysis of in vitro reconstituted H1.8-GFP-bound nucleosome.
(A) The single particle analysis pipeline for the MagIC-cryo-EM of the in vitro reconstituted H1.8-GFP-bound nucleosomes. Non-nucleosome or noisy particles were removed by heterogeneous refinement with decoy 3D classes (decoy classification). Using the particles assigned to the nucleosome class, another round of heterogeneous refinement was performed to isolate the classes with apparent H1.8 densities. The particles assigned to the classes A and B were mixed, and the 3D structure of the H1.8-GFP-bound nucleosome was determined at 3.63 Å resolution. (B) Comparison between manually picked particles used to train Topaz (middle panel) and the particles picked by Topaz (right panel). Green circles indicate the picked particles. (C) Particle orientation of cryo-EM structure of the H1.8-GFP-bound nucleosome. (D) Gold-standard Fourier Shell Correlation (FSC) curve of the H1.8-GFP nucleosome. The final resolutions of the cryo-EM maps were determined by the gold-standard with a threshold of 0.143. (E) 3D FSC curve of the H1.8-GFP nucleosome.
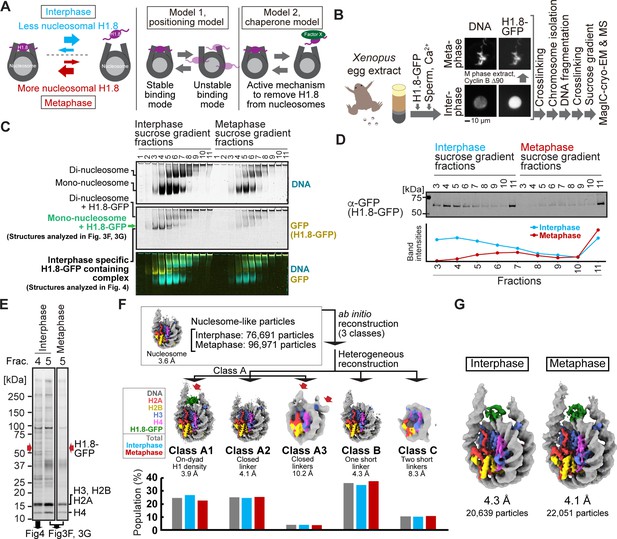
MagIC-Cryo-EM structural determination of H1.8-bound nucleosomes from interphase and metaphase chromosomes in Xenopus egg extract.
(A) Models of potential cell cycle-dependent H1.8 dynamic binding mechanisms (B) Experimental flow of MagIC-cryo-EM analysis for GFP-H1.8 containing complexes isolated from chromosomes assembled in interphase and metaphase Xenopus egg extract. Fluorescence microscopy images indicate localization of GFP-H1.8 to interphase and metaphase chromosomes. DNA and GFP-H1.8 were detected either by staining with Hoechst 33342 or GFP fluorescence, respectively. (C) Native PAGE of fragmented interphase and metaphase chromosome sucrose gradient fractions. GFP-H1.8 and DNA were detected with either GFP fluorescence or SYTO-60 staining, respectively. (D) Western blot of GFP-H1.8 in interphase and metaphase chromosome sucrose gradient fractions. GFP-H1.8 was detected using anti-GFP antibodies. (E) SDS-PAGE of the sucrose gradient fractions 4 and 5 shown in (C), demonstrating heterogeneity of the samples. Proteins were stained by gel code blue. Red arrows indicate the H1.8-GFP bands. The full gel image is shown in Figure 3—figure supplement 2A. (F) In silico 3D classification of interphase and metaphase H1.8-bound nucleosomes isolated from chromosomes in Xenopus egg extract. To assess the structural variations and their population of H1.8-bound nucleosomes, ab initio reconstruction and heterogenous reconstruction were employed twice for the nucleosome-like particles isolated by the decoy classification. The initial round of ab initio reconstruction and heterogenous reconstruction classified the particles into three nucleosome-containing 3D models (A, B, C). Subsequent ab initio reconstruction and heterogenous reconstruction on the class A, which has weak H1.8 density, yielded three new nucleosome-containing structures, A1, A2, and A3. 3D maps represent the structural variants of GFP-H1.8-bound nucleosomes. Red arrows indicate extra densities that may represent H1.8. Green densities indicate on-dyad H1.8. The bar graphs indicate the population of the particles assigned to each 3D class in both interphase and metaphase particles (gray), interphase particles (blue), and metaphase particles (red). The pipeline for structural analysis is shown in Figure 3—figure supplement 3A. (G) Structures of H1.8-bound nucleosomes isolated from interphase and metaphase chromosomes.
-
Figure 3—source data 1
Full images of gels and membranes shown in Figure 3.
- https://cdn.elifesciences.org/articles/103486/elife-103486-fig3-data1-v1.pdf
-
Figure 3—source data 2
Raw images of gels and membranes shown in Figure 3.
- https://cdn.elifesciences.org/articles/103486/elife-103486-fig3-data2-v1.zip
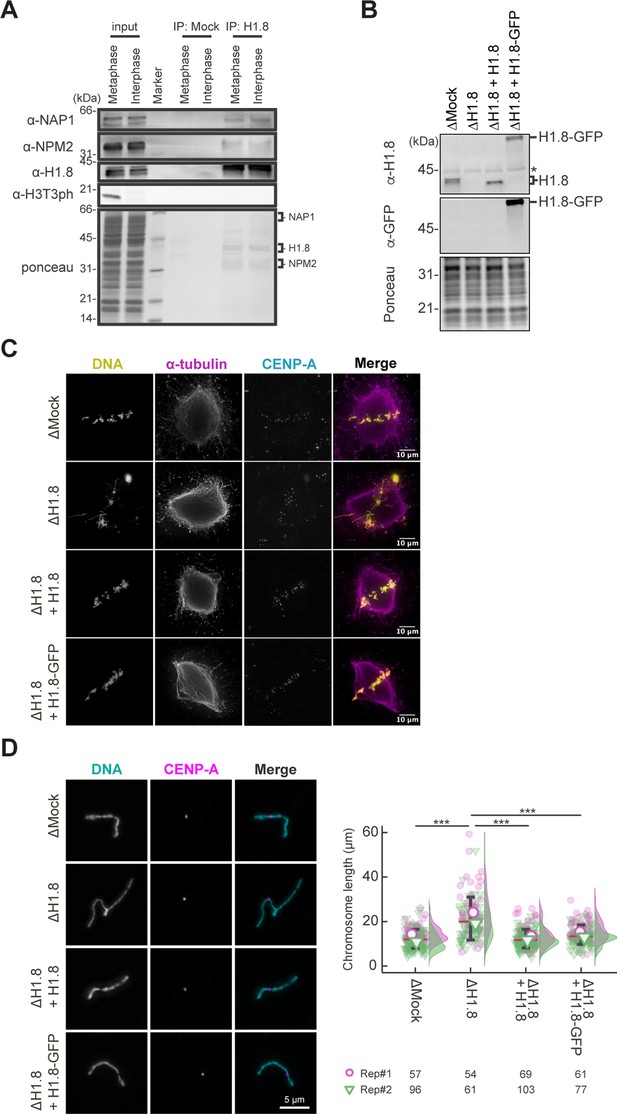
Functional assessment of H1.8-GFP in Xenopus egg extract.
(A) Cell-cycle-independent H1.8 binding to NAP1 and NPM2 in the Xenopus egg cytoplasm. H1.8 was immunoprecipitated from Xenopus CSF (metaphase) extracts or interphase extracts and analyzed by western blotting. Antibodies against phosphorylated histone H3 Thr3 H3T3ph were used as a marker for M phase. Amounts of NAP1 and NPM2 co-immunoprecipitated with anti-H1.8 antibodies did not change between metaphase and interphase. An example of two reproducible results is shown. (B) Western blots to show the depletion efficiency of the endogenous H1.8 and complementation of recombinant non-tag H1.8 and H1.8-GFP in Xenopus egg extract. The asterisk indicates a non-specific cross-reacting band. (C) Representative fluorescence images of metaphase chromosomes with spindles in Mock- (∆Mock), endogenous H1.8-depleted Xenopus egg extract (∆H1.8), and recombinant H1.8 or recombinant H1.8-GFP supplemented endogenous H1.8-depleted Xenopus egg extracts (∆H1.8+H1.8 or ∆H1.8+H1.8 GFP, respectively). Misaligned metaphase chromosome phenotype caused by H1.8 depletion was rescued in H1.8 or H1.8-GFP supplemented Xenopus egg extracts. (D) Left; representative fluorescence images of individualized metaphase chromosomes. Elongated chromosome morphology caused by H1.8 depletion was rescued in H1.8 or H1.8-GFP supplemented Xenopus egg extracts. Scale bar, 5 µm. Right; quantification of chromosome length visualized by SuperPlots. Data distribution of the length of each individual chromosome from two biological replicates (purple and green) is shown as jitter plot with half violin plot. Each mark (purple open circle and green open inverted triangle) represents the average length of chromosomes from a single replicate. Bar represents median (red) and SD (black) of two biological replicates. Given the result that dataset is not normal distribution, confirmed by Shapiro-Wilk normality test, each p-value was calculated using Welch’s t-test. ***, p<0.001. The number of individualized chromosomes analyzed in each condition for each replicate is indicated at the bottom of the figure.
