Science Forum: Viral factors in influenza pandemic risk assessment
Figures
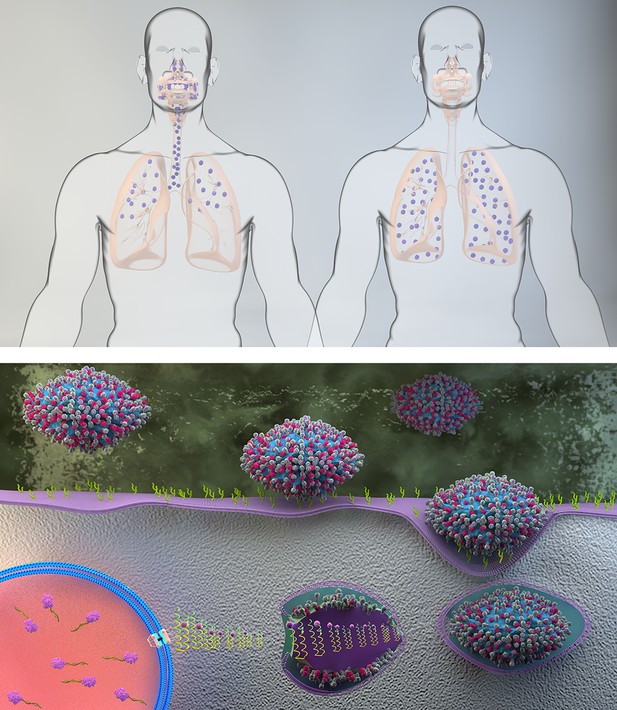
Key phenotypic traits for the adaptation of avian influenza viruses to replicate efficiently in humans.
(A) A switch in receptor binding preference from avian-like (α2,3-linked sialic acid) to human-like (α2,6-linked sialic acid) receptors. The human form on the left shows the typical distribution of human adapted influenza viruses determined by their receptor binding preference for a2,6, linked SA that is predominantly expressed in the upper respiratory tract but also in the lungs. The human form on the right shows that infection with avian influenza viruses is concentrated in the lungs where their preferred a2,3 linked SA receptor is expressed. (B) Lower HA pH of activation and increased polymerase complex efficiency. Free-floating viruses that enter the human respiratory tract (upper part of figure) encounter mucus and a mildly acidic extracellular environment that act as innate barriers to virus infection. If NA is able to desialylate decoy receptors on mucus and HA has a sufficiently low pH of activation, then the virus particle may reach the apical surface of the respiratory epithelium intact. There through a multiplicity of interactions between HA and cell-surface sialic acid, the virus enters the target cell. After the virus is internalized, it passes through the endosomal pathway where the pH is progressively decreased. The low pH of the endosomal environment triggers an irreversible conformational change in HA that fuses the viral and endosomal membranes and ultimately results in the release of virus genetic material in the form of the viral ribonucleoprotein complex (vRNP) into the cell cytoplasm. The eight vRNPs are subsequently imported into the cell nucleus by interactions between the vRNPs and cellular nuclear import machinery. Inside the nucleus the virus polymerase complex replicates the virus genome in conjunction with co-opted cell proteins.
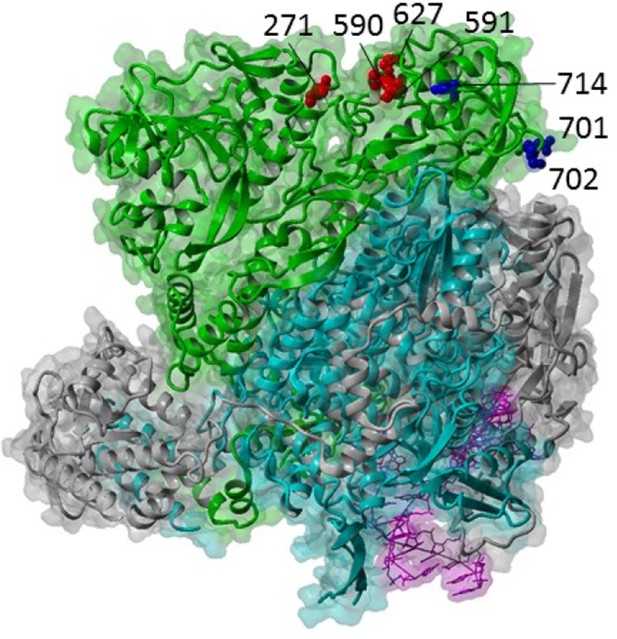
Influenza A polymerase complex from structure PDB:4WSB (Reich et al., 2014) consisting of PA (grey), PB1 (cyan), PB2 (green) and bound vRNA promoter (purple).
Key host adaptation sites are indicated as red balls. Sites for importin-alpha interaction are shown as blue balls. Structure visualized with YASARA (Krieger and Vriend, 2014).
