Peroxisomal dysfunctions cause lysosomal storage and axonal Kv1 channel redistribution in peripheral neuropathy
Figures
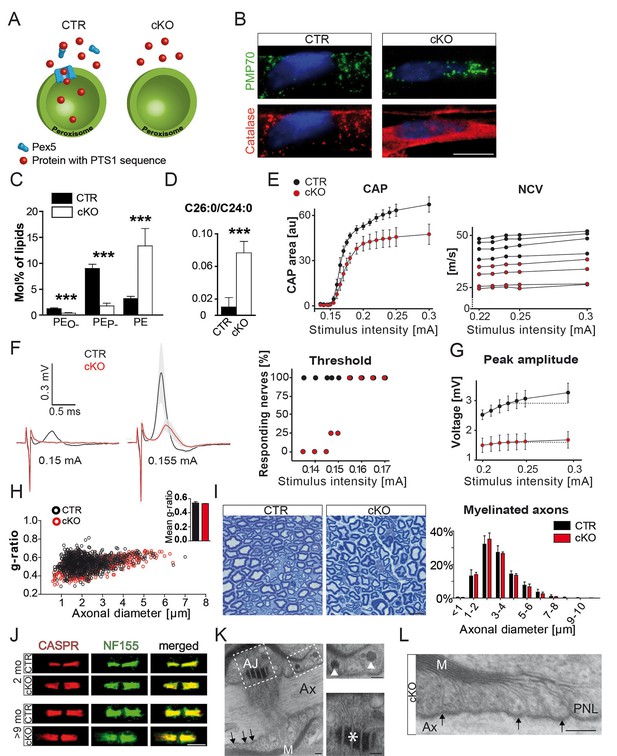
Schwann cell-specific PEX5-deficiency causes peroxisome dysfunction and peripheral neuropathy in the absence of axonal loss or dysmyelination.
(A) Scheme of normal (left) and impaired (right) PEX5-dependent peroxisomal protein import. PTS1, peroxisomal targeting signal type 1; PEX5, peroxisomal biogenesis factor peroxin 5. (B) Catalase (red) is present in peroxisomes (PMP70; green) of controls, but is localized in the cytoplasm of mutant fibers. PMP70, peroxisomal membrane protein 70; DAPI-stained SC nuclei are depicted in blue; Scale bar, 10 µm. (C, D) Lipid mass spectrometry of nerve lysates from controls and mutants aged 9 months indicates peroxisomal dysfunction. Peroxisomal products (PEO-) and its corresponding plasmalogens (PEP-) are reduced. Specific substrates of peroxisomal β-oxidation, VLCFA, are accumulated in mutant nerves. Statistics: means ± s.d.; n = 6; Student’s T-test; ***p<0.001. (E–G) Functional impairment of mutant compared to control sciatic nerves is assessed by electrophysiology at the age of 2 months (n = 4). To evoke significant responses of all measured nerves, larger stimulus intensity is required for mutant (0.155mA) as compared to control (0.135mA) nerves. Peak amplitudes plotted against increasing stimulus intensity indicates earlier response saturation of mutant nerves. CAP, compound action potentials; au, arbitrary units; NCV, nerve conduction velocities (each curve representing single-nerve mean responses across intensities). (H) g-ratio analysis by electron microscopy of sciatic nerves as measure of myelin thickness (n = 3; ≥100 randomly chosen axons per nerve at age 2 months). (I) Axonal analysis by methylene blue-stained semithin cross-sections of sciatic nerves of control and mutant mice at the age of 2 months (n = 3). Scale bar, 10 µm. (J) Immunostained teased sciatic nerve fibers of mutants and controls at 2 and 9 months show normal paranodal localization of CASPR (red) and anchoring protein neurofascin (NF155; green). (K, L) Intact transverse bands (arrows) at paranodal loops (PNLs) between axon (Ax) and myelin (M) by electron microscopy in a 9-month mutant nerve, even when PNLs harbor enlarged vesicles (top inset, arrowheads). Mutant nerves display normal adherens junctions (AJ) between loops (bottom inset, asterisk). Scale bars, 500 nm; scale bars in insets, 250 nm.