-
Figure 3—figure supplement 1—source data 1
Full images of gels and membranes shown in Figure 3—figure supplement 1.
(A) Full gel images used in Figure 3—figure supplement 1A. (B) Full gel image used in Figure 3—figure supplement 1B.
- https://cdn.elifesciences.org/articles/103486/elife-103486-fig3-figsupp1-data1-v1.pdf
-
Figure 3—figure supplement 1—source data 2
Raw images of gels and membranes shown in Figure 3—figure supplement 1.
- https://cdn.elifesciences.org/articles/103486/elife-103486-fig3-figsupp1-data2-v1.zip
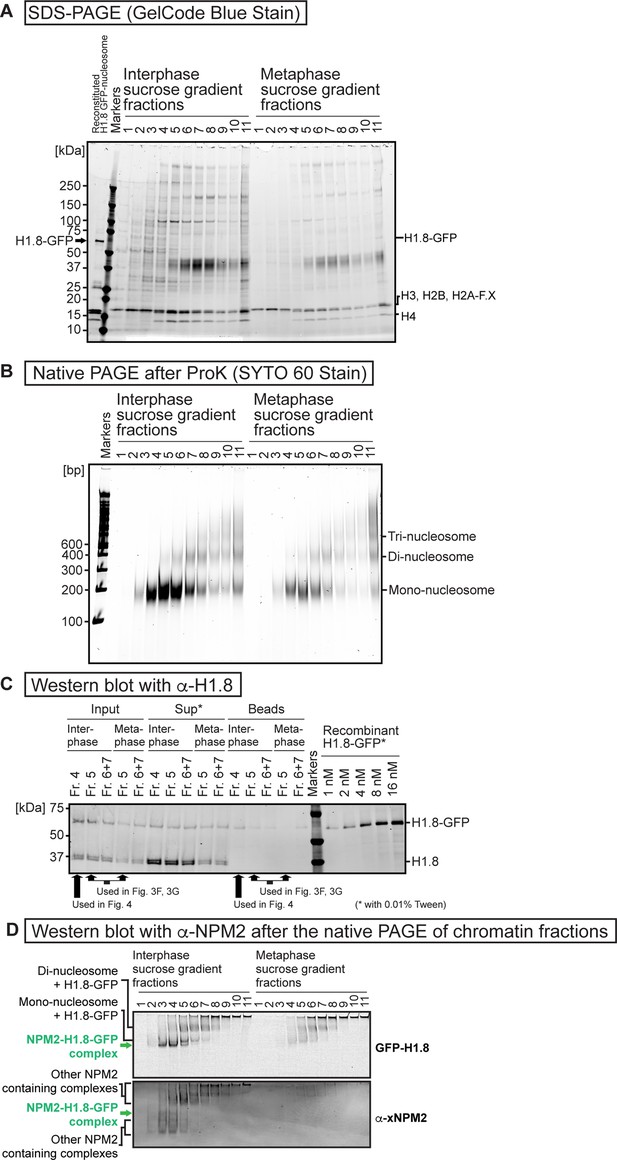
Sucrose gradient fractions of the fragmented interphase and metaphase chromosomes formed in Xenopus egg extract.
(A) Sucrose density gradient centrifugation fractionations of MNase-treated interphase and metaphase chromosomes formed in Xenopus egg extract. SDS-PAGE analysis was done by applying 15 µL samples to 4–20% Criterion TGX Precast Midi Protein Gel (Bio-Rad 5671095) and ran at 200 V for 40 min. GelCode Blue stained gel is shown. (B) Native PAGE analysis of DNA lengths of the sucrose gradient fractions shown in A. 15 µL samples were treated with protease K and RNaseA and applied to 6% native PAGE gel with x0.5 TBE and ran at 150 V for 80 min. (C) Western blot of the sucrose gradient fractions used for the MagIC-cryo-EM. H1.8-GFP and endogenous H1.8 were detected by antibody for H1.8. ‘Input’ lanes indicate the sucrose gradient fractions before isolating H1.8-GFP-containing complexes with MagIC-cryo-beads. ‘Sup’ lanes indicate the unbound fractions after the isolation by MagIC-cryo-beads. ‘Beads’ lanes indicate the MagIC-cryo-beads isolating H1.8-GFP-containing complexes. ‘Recombinant H1.8-GFP’ lanes indicate purified H1.8-GFP with known concentrations. The samples for ‘Sup’ and ‘Recombinant H1.8-GFP’ lanes contained 0.01% Tween20, which increased the western blot signals presumably by reducing the sample absorption to the tubes and tips side wall. (D) Comparison of the H1-GFP and NPM2 containing bands in native PAGE of the sucrose density gradient centrifugation fractionation of MNase-treated interphase and metaphase chromosomes formed in Xenopus egg extract. H1-GFP was detected by GFP fluorescence (identical image with Figure 3C). NPM2 was detected by an anti-NPM2 antibody through western blotting, following protein transfer from the native PAGE gel to the membrane.
-
Figure 3—figure supplement 2—source data 1
Full images of gels and membranes shown in Figure 3—figure supplement 2.
(A) Full gel images used in Figure 3—figure supplement 2A. (B) Full gel image used in Figure 3—figure supplement 2B. (C) Full membrane image used in Figure 3—figure supplement 2C. (D) Full membrane images used in Figure 3—figure supplement 2D.
- https://cdn.elifesciences.org/articles/103486/elife-103486-fig3-figsupp2-data1-v1.pdf
-
Figure 3—figure supplement 2—source data 2
Raw images of gels and membranes shown in Figure 3—figure supplement 2.
- https://cdn.elifesciences.org/articles/103486/elife-103486-fig3-figsupp2-data2-v1.zip
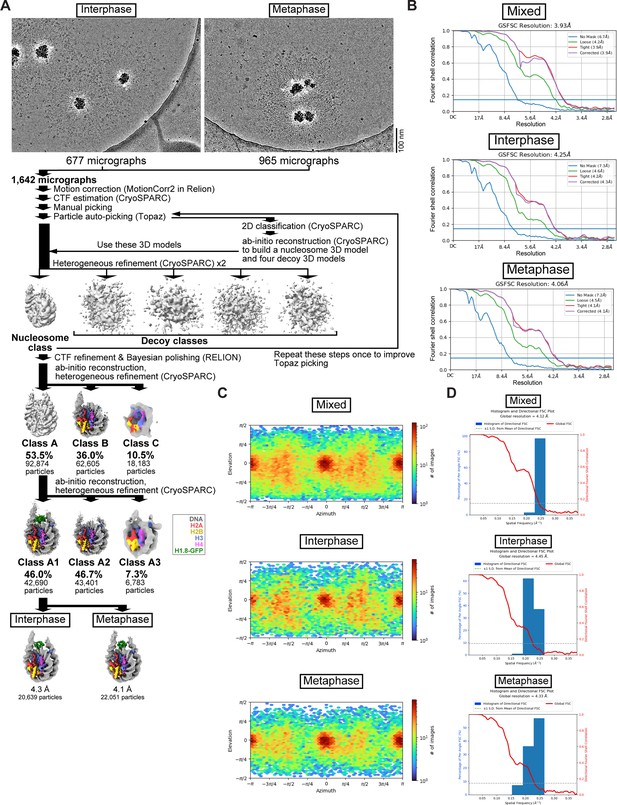
Single-particle analysis pipeline for the MagIC-cryo-EM of the interphase and metaphase H1.8-GFP-bound nucleosomes formed in Xenopus egg extract.
Related to Figure 3. (A) Single-particle analysis and in silico mixing 3D classification pipeline for the MagIC-cryo-EM. (B) Gold-standard FSC curves of the interphase and metaphase H1.8-GFP-bound nucleosomes. The final resolutions of the cryo-EM maps were determined by the gold-standard with a threshold of 0.143 (C) Particle orientation of cryo-EM structure of the interphase and metaphase H1.8-GFP-bound nucleosomes. (D) 3D FSC of the interphase and metaphase H1.8-GFP-bound nucleosomes.