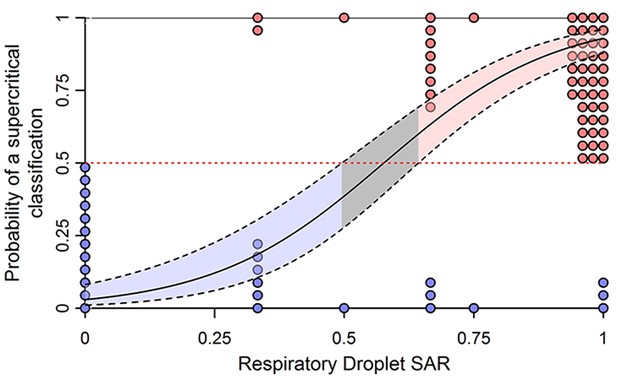
Ferret respiratory droplet transmission experiments predict the potential for sustained human-to-human transmission of influenza viruses.
The solid line shows the weighted logistic regression relationship predicting the probability that a given strain is supercritical (i.e. capable of sustained spread among humans), and the dashed lines show the 95% confidence interval for the prediction. Filled circles show the measured secondary attack rates (SAR) in ferrets for influenza subtypes that are known to be subcritical (blue) or supercritical (red) in humans. The filled pink area shows the range of SAR for which the virus is significantly likely to be supercritical. Reprinted from (Buhnerkempe et al., 2015).
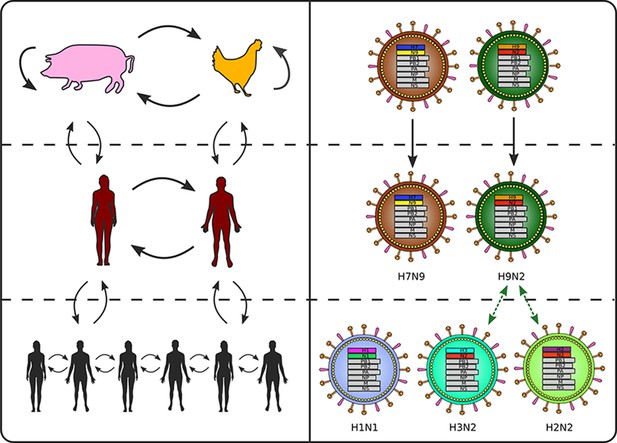
Transmission genomics of non-human transmission (top), spillover transmission (middle) and sustained human transmission (bottom).
Haemagglutinin and neuraminidase gene segments have been color-coded to show an example shared infection history in humans who are current spillover hosts for H7N9 and H9N2. These shared evolutionary histories make it challenging to interpret serological studies of human spillover infections. Humans infected by H2N2 or H3N2 will likely have cross-reactive antibodies to H9N2, because of the similarity between the neuraminidase in those viruses. Because incidence of spill-over infection is likely to be low, even low-levels of cross-reactivity can make the interpretation of serological studies of the general population challenging.
Tables
Influenza virus adaptations that appear to be required for human-to-human transmission.
Trait | Adaptation |
---|---|
HA receptor binding specificity | Preference for α2,6-linked mammalian sialic acid receptors over α2,3-linked avian ones (Russell et al., 2006) |
HA pH of activation | HA avoids extracellular inactivation and undergoes conformational changes leading to membrane fusion at appropriate pH for human cells (5.0–5.4 or perhaps 5.5) (Russell, 2014) |
Polymerase complex efficiency | Efficient replication in human cells (Cauldwell et al., 2014; Naffakh et al., 2008) |
Virus morphology | Filamentous morphology associated with several adaptations to mammals (Seladi-Schulman et al., 2014; Seladi-Schulman et al., 2013; Campbell et al., 2014; Beale et al., 2014) |
Length of NA stalk | Longer stalk of NA required to penetrate human mucus and deaggregate virions (Blumenkrantz et al., 2013) |
Antagonism of interferon production | Species-specific binding of the NS1 protein to host factors (Rajsbaum et al., 2012) |
HA-NA “balance” | Substrate selectivity and catalytic rate of NA are calibrated to “balance” avidity of HA for the cell-surface glycan receptor (Zanin et al., 2015; Baum and Paulson, 1991; Yen et al., 2011; Handel et al., 2014) |
Hemagglutinin receptor binding preference and examples of viruses isolated from avian and human hosts showing preference for human or avian receptors, or mixed preference. Yellow-shaded cells show concordance between expected and observed properties.