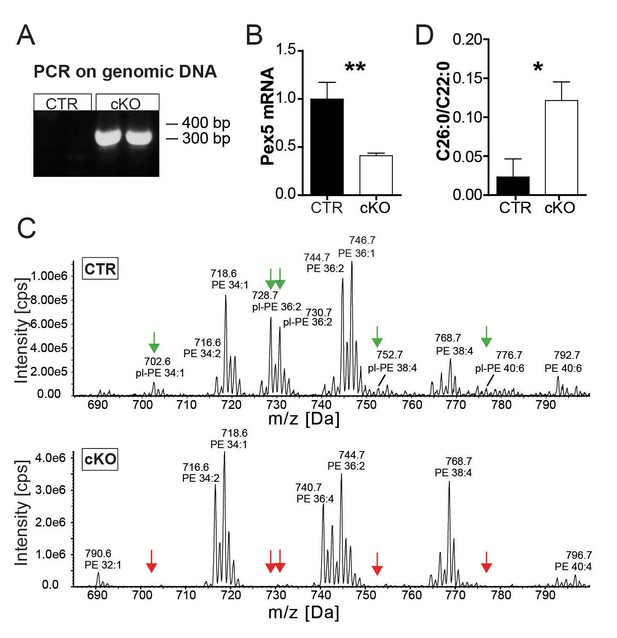
Successful PEX5 ablation causes peroxisomal dysfunction.
(A) PCR on genomic tail DNA using primers specifically generating a 300 bp amplicon after Cre-mediated excision of Pex5-floxed exons 11–14. (B) qRT-PCR reveals a 50% (corresponding to the fraction of SC nuclei) reduction of Pex5 mRNA level in mutant nerves (n = 6). (C) Quantitative mass spectrometry of sciatic nerve lysates. Plasmalogens were abundant in a control nerve (top, green arrows at peaks). The same plasmalogens were hardly detectable in a mutant nerve lysate (bottom, red arrows at peaks). (D) Analysis of VLCFA by gas chromatography–mass spectrometry in control and mutant mice. Statistics: means ± s.e.m.; n = 6; *p<0.05; **p<0.01; ***p<0.001, Student’s T-test (B, D).
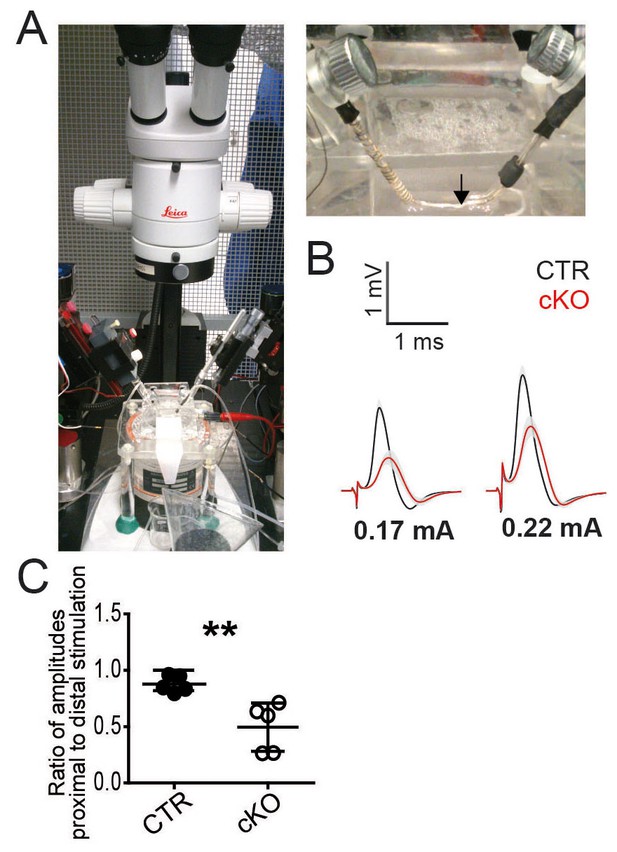
Electrophysiology of mouse sciatic nerves.