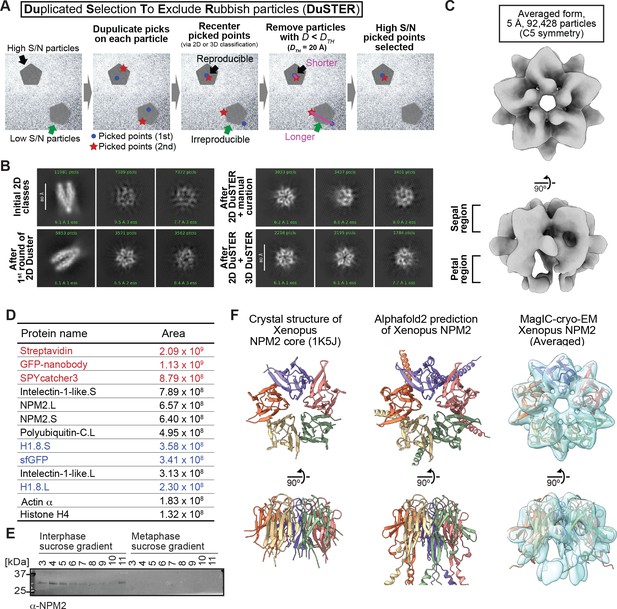
MagIC-cryo-EM and DuSTER reconstructed cryo-EM structures of interphase-specific H1.8-bound NPM2.
(A) Schematic of DuSTER workflow. (B) 2D classes before and after particle curation with DuSTER. More 2D classes are shown in Figure 4—figure supplement 5B–E. (C) 3D cryo-EM structure of interphase-specific H1.8-containing complex. C5 symmetry was applied during structural reconstruction. The complete pipeline is shown in Figure 4—figure supplement 3, Figure 4—figure supplement 5, and Figure 4—figure supplement 6. (D) MS identification of proteins that cofractionated with H1.8 in sucrose gradient fraction 4 from interphase chromosomes shown in Figure 3C. Portions of MagIC-cryo-EM beads prepared for cryo-EM were subjected to MS. Proteins shown in red are the proteins that comprise the GPF nanobody-MagIC-cryo-EM beads. Proteins shown in blue represent signals from H1.8-GFP. (E) Western blot of NPM2 in the sucrose gradient fractions of interphase and metaphase chromosome fragments. (F) The structural comparison of the crystal structure of the pentameric NPM2 core (PDB ID: 1K5J), and AF2 predicted structure of the pentameric NPM2 core, and MagIC-cryo-EM structures of NPM2-H1.8. The MagIC-cryo-EM structures indicate NPM2 in the NPM2-H1.8 complex forms pentamer.
-
Figure 4—source data 1
The full image of the membrane shown in Figure 3E.
- https://cdn.elifesciences.org/articles/103486/elife-103486-fig4-data1-v1.pdf
-
Figure 4—source data 2
The raw image of the membrane shown in Figure 3E.
- https://cdn.elifesciences.org/articles/103486/elife-103486-fig4-data2-v1.zip
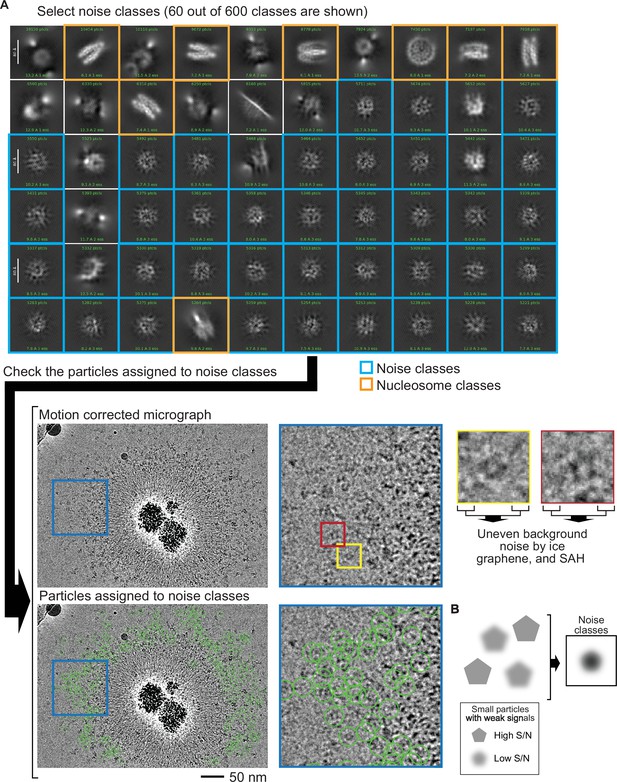
The fraction with the interphase-specific GFP-H1.8 containing complex had many particles with low S/N by cryo-EM.
(A) The sucrose gradient fraction enriched with interphase-specific GFP-H1.8 containing complex (fraction 4 in Figure 3C) was subjected for MagIC-cryo-EM analysis. Initial 2D classification based on particles picked by Topaz generated only noise 2D classes (outlined with blue in the top panel) beside obvious nucleosome classes (outlined with orange in the top). Although 2D classes seem noisy, many of the original pick points marked apparent protein particles on the original motion-corrected micrograph (bottom). This suggests that these particle images were not properly aligned during 2D classification due to the low S/N of these particles. Uneven background noises were likely generated by uneven ice thickness, graphene and the SAH spacer proteins on MagIC-cryo-EM beads. (B) Graphical presentation of how noise 2D classes were generated. Although Topaz picked target protein particles on micrographs, many small target particles do not have strong enough S/N to be properly aligned during 2D classification.

Reproducible particle centering after 2D classification as a criterion for particles with high S/N.
The sucrose gradient fraction enriched with interphase-specific GFP-H1.8 containing complex (fraction 4 in Figure 3C) was isolated and analyzed by MagIC-cryo-EM. The initial particle locations assigned by particle picking software are updated during 2D to align the multiple images on a 2D map and place the reconstituted 2D map at the center of the reconstituted 2D space. To assess the reproducibility of particle centering during 2D classification, particle picking was repeated and subjected to the 2D classification individually. Particle images were sorted based on the distance D between a recentered picked point from the first picking set and another re-centered picked point from the second picking set. The sorted particle images were again applied to 2D classification. Particle images with D>20 Å, which were not reproducibly recentered, generated noise 2D classes. In contrast, particle images with D≤20 Å, which were reproducibly recentered, generated 2D classes with less background noises.
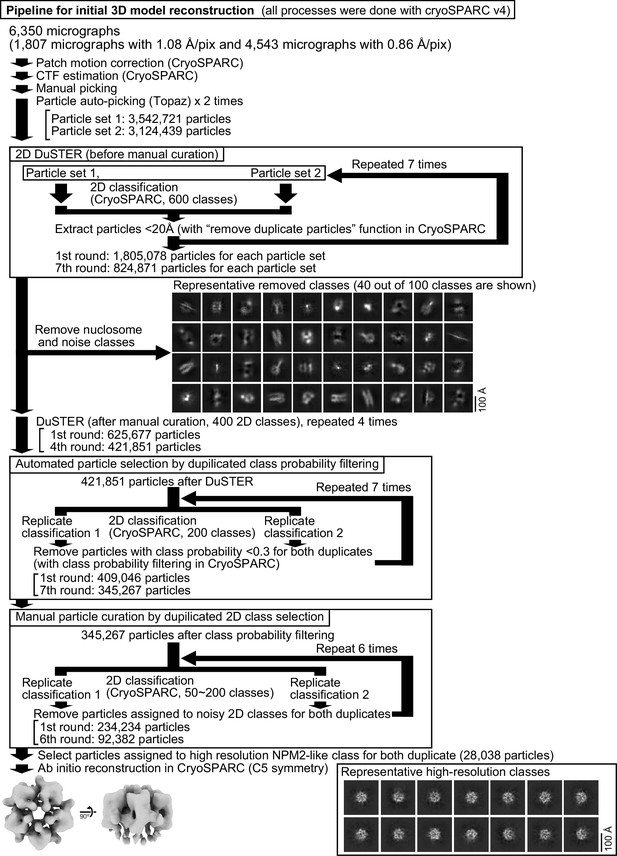
Pipeline for 2D DuSTER for reconstructing a 3D initial model of the interphase-specific H1.8-containing complex (NPM2-H1.8) that is used as a template of 3D DuSTER.
Please refer to the Materials and methods section for a detailed description.
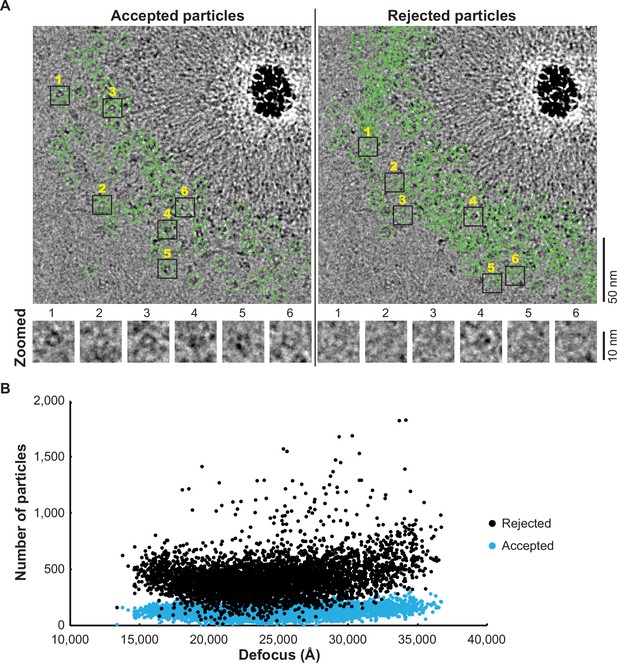
The particles selected by DuSTER curation.