Avian receptor preference | Mixed receptor preference | Human receptor preference | |
---|---|---|---|
Expected sequence, trait. Hallmark residues HA 190, 225 (H1,H3), 226 (H3); many others | Preferential binding to α2,3 sialylated glycans. HA 190Glu, 225Gly, 226Gln | Similar binding to both classes of glycans | Preferential binding to α2,6 sialylated glycans. HA 190Asp, 225Glu, 226Leu |
Found in avian isolates | Many examples: many avian isolates of subtypes H5N1 (Russell et al., 2012; Yamada et al., 2006), H2 (Connor et al., 1994) and H3 (Connor et al., 1994) | avian isolates of H5N5 (Li et al., 2015), North American H7 (Belser et al., 2008), H7N9 (Schrauwen et al., 2016), as well as examples from H2 (Connor et al., 1994; Liu et al., 2009) and H3 (Connor et al., 1994) | Some H9N2 avian isolates (Matrosovich et al., 2001; Li et al., 2014) |
Found in human isolates | H5N1 zoonotic isolate (Imai et al., 2012; Yamada et al., 2006); one H1N1 isolate from 1957 (Rogers and D'Souza, 1989)*; some early H2N2 pandemic/seasonal isolates (Connor et al., 1994; Pappas et al., 2010; Matrosovich et al., 2000)* | Early H1N1 pandemic isolates from 2009(Childs et al., 2009) and 1918 (Stevens et al., 2006; Glaser et al., 2005); several H1N1 from the 1918-1956 period (Rogers and D'Souza, 1989)*; early H2N2 isolate from 1958 (Pappas et al., 2010); human isolate of zoonotic H7N9 (Xiong et al., 2013b) | Many examples: H1N1 post-1977 (Rogers and D'Souza, 1989); early H1N1 pandemic isolates from 2009 (Chen et al., 2011) and 1918 (Stevens et al., 2006; Glaser et al., 2005); most human H2 and H3 seasonal isolates (Connor et al., 1994; Matrosovich et al., 2000) |
-
*These anomalous results are speculated by the authors to be possibly, or even probably the result of laboratory adaptation to egg passage and may not reflect the properties of the primary isolate. A possible counter to this interpretation is that it is seen only in the earliest isolates from human pandemic viruses, while nearly all isolates from after the pandemic year, which should also have been passaged in eggs, show human-adapted phenotypes.
Hemagglutinin pH of acivation.Yellow-shaded cells show concordance between expected and observed properties.
Avian-adapted for transmissibility | Human-adapted for transmissibility | |
---|---|---|
Expected trait | pH of fusion >5.4 (Reed et al., 2010) | pH of fusion 5.0-5.4 (5.5 for early H1N1pdm) (Russier et al., 2016) |
Found in avian isolates | Avian H1-H4, H11 isolates (Galloway et al., 2013; Russier et al., 2016; DuBois et al., 2011; Reed et al., 2010) | Avian H5, H8, H9,H10,H14,H15 isolates (Galloway et al., 2013) |
Found in human isolates | H5N1(Imai et al., 2012; Linster et al., 2014) and H7N9 (Schrauwen et al., 2016) human zoonotic isolates with pH ≥5.6. One human H1N1 (2008) isolate. | Human isolates of H1N1 (1918 and 2009 lineages), H2N2, H3N2 (Galloway et al., 2013) |
Polymerase complex efficiency; entries list amino acid at PB2 627, though other residues are clearly relevant to this trait.Yellow-shaded cells show concordance between expected and observed properties.
Avian-adapted | Human-adapted | |
---|---|---|
Expected trait | Low efficiency in mammals, PB2 590/591 G/Q, 627E, 701D | High efficiency in mammals, PB2 590/591 S/R, PB2 627K, 701N; |
Found in avian isolates | Nearly all avian sequences in databases as of 2005 (Chen et al., 2006) | A few entries in databases show sequences associated with human adaptation as of 2005 (Chen et al., 2006)*** |
Found in human isolates | zoonotic H9N2 (Chen et al., 2006); some zoonotic H5N1 (de Jong et al., 2006; Chen et al., 2006); some zoonotic H7N9 (associated with milder course) (Sha et al., 2016); one zoonotic H5N6 (Zhang et al., 2016)** | Pandemic and seasonal H1N1, H2N2, H3N2 from 1918-2008 (Cauldwell et al., 2014); some zoonotic H5N1 (Chen et al., 2006); some zoonotic H7N9 (associated with more severe course) (Sha et al., 2016); H1N1pdm (Herfst et al., 2010)*; one zoonotic H5N6 and one zoonotic H10N8 (Zhang et al., 2016) |
-
* the role of amino acids 590 and 591 in adaptation was not recognized until after the 2009 strain had already emerged (Mehle and Doudna, 2009); it has the residues associated with avian adaptation at sites 627 and 701 that were known at that time (Herfst et al., 2010).
-
** complete sequence information not given in the paper
-
*** the rarity of these raises questions about possible sequencing errors.