(A) Photographs of electrophysiology setup with a pair of suction electrodes. (B) Pex5 mutant nerves display smaller and delayed responses when elicited at stimulus intensities of 0.17mA (left) or 0.22mA (right). (C) In vivo electrophysiology of controls and PEX5 cKO shows a greater decline of the compound muscle action potential (CMAP) amplitudes measured at more proximal versus distal stimulation indicating conduction blocks. The ratios of the amplitudes elicited by proximal vs. distal stimulation are depicted. Statistics: n = 5, means ± s.d. **p<0.01, Student’s T-test.
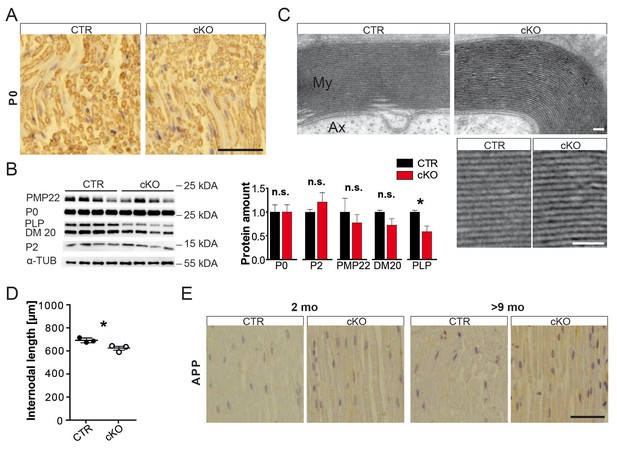
Myelin analysis of mouse sciatic nerves.
(A) Chromogenic immune-staining for myelin protein zero (MPZ/P0) on paraffin-embedded sciatic nerve sections at 2 months. Scale bar, 50 µm. (B) Abundance of major PNS myelin proteins P0 and PMP22, as well as fatty-acid-binding protein P2, and DM20 were not significantly altered by quantification of Western blot analysis of sciatic nerve lysates obtained at the age of 2 months (n = 4; each normalized to control levels; 1.0). Only the covalently lipid-binding protein PLP showed significant reduction in mutant as compared to control nerves. Alpha-Tubulin served as loading control. Statistics: mean ± s.e.m. *p<0.05; **p<0.01; ***p<0.001; n.s., not significant, Student’s T-test. (C) Electron micrographs of sciatic nerve cross-section showing myelin sheaths of animals aged 2 months. Scale bars, 10 nm. (D) Internodal lengths measured using teased fibers of 2-month-old PEX5 mutant and control animals. Statistics: n = 3, mean ± s.d. *p<0.05, Student’s T-test. (E) Chromogenic immune-staining for APP on longitudinal paraffin-embedded sciatic nerve sections. Scale bar, 50 µm.
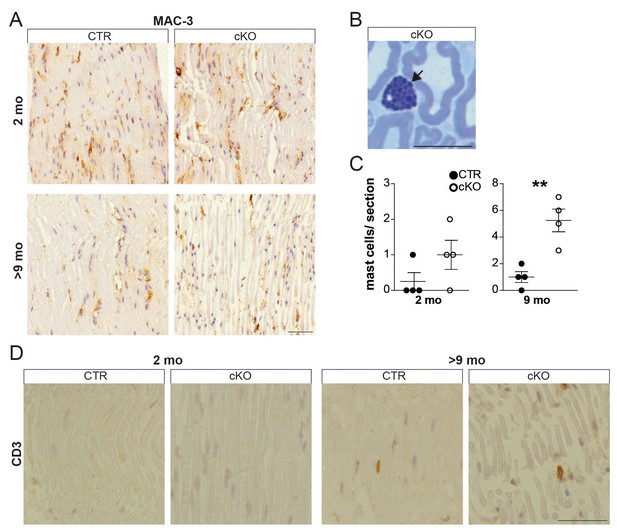
Young Pex5 mutant nerves lack signs of inflammation.