(A) The particles accepted and rejected by DuSTER. Particles are mapped on motion-corrected micrographs. (B) DuSTER accepted particles across all defocus ranges. Numbers of accepted (cyan) and rejected (black) particles on each micrograph and their defocus length are plotted.
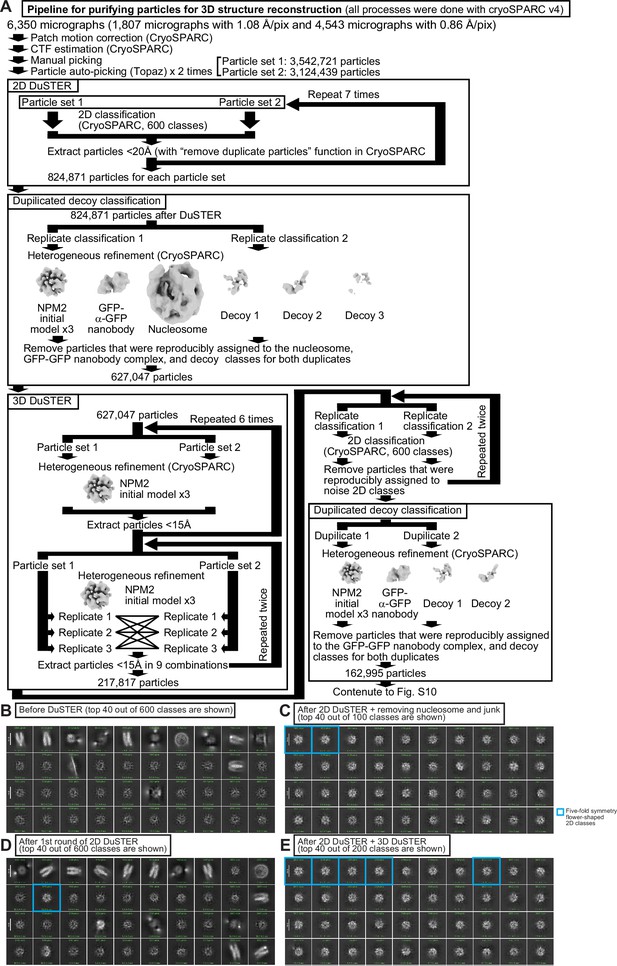
Pipeline for 3D DuSTER.
(A) Pipeline for 3D DuSTER. Please refer to the materials and methods section for a detailed description. (B) A 2D classification result of the particles picked by Topaz without particle curation with DuSTER. Beside obvious nucleosome classes, no reasonable 2D classes were observed before the DuSTER curation. (C) 2D classification of the particle after the single round of 2D DuSTER. Fivefold symmetry flower-shaped 2D classes (outlined with cyan) are observed. (D) 2D classification of the particles after seven rounds of 2D DuSTER and manual curation of non-target complex classes. (E) 2D classification of the particles after the completion of 2D DuSTER and 3D DuSTER.
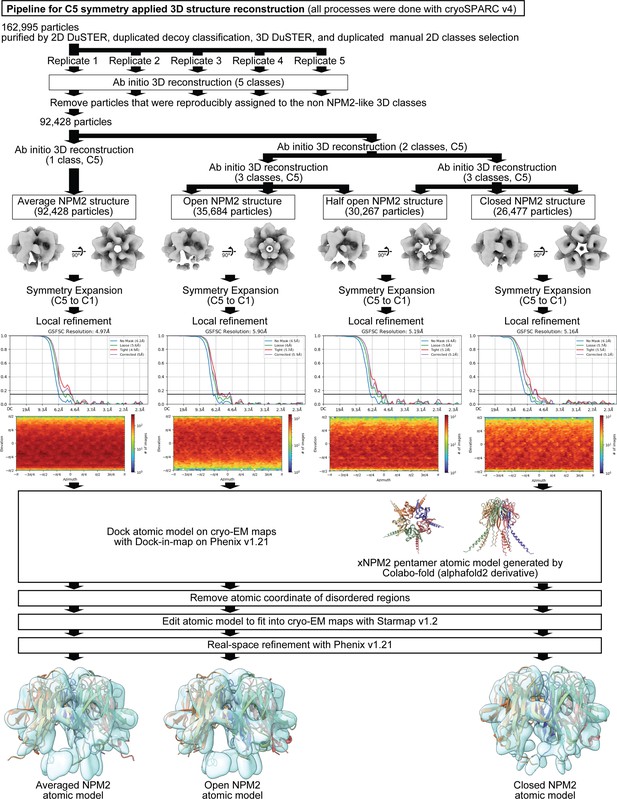
Pipeline for 3D structure determination of the interphase-specific H1.8-containing complex (NPM2-H1.8).
Please refer to the Materials and methods section for a detailed description.
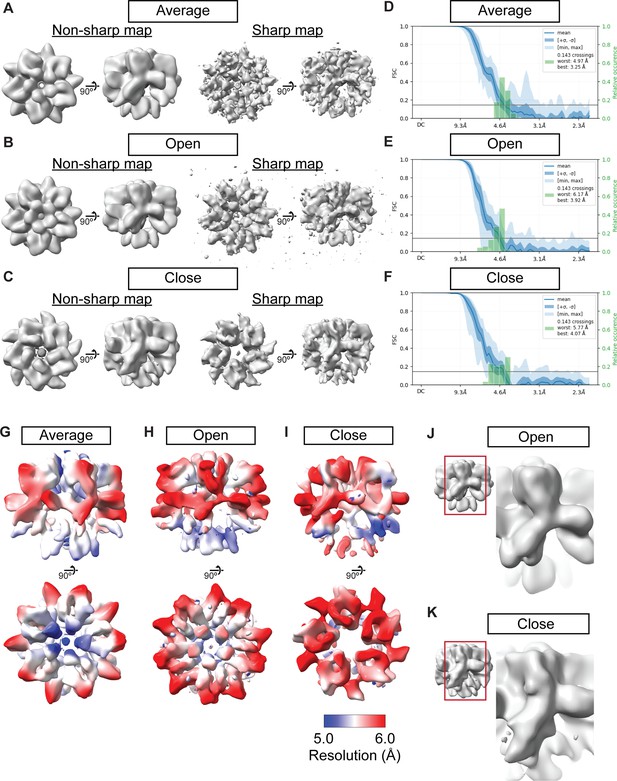
Cryo-EM maps of NPM2 co-isolated with H1.8.
(A–C) The NPM2 cryo-EM maps before and after the map sharpening. (D–F) 3D FSC of the NPM2 cryo-EM maps (G–I) Local resolution of NPM2 cryo-EM maps. (J–K) The structural comparison of the open and closed form NPM2 cryo-EM maps.
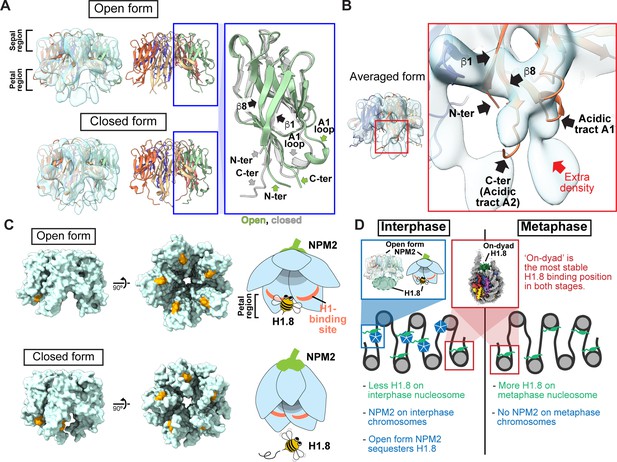
Structural variations of NPM2 bound to H1.8.
(A) Structural differences between the opened and closed forms of NPM2. Left panels show cryo-EM maps of the opened and closed forms of NPM2 with H1.8. Middle panels show the atomic models. The right panel shows the zoomed-in view of the open form (green) and closed form (gray) of the NPM2 protomer. In the closed form, β8 runs straight from the sepal side to the petal side. In the open form, the C-terminal portion of β8 is bent outward to the rim. (B) Putative H1.8 density (red arrow) in the averaged NPM2-H1.8 structure. (C) The NPM2 surface that contacts the putative H1.8 density (corresponding to aa 42–44) is shown in orange. The surface structures were generated from atomic models. The H1.8-binding sites are accessible in the open form while they are internalized in the closed form. Note that C-terminal acidic tracts A2 and A3 (Figure 5—figure supplement 1A) are not visible in the cryo-EM structure but are likely to contribute to H1.8 binding as well in both open and closed forms. (D) Model of the mechanism that regulates the amount of the H1.8 in interphase and metaphase nucleosome.
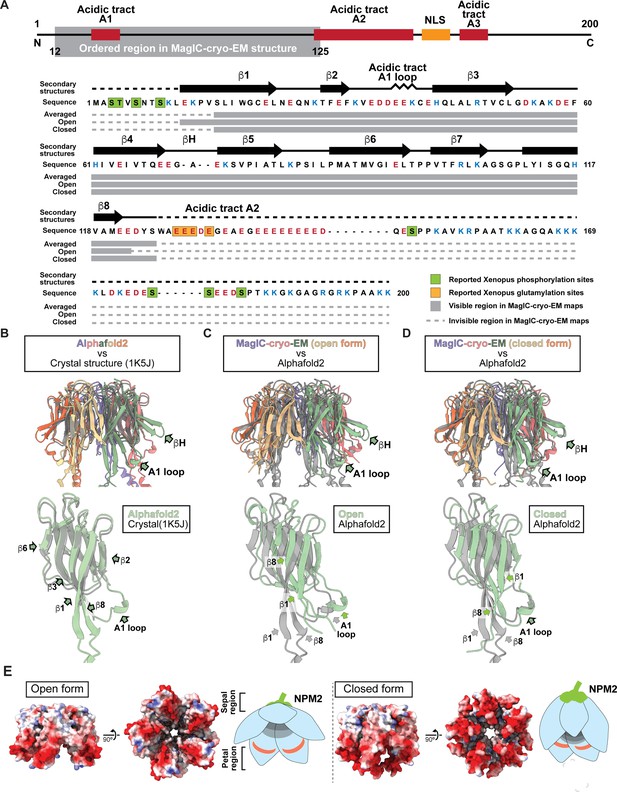
Cryo-EM maps and atomic models of NPM2 co-isolated with H1.8.
(A) The secondary structures of the atomic models of open and closed NPM2. (B-D) The structural comparison of the crystal structure of the pentameric NPM2 core (PDB ID: 1K5J) (B), and AF2 predicted structure of the pentameric NPM2 core (C), and MagIC-cryo-EM structures of NPM2-H1.8 (D). The MagIC-cryo-EM structures indicate NPM2 in the NPM2-H1.8 complex forms pentamer. (E) The surface electropotential of open and closed NPM2 atomic models.
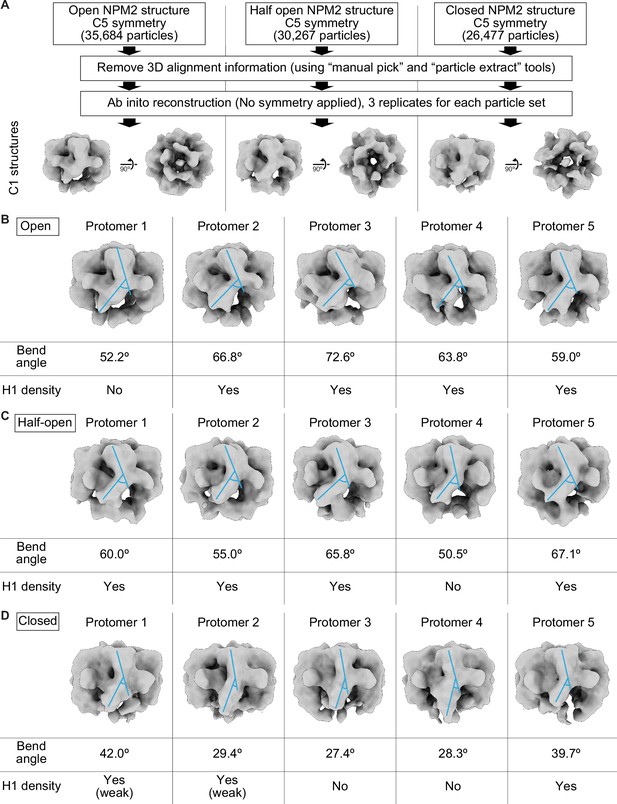
Asymmetric structures of NPM2 co-isolated with H1.8 without applying C5 symmetry.
(A) Pipeline to reconstitute C1 symmetry NPM2-H1.8 structures. (B–D) Structural features of the open (B), half open (C), and closed (D) NPM2-H1.8 structures without applying C5 symmetry. Blue lines indicate the angles of the sepal and petal domains used for measuring the bend angles of each protomer. The bending is critical in forming the open-form NPM2 structures and increases the accessibility of the H1-binding sites. The bend angles and the bindings of the H1 densities are not consistent in each protomer, suggesting that NPM2 protomers with various openness co-exist in a single NPM2-H1.8 complex.
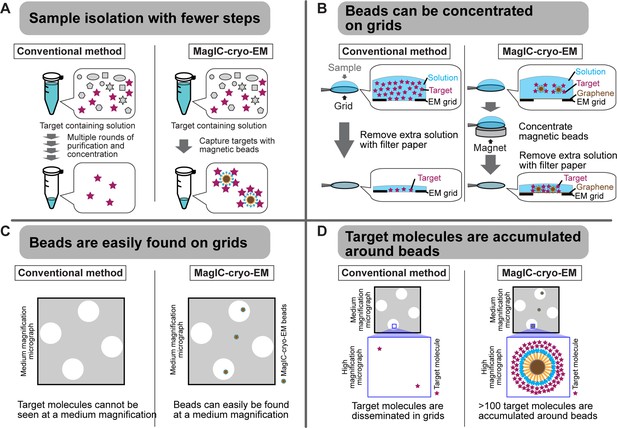
Advantages of MagIC-cryo-EM over conventional cryo-EM methods.
(A) The on-bead-cryo-EM approach reduces preparation steps (e.g. target isolation, enrichment, and buffer exchange), which can lead to sample loss. (B) Sample loss during the grid-freezing process is reduced by magnet-based enrichment of the targets on cryo-EM grids. (C) The magnetic beads are easily identified in medium -magnification montage maps, enabling the selection of areas where targets exist prior to high-magnification data collection. (D) Targets are highly concentrated around the beads, ensuring that each micrograph contains more than 100 usable particles for 3D structure determination.
Tables
The required sample amount for analyzing chromatin samples.
Methods | Purity | Concentration | Volume | Amount | Advantage | Disadvantage |
---|---|---|---|---|---|---|
Cryo-EM (conventional) | Purified | 0.5~5.0 mg/mL | 3~4 µL/grid | >10 µg/sample | simple | High concentration of sample is required |
Cryo-EM (Jet vitrification) | Purified | 4 mg/mL | 0.001 µL/grid | >4 ng/sample | Very low volume of sample is required | High concentration of sample is required |
Cryo-EM (Affinity grid) | Crude | 0.05 mg/mL | 3~4 µL/grid | >1 µg/sample | Sample can be isolated and concentrated on grid | The maximum sample volume is limited |
ChIP-seq | Crude | - | - | 10~50 ng DNA | Sample can be isolated and concentrated by beads | |
SDS-PAGE (CBB stain) | Crude | 0.005~0.100 mg/mL | 1~20 µL/lane | >30 ng/band | ||
MagIC-cryo-EM | Crude | <0.0005 mg/mL | 1~2000 µL | >5 ng (2 ng DNA) /grid | Sample can be isolated and concentrated by beads | cryo-EM data collection points are selected manually. |
SDS-PAGE (Silver stain) | Crude | 0.0001~0.001 mg/mL | 1~20 µL/lane | >1 ng/band |
The list of the chromatin proteins detected by mass spectrometry (MS) before and after enrichment on the MagIC-cryo-EM-beads.
The MS analysis was conducted to the sucrose gradient fractions 5, before (Figure 3C) and after (Figure 3E) enrichment with the GPF nanobody-MagIC-cryo-EM beads. Detectable MS signals for known chromatin proteins and the recombinant proteins used for assembling the MagIC-cryo-EM beads were manually selected and are listed here. See Supplementary file 2 of the full MS data.