(A, D) Chromogenic immunostaining for MAC-3 (A) and CD3 (D) on longitudinal paraffin-embedded sciatic nerve sections of mice aged 2 and 9 months. (B, C) Mast cells (arrow) visualized and quantified using entire semithin (500 nm) methylene-blue-stained cross-sections of mouse sciatic nerves at 2 months of age (n = 4). Statistics: means ± s.e.m. *p<0.05; **p<0.01; ***p<0.001; Student’s T-test.
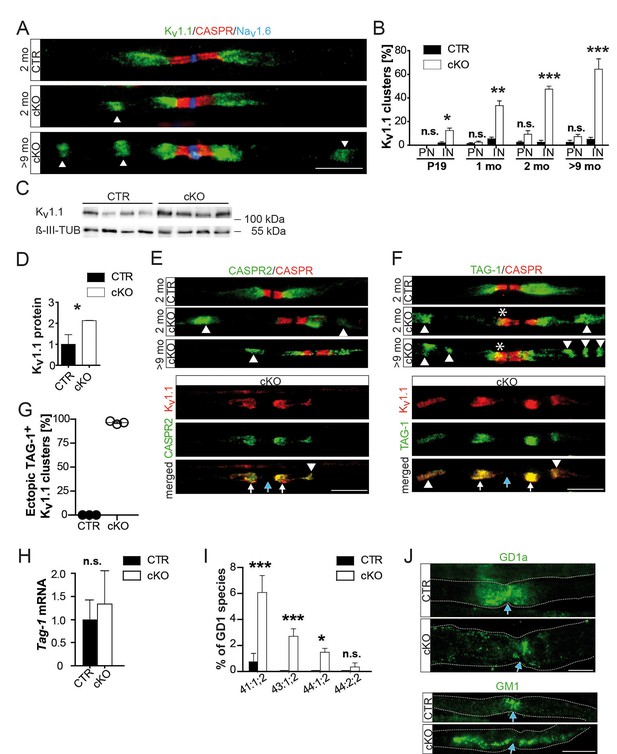
Abnormal distribution of axonal ion channels Kv1.1, its anchoring proteins, and associated lipids in conditional Pex5 mutant nerves.
(A, B) Immune-stained sciatic teased fibers of 2- and 9-month-old mice reveal normally localized nodal sodium channels (Nav1.6; blue) flanked by paranodal CASPR (red; scale bar, 10 µm). Only mutant nerves show extra Kv1.1+ patches within internodes. This is progressive with age shown by the corresponding quantification at different ages, starting at P19, revealing a shift of Kv1.1 primarily to internodes (IN), and (not significantly) to paranodes (PN). n = 3 for P19 and 1 month; n = 5 for 2 months; n = 7 for>9 months. Statistics: means ± s.e.m, two-way ANOVA followed by Student’s T-test. *p<0.05; **p<0.01; ***p<0.001; n.s., not significant. (C, D) Western blot analysis of sciatic nerve lysates of 9-month-old mice shows increased Kv1.1 protein abundance in mutant nerve lysates. β-III Tubulin served as loading control for normalization (n = 4). (E–G) Kv1-anchoring proteins (green) CASPR2 and TAG-1 showed in addition to normal juxtaparanodal localization an ectopic internodal (arrow heads) and occasionally a partial overlap (asterisk) with paranodal CASPR (red) in mutant sciatic teased fibers. Both proteins colocalized at original (white arrows) and ectopic (arrow heads) positions (bottom panels). Quantification of TAG-1/Kv1.1-positive ectopic clusters is depicted, (G). Blue arrows indicate nodes of Ranvier. Scale bars, 10 µm. (H) qRT-PCR analysis (n = 5) for TAG-1 showing a tendency of increased mRNA expression in mutant compared to control nerves at 2 months. (I) Mass spectrometry of sciatic nerve lysates (n = 3) obtained at the age of 9 months shows percentage of GD1 species per genotype containing 41, 43, or 44 C-atoms (‘:’ separates number of double-bonds and ‘;’ separates number of hydroxyl-groups). Only 0.74% of GD1 in control lysates contain 41 carbons, species with more carbons are absent, while highly enriched in mutant nerve lysates. (J) Antibody-staining for gangliosides of nerves from mice aged 9 months shows GD1a+ vesicles abnormally distended into mutant internodes (green, top). GM1 is localized at paranodes of control fibers, but extends into mu internodes. Dotted lines indicate borders of myelinated fibers. Blue arrows indicate nodes of Ranvier. Scale bars, 10 µm. Statistics: means ± s.d.; *p<0.05; **p<0.01; ***p<0.001; n.s., not significant, Student’s T-test.