Description | Full name or functions | Input interphase, fraction 5 | Input metaphase, fraction 5 | MagIC-cryo-EM, interphase, fraction 5 | MagIC-cryo-EM, metaphase, fraction 5 |
---|---|---|---|---|---|
XBmRNA11963| h2ac17.L | histone H2A | 1.37x1010 | 4.12x109 | 2.08x108 | 1.49x108 |
XBmRNA31731| LOC121402261 | histone H2B | 7.87x109 | 2.26x109 | 1.10x108 | 2.25x108 |
XBmRNA31368| LOC121402047 | histone H4 | 7.34x109 | 2.00x109 | 1.11x108 | 1.63x108 |
XBmRNA36987| h2aj.L | histone H2A.J | 6.74x109 | 3.64x109 | 2.44x108 | 1.29x108 |
XBmRNA58735| h2ax.2.L | histone H2A.X | 6.56x109 | 1.73x109 | 2.44x108 | 1.75x108 |
XBmRNA1949| h2az1.L | histone H2A.Z | 4.50x109 | 3.64x109 | 2.44x108 | 1.52x108 |
XBmRNA75391| h3-3b.L | histone H3.3 | 3.33x109 | 1.19x109 | 3.84x107 | 5.29x107 |
XBmRNA25971| npm2.L | Nucleoplasmin | 1.77x109 | n.d. | 1.89x108 | n.d. |
XBmRNA28658| npm2.S | Nucleoplasmin | 1.76x109 | n.d. | 1.31x108 | n.d. |
XBmRNA25690| pcna.L | PCNA | 1.29x109 | n.d. | n.d. | n.d. |
XBmRNA28883| pcna.S | PCNA | 1.29x109 | n.d. | n.d. | n.d. |
XBmRNA41314| h1-8.S | Linker histone H1.8 | 7.12x108 | 1.93x108 | 5.67x107 | 6.87x107 |
XBmRNA9392| supt16h.S | supt16h (histone chaperone FACT complex component) | 3.14x108 | n.d. | n.d. | n.d. |
XBmRNA4198| supt16h.L | supt16h (histone chaperone FACT complex component) | 3.04x108 | n.d. | n.d. | n.d. |
XBmRNA62381| ssrp1.S | ssrp1 (histone chaperone FACT complex component) | 2.96x108 | n.d. | n.d. | n.d. |
sfGFP | sfGFP (Tagged with H1.8) | 2.84x108 | 8.15x107 | 6.02x107 | 4.58x107 |
XBmRNA26658| dnmt1.L | DNA methyltransferase 1 | 1.41x108 | n.d. | n.d. | n.d. |
XBmRNA31618| dnmt1.S | DNA methyltransferase 1 | 1.35x108 | n.d. | n.d. | n.d. |
XBmRNA5952| sub1.L | Activated RNA polymerase II transcriptional coactivator p15 | 8.60x107 | n.d. | n.d. | n.d. |
XBmRNA24726| pclaf.L | PCNA-associated factor | 8.38x107 | n.d. | n.d. | n.d. |
XBmRNA4881| ran.L | GTP-binding nuclear protein Ran | 7.38x107 | n.d. | n.d. | n.d. |
XBmRNA42021| msh2.L | DNA repair protein MutS | 7.08x107 | n.d. | n.d. | n.d. |
XBmRNA52420| mcm4.L | DNA replication licensing factor MCM4 | 6.61x107 | n.d. | n.d. | n.d. |
XBmRNA51197| mcm6.L | DNA replication licensing factor MCM6 | 6.54x107 | n.d. | n.d. | n.d. |
XBmRNA60815| rcc2.L | Regulator of chromosome condensation 2 | 6.29x107 | n.d. | n.d. | n.d. |
XBmRNA29645| pclaf.S | PCNA-associated factor | 5.26x107 | n.d. | n.d. | n.d. |
XBmRNA12919| rcc1.L | Regulator of chromosome condensation 1 | 5.24x107 | n.d. | n.d. | n.d. |
XBmRNA27060| mcm7.L | DNA replication licensing factor MCM7 | 5.08x107 | n.d. | n.d. | n.d. |
XBmRNA73621| LOC108700788 | Importin subunit beta-like isoform X2 | 5.03x107 | n.d. | n.d. | n.d. |
XBmRNA42017| msh6.L | DNA repair protein MutS | 4.96x107 | n.d. | n.d. | n.d. |
XBmRNA39469| top1.2.S | DNA topoisomerase I | 4.92x107 | n.d. | n.d. | n.d. |
XBmRNA82329| kpna7.S | Importin subunit beta | 4.80x107 | n.d. | n.d. | n.d. |
XBmRNA74418| csnk2a1.L | Casein kinase II subunit alpha | 4.65x107 | n.d. | n.d. | n.d. |
XBmRNA25938| XB5867546.L | Nucleoplasmin isoform (lacking C-ter tail) | 4.31x107 | n.d. | n.d. | n.d. |
XBmRNA7871| uhrf1.S | E3 ubiquitin-protein ligase UHRF1 | 4.27x107 | n.d. | n.d. | n.d. |
XBmRNA12245| rpa1.L | Replication protein A | 4.10x107 | n.d. | n.d. | n.d. |
XBmRNA41384| LOC100192369 | Sperm-specific nuclear basic protein 1 | 4.08x107 | n.d. | n.d. | n.d. |
XBmRNA52377| mcm3.L | DNA replication licensing factor MCM3 | 4.04x107 | n.d. | n.d. | n.d. |
XBmRNA36255| mcm5.L | DNA replication licensing factor MCM5 | 3.86x107 | n.d. | n.d. | n.d. |
XBmRNA36962| mcm2.L | DNA replication licensing factor MCM2 | 3.73x107 | n.d. | n.d. | n.d. |
XBmRNA60207| pold1.L | DNA polymerase | 3.63x107 | n.d. | n.d. | n.d. |
XBmRNA33152| fen1.L | Flap endonuclease 1 A | 3.59x107 | n.d. | n.d. | n.d. |
XBmRNA25831| pold2.L | DNA polymerase delta subunit 2 | 3.37x107 | n.d. | n.d. | n.d. |
XBmRNA38234| ddb1.S | DNA damage-binding protein 1 | 2.98x107 | n.d. | n.d. | n.d. |
XBmRNA78857| dppa2.L | Developmental pluripotency associated 2 | 2.96x107 | n.d. | n.d. | n.d. |
XBmRNA34892| gins3.L | DNA replication complex GINS protein PSF3 | 2.93x107 | n.d. | n.d. | n.d. |
XBmRNA83211| hirip3.S | HIRA-interacting protein 3 | 2.69x107 | n.d. | n.d. | n.d. |
XBmRNA35380| nasp.L | histone chaperone NASP | 1.84x107 | n.d. | n.d. | n.d. |
XBmRNA23497| hmga2.L | High mobility group AT-hook 2 | 1.45x107 | n.d. | n.d. | n.d. |
XBmRNA30566| hmga2.S | High mobility group AT-hook 2 | 1.43x107 | n.d. | n.d. | n.d. |
XBmRNA33324| pola2.L | DNA polymerase alpha subunit B | 1.10x107 | n.d. | n.d. | n.d. |
Streptavidin | MagIC-cryo-EM beads proteins | n.d. | n.d. | 1.22x109 | 2.19x109 |
SPY-tagGFPnanobody | MagIC-cryo-EM beads proteins | n.d. | n.d. | 7.69x108 | 1.41x109 |
Spycatcher3 | MagIC-cryo-EM beads proteins | n.d. | n.d. | 5.99x108 | 7.82x108 |
11 nm_3HB | MagIC-cryo-EM beads proteins | n.d. | n.d. | 4.32x107 | 5.90x107 |
60 nm_SAH | MagIC-cryo-EM beads proteins | n.d. | n.d. | 1.21x107 | 7.73x106 |
Expected mass of the NPM2-H1.8-GFP complex.
Sucrose gradient elution volume indicates that the NPM2-H1.8-GFP complex is smaller than mono-nucleosome (around 230 kDa). Only the NMP2 pentamer complexed H1.8-GFP monomer (166 kDa) reasonably explains the sucrose gradient result.