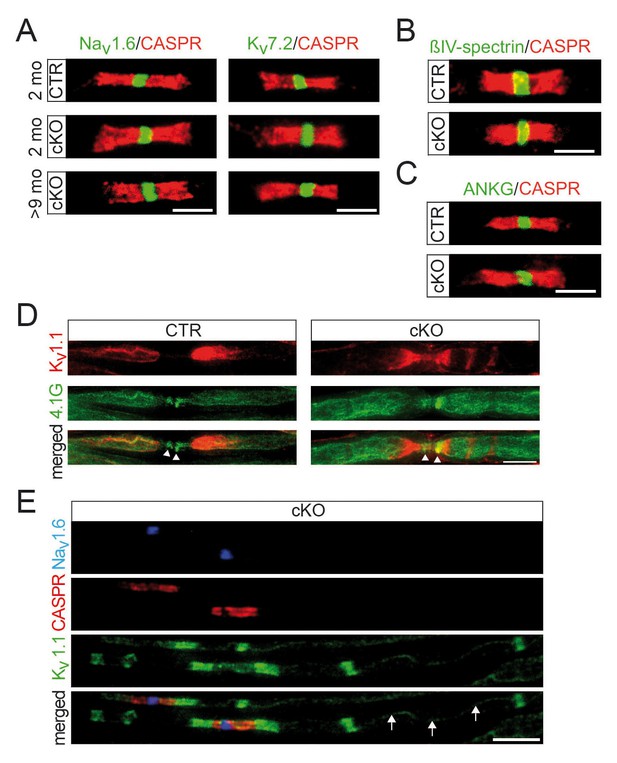
Normal distribution of nodal proteins and associated anchors in Pex5 mutant nerves.
Immune-stained sciatic teased fibers of 2- and 9-month-old mice. (A) Nodal sodium (Nav1.6; left) and potassium (Kv7.2; right) channels are normally localized and flanked by paranodal CASPR (red, scale bars, 5 µm). (B, C) Ion channel anchoring proteins βIV-spectrin (green) (B) and ankyrinG (green) (C) are normally localized to nodes of Ranvier and flanked by paranodal CASPR (red). Scale bars, 5 µm. (D) Staining for 4.1G (green), which unlike 4.1B is abundantly present at paranodes, shows normal localization (arrowheads). Potassium channel Kv1.1 clusters (red) are located at juxtaparanodes but in mutants additionally localize along internodes. (E) Triple-staining of Nav1.6 (blue), paranodal CASPR (red), and juxtaparanodal potassium channels Kv1.1 (green) shows preservation of Kv1.1 at juxtaparanodes and at mesaxonal lines (arrows) of mutant fibers. In addition, ectopic internodal Kv1.1 patches are depicted. Scale bar, 10 µm.
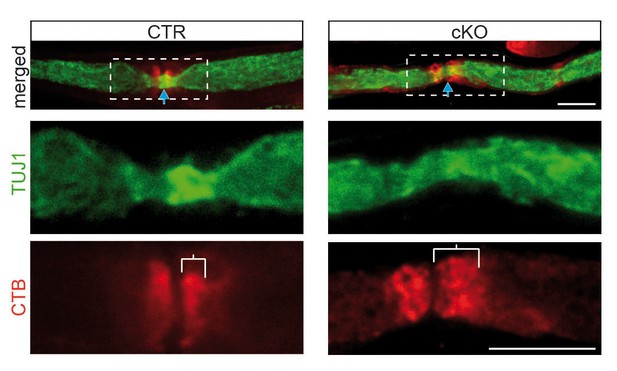
Abnormal distribution of GM1 ganglioside in the perinodal region of mutant nerves by CTB-staining.
GM1-labeling by CTB (red) of sciatic teased fibers from mice aged 9 months shows a defined paranodal staining in control (left) but is more distended in mutant fibers (right). Area in rectangles (top) is magnified in bottom panels. Axons are stained for TUJ1 (green). CTB, fluorescently tagged subunit B of cholera toxin.
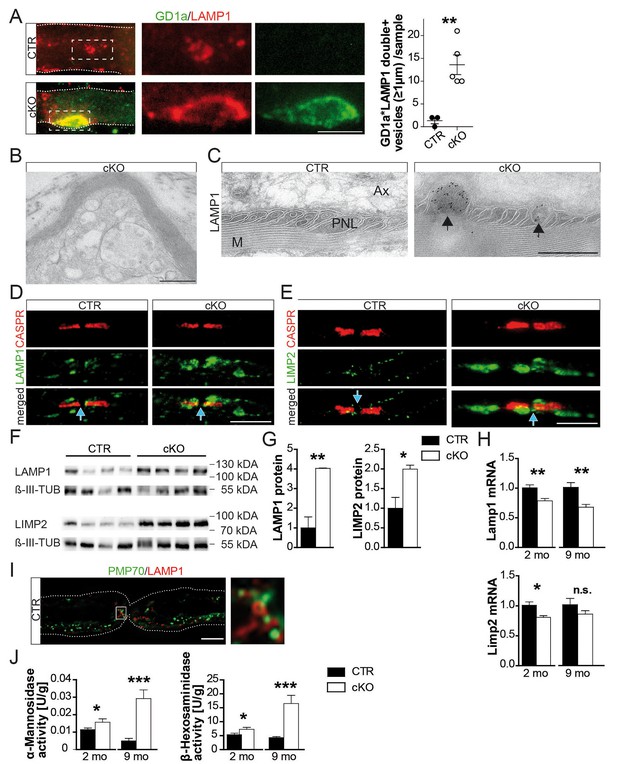
Hallmarks of lysosomal storage disorders in Pex5 mutant nerves.
(A) Double-staining of sciatic teased fibers displayed giant GD1a+ (green) vesicles that colocalized with LAMP1 (red) in internodes only of mutant animals (rectangular inset magnified in right panels). Dotted lines indicate borders of myelinated fibers; scale bar in inset, 5 µm. (B) Electron microscopy of a teased and sectioned mutant sciatic nerve depicting paranodal vesicle accumulations. Scale bar, 500 nm. (C) Immuno-electron microscopy of sciatic nerves from mice aged 9 months identifies enlarged LAMP1+ vesicles (arrows) within paranodal loops (PNLs). M, myelin; Ax, axon. Scale bar, 500 nm. (D, E) Immunolabeling of teased sciatic nerves at the age of 2 months shows enlarged LAMP1+ (left, green) or LIMP-2+ (right, green) compartments close to CASPR+ paranodes (red) of mutant nerves. Scale bars, 10 µm. (F–H) Analysis of sciatic nerve lysates by Western blotting (n = 4; β-III Tubulin, served as loading control for normalization) and qRT-PCR (n = 6) for LAMP1 and LIMP-2 showing increased protein, but decreased mRNA levels in mutants. (I) Immunostaining of control sciatic fibers shows close association of peroxisomal PMP70 (green) and LAMP1 on lysosomal membranes (red) in the paranodal region, suggesting physical interactions. A wide-field flourescent image after deconvolution (250 nm z-stack width) is shown. Scale bar, 10 µm. Dotted lines indicate borders of the myelinated fiber. (J) Lysosomal enzyme activities of nerve lysates was enhanced in mutants compared to controls as assessed by assays using substrates of α-Mannosidase (left) and β-Hexosaminidase (right; n = 4). Statistics: means ± s.e.m. *p<0.05; **p<0.01; ***p<0.001; n.s., not significant, Student’s T-test (G, H, J). Blue arrows indicate nodes of Ranvier (D, E, I).