Name | Molecular wight (Da) |
---|---|
NPM2 monomer | 21,917 |
NPM2 pentamer | 109,587 |
NPM2 decamer | 219,175 |
H1.8-GFP | 56,704 |
NPM2 pentamer +H1.8 GFP monomer | 166,291 |
NPM2 pentamer +H1.8 GFP pentamer | 393,107 |
NPM2 decamer +H1.8 GFP monomer | 275,878 |
NPM2 decamer +H1.8 GFP pentamer | 502,694 |
Nucleosome (193 bp DNA) | 228,111 |
Reagent type (species) or resource | Designation | Source or reference | Identifiers | Additional information |
---|---|---|---|---|
Antibody | Anti-histone H1.8 (Xenopus laevis) Rabbit polyclonal antibody | Jenness et al., 2018 | RU2130 | 1 µg/mL |
Antibody | Anti-histone H3T3ph mouse monoclonal antibody | Kelly et al., 2008 | 16B2 | 1:5000 dilution |
Antibody | IRDye 680LT goat anti-rabbit IgG | LI-COR | 926–68021; RRID:AB_10706309 | 1:15,000 dilution |
Antibody | IR Dye 800CW goat anti-mouse IgG | LI-COR | 926–32210; RRID:AB_621842 | 1:10,000 dilution |
Antibody | Anti-histone NAP1 (Xenopus laevis) Rabbit polyclonal antibody | Lorton et al., 2023 | N/A | 1:500 dilution |
Antibody | Anti-histone NPM2 (Xenopus laevis) Rabbit polyclonal antibody | Lorton et al., 2023 | N/A | 1:500 dilution |
Antibody | Anti-rabbit IgG | SIGMA | I5006, RRID:AB_1163659 | |
Antibody | Anti-rabbit IgG (purified from pre-bleed sera) | This work | N/A | |
Antibody | Cy3 AffiniPure F(ab')₂ Fragment Donkey Anti-Mouse IgG (H+L) | Jackson ImmunoResearch | 715-166-150, RRID:AB_2340816 | 1:500 dilution |
Antibody | Cy5 AffiniPure Donkey Anti-Rabbit IgG (H+L) | Jackson ImmunoResearch | 711-175-152, RRID:AB_2340607 | 1:500 dilution |
Strain, strain background (E. coli) | E. coli Rosetta (DE3) | Novagen | 70954–3 | |
Strain, strain background (E. coli) | E. coli BL21(DE3) | Novagen | 69450–3 | |
Strain, strain background (E. coli) | E. coli JM101 | Agilent | 200234 | |
Biological sample (Xenopus laevis) | Xenopus laevis sperm nuclei | Nasco, Isolated from male Xenopus laevis | LM00715 (male Xenopus laevis) | |
Biological sample (Xenopus laevis) | Xenopus laevis egg | Nasco, Laid by female Xenopus laevis | LM00535 (female Xenopus laevis) | |
Biological sample (Xenopus laevis) | Alexa594-labeled-tubulin | Hyman et al., 1991; Isolated from bovine brain | n/a | |
Chemical compound, drug | Chorionic gonadotropin human | Sigma | CG10-1VL | |
Chemical compound, drug | Pregnant Mare Serum Gonadotropin (PMSG) | Prospec | HOR-272 | |
Chemical compound, drug | Cycloheximide | Sigma | C-7698 | |
Chemical compound, drug | CaCl2 | Sigma Aldrich | C7902-500G | |
Peptide, recombinant protein | Cyclin B Δ90 | Glotzer et al., 1991 | N/A | |
Chemical compound, drug | Cysteine | Sigma | C7352-1KG | |
Chemical compound, drug | PIPES | Alfa Aesar | A16090 | |
Chemical compound, drug | NaCl | Millipore Sigma | SX0420-5 | |
Chemical compound, drug | Glycerol | Alfa Aesar | 36646 | |
Chemical compound, drug | EGTA | Sigma Aldrich | E4378-250G | |
Chemical compound, drug | MgCl2 | Acros organic | 197530010 | |
Chemical compound, drug | β-glycerophosphate | Acros organic | 410991000 | |
Chemical compound, drug | Sodium butyrate | Aldrich | 303410–100 G | |
Chemical compound, drug | Formaldehyde | Fisher bioreagents | BP531-25 | |
Chemical compound, drug | HEPES | Akron biotech | AK1069-1000 | |
Chemical compound, drug | Sucrose | Fisher chemical | S5-3 | |
Chemical compound, drug | KCl | Fisher chemical | P217-3 | |
Chemical compound, drug | Spermidine | Sigma | S-2501 | |
Chemical compound, drug | Spermine | Sigma | S1141-5G | |
Chemical compound, drug | cOmplete EDTA-free Protease Inhibitor Cocktail | Roche | 11873580001 | |
Chemical compound, drug | Leupeptin | Millipore Sigma | El8 | |
Chemical compound, drug | Pepstatin | Sigma | P5318-25MG | |
Chemical compound, drug | Chymostatin | Millipore Sigma | El6 | |
Peptide, recombinant protein | RNaseA | Thermo Scientific | EN0531 | |
Peptide, recombinant protein | Proteinase K solution | Roche | 3115828001 | |
Chemical compound, drug | SYBR-safe | Invitrogen | S33102 | |
Chemical compound, drug | 4–20% gradient SDS-PAGE gel | BioRad | 4561096 | |
Chemical compound, drug | SYTO-60 | Invitrogen | S11342 | |
Chemical compound, drug | Tris | Sigma | T1503-5KG | |
Chemical compound, drug | Glycine | Sigma | G7126-5KG | |
Chemical compound, drug | Amicon Ultra centrifugal filter 100 K | Millipore Sigma | UFC510024 | |
Chemical compound, drug | Methanol | Fisher chemical | A452SK-4 | |
Chemical compound, drug | Tube-o-dialyzer 15 kDa | G-Biosciences | 786–618 | |
Chemical compound, drug | Amicon Ultra centrifugal filters 3 K | Millipore Sigma | UFC500324 | |
Chemical compound, drug | Coomassie Brilliant Blue G-250 | Calbiochem | 3340 | |
Peptide, recombinant protein | Xenopus laevis H2A, H2B, H3.2, H4 | Zierhut et al., 2014 | N/A | |
Chemical compound, drug | Ni-NTA beads | QIAGEN | 30210 | |
Chemical compound, drug | Carbenicillin | Alfa Aesar | J61949 | |
Chemical compound, drug | Methanol | Fisher chemical | A452SK-4 | |
Chemical compound, drug | Trehalose | Sigma | T0167-100G | |
Chemical compound, drug | 1,6,-hexanediol | Alfa Aesar | A12439 | |
Peptide, recombinant protein | MluI | New England Biolabs | R3198S | |
Peptide, recombinant protein | AscI | New England Biolabs | R0558S | |
Peptide, recombinant protein | XhoI | New England Biolabs | R0146S | |
Chemical compound, drug | EZ-link Maleimide-PEG2-Biotin | Thermo | A39261 | |
Chemical compound, drug | Isopropyl-β-D-thiogalactopyranoside (IPTG) | RPI research products | I56000-25.0 | |
Peptide, recombinant protein | Mono-SPYtag-avidin tetramer | This work | ||
Peptide, recombinant protein | Biotin-30 nm-SAH-SPYcatcher003 | This work | ||
Peptide, recombinant protein | Biotin-60 nm-SAH-SPYcatcher003 | This work | ||
Peptide, recombinant protein | Biotin-90 nm-SAH-SPYcatcher003 | This work | ||
Peptide, recombinant protein | Biotin-3HB-SPYcatcher003 | This work | ||
Peptide, recombinant protein | SPYtag-GFPenhancer-GGGGS4-LaG16 | This work | ||
Peptide, recombinant protein | SPYtag-GFP enhancer nanobody | This work | ||
Peptide, recombinant protein | SPYtag-LaG (llama antibody against GFP)–10 | This work | ||
Peptide, recombinant protein | H1.8-GFP | This work | ||
Peptide, recombinant protein | MNase | This work | ||
Peptide, recombinant protein | HaeII | New England Biolabs | R0107S | |
Peptide, recombinant protein | DraI | New England Biolabs | R0129S | |
Peptide, recombinant protein | EcoRI | New England Biolabs | R3101S | |
Peptide, recombinant protein | XbaI | New England Biolabs | R0145S | |
Peptide, recombinant protein | Klenow fragment | New England Biolabs | M0212S | |
Chemical compound, drug | biotin-14-dATP | Jena Bioscience GmbH | NU-835-BIO14-S | |
Chemical compound, drug | Intercept TBS Blocking Buffer | LI-COR Biosciences | 927–60001 | |
Chemical compound, drug | nitrocellulose membrane | Cytiva | 10600000 | |
Chemical compound, drug | Protein-A coupled Dynabeads | Thermo Fisher Scientific | 10001D | |
Chemical compound, drug | Hi-load Superdex200 16/600 column (Cytiva) | Cytiva | 28989335 | |
Chemical compound, drug | Hi-load Superdex75 16/600 column (Cytiva) | Cytiva | 28989333 | |
Chemical compound, drug | BS3 (bis(sulfosuccinimidyl)suberate) | Thermo Fisher Scientific | A39266 | |
Chemical compound, drug | 1 x PhosSTOP | Roche | 4906845001 | |
Chemical compound, drug | Absolute Mag streptavidin nano-magnetic beads | CD bioparticles | WHM-X047 | |
Chemical compound, drug | monolayer graphene grown on the copper foil | Grolltex | GRF23-L062−6x6 | |
Chemical compound, drug | polymethyl methacrylate | Micro chem | EL6 | |