-
Figure 3—source data 1
Lysosomal accumulation within paranodal loops.
- https://doi.org/10.7554/eLife.23332.012
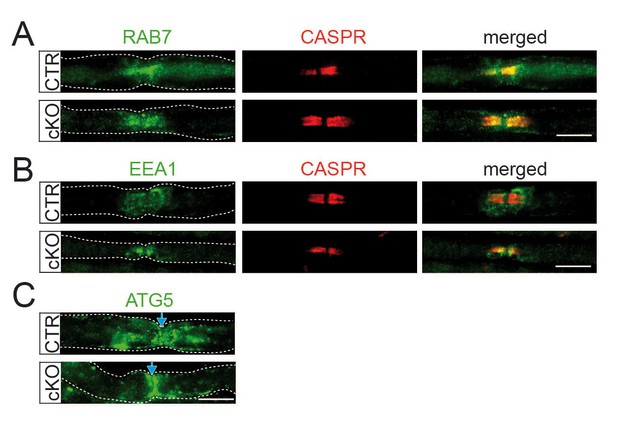
Characterization of paranodal vesicle inclusions in Pex5 mutant nerves.
(A–C) Immunofluorescence showing normal abundance of early/late endosomal and autophagosomal vesicles (green) in the vicinity of nodes of Ranvier (indicated by paranodal CASPR or blue arrow) of teased fibers obtained from 9-month-old animals. RAB7, Ras-related protein 7 (late endosomes; top); EEA1, early endosome-associated protein 1 (middle); ATG5, autophagy-related protein 5 (autophagosomes; bottom). Scale bars, 10 µm.
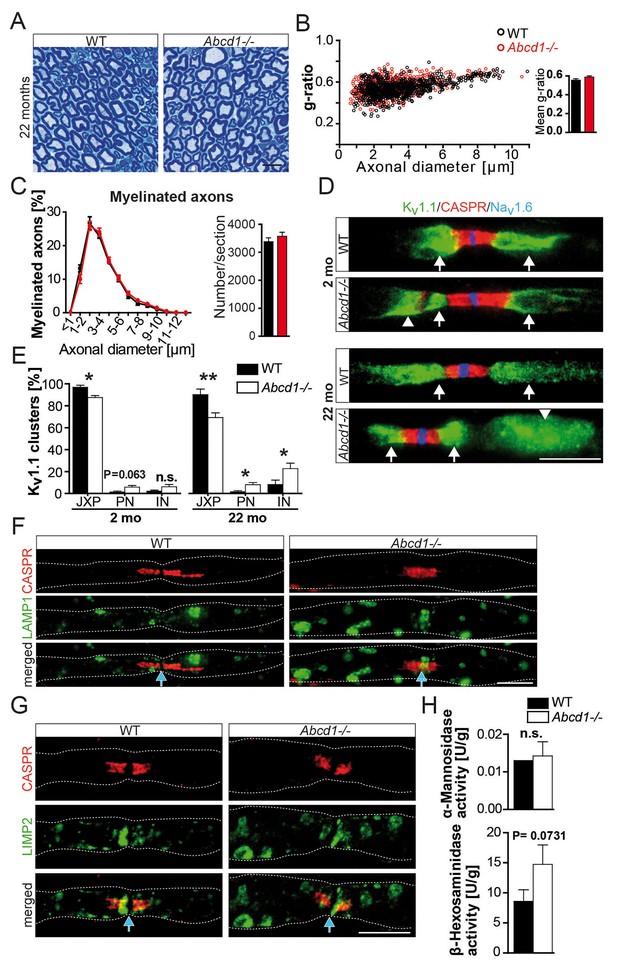
Normal nerve morphology, but ectopic ion channels and lysosomal accumulates in 22 months aged Abcd1 null mutants.