Chemical compound, drug | Ammonium Persulfate | Thermo Scientific | AC401165000 | |
Chemical compound, drug | 2-butanone | Thermo Scientific | AA39119K7 | |
Chemical compound, drug | 2-propanol | Fisher Scientific | BP26184 | |
Chemical compound, drug | NucBlue Fixed Cell ReadyProbes Reagent | Thermo Fisher | R37606 | |
Other | in vitro reconstituted poly-nucleosome on magnetic beads | This work | EMD-42599 | |
Other | in vitro reconstituted H1-GFP bound nucleosome (MagIC-cryo-EM) | This work | EMD-42598 | |
Other | Xenopus egg extract H1-GFP bound nucleosome structure containing both interphase and metaphase particles (MagIC-cryo-EM) | This work | EMD-42594 | |
Other | interphase Xenopus egg extract H1-GFP bound nucleosome (MagIC-cryo-EM) | This work | EMD-42596 | |
Other | metaphase Xenopus egg extract H1-GFP bound nucleosome (MagIC-cryo-EM) | This work | EMD-42597 | |
Other | Averaged NPM2-H1.8-GFP structure (MagIC-cryo-EM) | This work | EMD-43238 | |
Other | open NPM2-H1.8-GFP structure (MagIC-cryo-EM) | This work | EMD-43239 | |
Other | closed NPM2-H1.8-GFP structure (MagIC-cryo-EM) | This work | EMD- 43240 | |
Other | averaged NPM2-H1.8-GFP structure | This work | PDB 8VHI | |
Other | open NPM2-H1.8-GFP structure | This work | PDB 8VHJ | |
Other | closed NPM2-H1.8-GFP structure | This work | PDB 8VHK | |
Recombinant DNA reagent | pET21-SPY-His6-tag streptavidin | This work | Addgene 214836 | |
Recombinant DNA reagent | pET21-streptavidin | This work | Addgene 214835 | |
Recombinant DNA reagent | pQE80-His14-bdSUMO-Cys-30nm-SAH-SPYcatcher003 | This work | Addgene 214839 | |
Recombinant DNA reagent | pQE80-His14-bdSUMO-Cys-60 nm-SAH-SPYcatcher003 | This work | Addgene 214840 | |
Recombinant DNA reagent | pQE80-His14-bdSUMO-Cys-3HB-SPYcatcher003 | This work | Addgene 214838 | |
Recombinant DNA reagent | pQE80-SPYtag-GFP enhancer nanobody | This work | Addgene 214837 | |
Recombinant DNA reagent | Xenopus laevis | NASCO | Wild type | |
Recombinant DNA reagent | pSF1389 | Frey and Görlich, 2014 | Addgene 104962 | |
Recombinant DNA reagent | pCDNA-FRT-FAK30 | Ritt et al., 2013 | Addgene 59121 | |
Recombinant DNA reagent | pET21a-Streptavidin-Alive | Howarth et al., 2006 | Addgene 20860 | |
Software, algorithm | MASCOT through Proteome Discoverer v.1.4 | Thermo Scientific | N/A | |
Software, algorithm | MOTIONCOR2 | Zheng et al., 2017 | https://emcore.ucsf.edu/ucsf-software | |
Software, algorithm | RELION v4 | Scheres, 2012; Scheres, 2022 | https://github.com/3dem/relion | |
Software, algorithm | CryoSPARC v3 and v4 | Punjani et al., 2017 | https://cryosparc.com | |
Software, algorithm | Chimera | Pettersen et al., 2004 | https://www.cgl.ucsf.edu/chimera/ | |
Software, algorithm | ChimaraX | Pettersen et al., 2004 | https://www.rbvi.ucsf.edu/chimerax/ | |
Software, algorithm | Topaz v0.2 | Bepler et al., 2019a; Bepler et al., 2019b | https://github.com/tbepler/topaz | |
Software, algorithm | Bsoft | Cardone et al., 2013 | https://lsbr.niams.nih.gov/bsoft/ | |
Software, algorithm | Coot | Emsley and Cowtan, 2004 | https://www2.mrc-lmb.cam.ac.uk/personal/pemsley/coot/ | |
Software, algorithm | PHENIX | Liebschner et al., 2019 | https://phenix-online.org/documentation/reference/refinement.html | |
Software, algorithm | APBS | Baker et al., 2001 | https://www.cgl.ucsf.edu/chimera/docs/ContributedSoftware/apbs/apbs.html | |
Software, algorithm | PDB2PQR | Dolinsky et al., 2007 | https://www.cgl.ucsf.edu/chimera/docs/ContributedSoftware/apbs/pdb2pqr.html | |
Software, algorithm | SWISS-MODEL | Waterhouse et al., 2018 | https://swissmodel.expasy.org | |
Software, algorithm | RING 2.0 webserver | Piovesan et al., 2016 | http://old.protein.bio.unipd.it/ring/ | |
Software, algorithm | Pyem v0.5 | Asarnow et al., 2019 | https://github.com/asarnow/pyem | |
Software, algorithm | ImageJ | Schneider et al., 2012 | RRID:SCR_003070, https://imagej.nih.gov/ij/ | |
Software, algorithm | Python | Python Software Foundation | https://www.python.org | |
Software, algorithm | Jupyter Notebook | Project Jupyter | https://jupyter.org | |
Software, algorithm | Microsoft Excel | Microsoft | https://www.microsoft.com/en-us/microsoft-365/excel | |
Software, algorithm | Soft-WoRx (Applied Precision) | SoftWoRx software | RRID:SCR_019157 | |
Software, algorithm | Fiji (ver. 2.9.0) | Schindelin et al., 2012 | RRID:SCR_002285, https://imagej.net/software/fiji/downloads | |
Software, algorithm | RStudio ver. RSTUDIO-2023.09.1–494 | RStudio Team, 2020 | https://posit.co/download/rstudio-desktop/ | |
Software, algorithm | R (ver. 4.2.2) | R Development Core Team, 2021 | https://www.R-project.org/ | |
Software, algorithm | ggplot2 | Wickham et al., 2016 | https://ggplot2.tidyverse.org/ | |
Software, algorithm | ggthemes (v5.1.0.9000) | Arnold, 2024 | https://jrnold.github.io/ggthemes/ | |
Software, algorithm | ggpubr | Kassambara, 2023 | https://rpkgs.datanovia.com/ggpubr/ | |
Software, algorithm | dplyr | Wickham et al., 2023 | https://dplyr.tidyverse.org/ | |
Software, algorithm | lawstat | Hui et al., 2008 | https://doi.org/10.18637/jss.v028.i03 | |
Software, algorithm | see | Lüdecke et al., 2021 | https://joss.theoj.org/papers/10.21105/joss.03393 | |
Software, algorithm | scales | Wickham et al., 2025 | https://scales.r-lib.org | |
Software, algorithm | GIMP software (ver. 4.2.2). | GIMP (GNU Image Manipulation Program) | https://gimp.org | |
Software, algorithm | Inkscape 1.2 | Inkscape Developers 2022 | https://inkscape.org/ | |
Software, algorithm | ColabFold v1.5.5 | Mirdita et al., 2022; Mirdita et al., 2025 | https://github.com/sokrypton/ColabFold | |
Software, algorithm | Starmap v1.2.15 | Lugmayr et al., 2023a; Lugmayr et al., 2023b | https://github.com/wlugmayr/chimerax-starmap | |
Other | Xenopus laevis MS database | Wühr et al., 2014 | https://scholar.princeton.edu/wuehr/protein-concentrations-xenopus-egg | |
Other | Centrifuge rotor | Beckman Coulter | SX241.5 | |
Other | Ultra centrifuge rotor | Beckman Coulter | SW55Ti | |
Other | Ultra centrifuge rotor | Beckman Coulter | SW40Ti | |
Other | Centrifuge rotor | Beckman Coulter | JS 5.3 | |
Other | Centrifuge | Beckman Coulter | Allegron X-30R | |
Other | Ultra centrifuge | Beckman Coulter | Optima L80 | |
Other | Centrifuge | Beckman Coulter | Avanti J-26S | |
Other | Imaging System | Li-Cor | Odyssey Infrared Imaging System | |
Other | LC-MS/MS system | Thermo Scientific | Dionex3000 HPLC, NCS3500RS nano- and microflow pump, Q-Exactive HF mass spectrometer | |
Other | Plunge freezer | FEI | Vitrobot Mark IV | |
Other | Plasma Cleaner | Gatan | Solarus II | |
Other | Cryo-EM | FEI | Talos Arctica TEM | |
Other | Cryo-EM | FEI | Titan Krios TEM | |
Other | Cryo-EM detector | GATAN | K2 Camera | |
Other | Cryo-EM detector | GATAN | K3 Camera | |
Other | N52 neodymium magnets | DIYMAG | D40x20–2P-NEW | |
Other | Cryo-EM grid | Quantifoil | Gold R 1.2/1.3 300 | |
Other | Spectrophotometers | Thermo Scientific | Nanodrop ND-2000C | |
Other | Centrifuge rotor | Beckman Coulter | JS 7.5 | |
Other | Fluorescence microscope | Applied Precision | DeltaVision Image Restoration microscope |
Additional files
-
Supplementary file 1
Statistics of the cryo-EM structures.
- https://cdn.elifesciences.org/articles/103486/elife-103486-supp1-v1.xlsx
-
Supplementary file 2
Mass spectrometry data.
- https://cdn.elifesciences.org/articles/103486/elife-103486-supp2-v1.xlsx
-
MDAR checklist
- https://cdn.elifesciences.org/articles/103486/elife-103486-mdarchecklist1-v1.docx