(A–C) Analysis of nerve morphology using entire methylene blue-stained semithin cross-sections (A, C) and g-ratios as measure of myelin thickness by electron microscopy of sciatic nerves (≥200 randomly chosen axons per nerve) obtained from control and Abcd1 knockout mice (n = 4). Scale bar, 10 µm. (D, E) Immune-stained teased sciatic nerve fibers obtained from mutants and controls with corresponding quantifications show progressively abnormal Kv1.1 localization (green), while Nav1.6 (blue) and CASPR (red) are normally localized. Scale bars, 10 µm. (n = 4, 2 mo; n = 6, 22 mo). Statistics: means ± s.e.m, Student’s T-test *p<0.05; **p<0.01; ***p<0.001; n.s., not significant. (F–H) Analysis of lysosomes reveals LAMP1+ and LIMP-2+ accumulations (green) by immune-stained sciatic teased fibers (n = 3) and a mild increase of lysosomal β-Hexosaminidase activity measured using sciatic nerve lysates (n = 4). Blue arrows indicate nodes of Ranvier. Scale bars, 10 µm. Statistics: means ± s.e.m, two-way ANOVA followed by Bonferroni (B) or Student’s T-test (H). *p<0.05; **p<0.01; ***p<0.001; n.s., not significant.
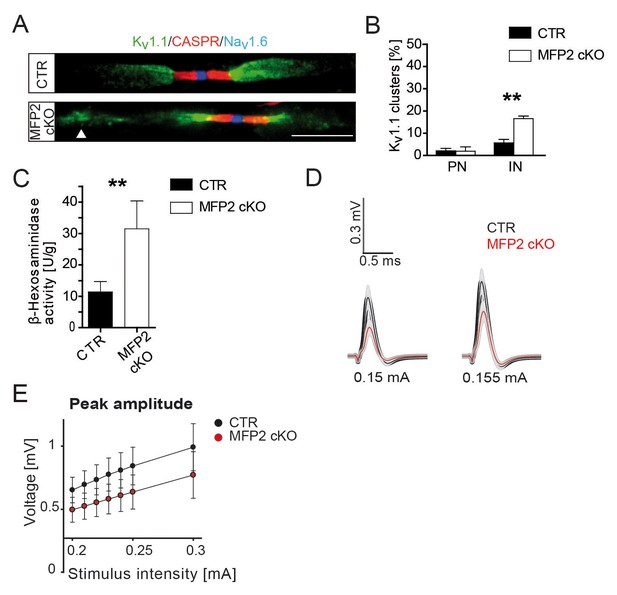
Ectopic, internodal Kv1-channel clusters in nerves of conditional MFP2 knockout mice.
(A) Fluorescent image of aged (19 months) Cnp-Cre::Mfp2flox/flox (MFP2 cKO) sciatic teased nerve fibers show normal distribution of nodal Nav1.6 (blue), paranodal CASPR (red), and juxtaparanodal Kv1.1 (green), as in controls. Mutant fibers exhibit in addition ectopic internodal Kv1.1 clusters (arrow head). Scale bar, 10 µm. (B) Quantification of ectopic Kv1.1 channel distribution at paranodes (PN) or internodes (IN). Statistics: means ± s.e.m. n = 3; *p<0.05; **p<0.01; ***p<0.001, Student’s T-test. (C) Enzyme assay measuring lysosomal β-Hexosaminidase in nerve lysates from animals aged 16 months showing increased activity in mutant nerve lysates. (D, E) Functional impairment of MFP2 mutant compared to control sciatic nerves assessed by ex vivo electrophysiology at age 16 months (n = 6). Mfp2 mutant nerves display smaller responses (red curves) as compared to controls (black curves) when elicited at stimulus intensities of 0.15mA (left) or 0.155mA (right). Peak amplitudes plotted against increasing stimulus intensity indicate reduced compound action potentials of MFP2 mutant nerves (red dots) compared to controls (black dots) at all intensities measured. Statistics: Two-way ANOVA, effect of genotype, F(1200)=27.37; p=0.
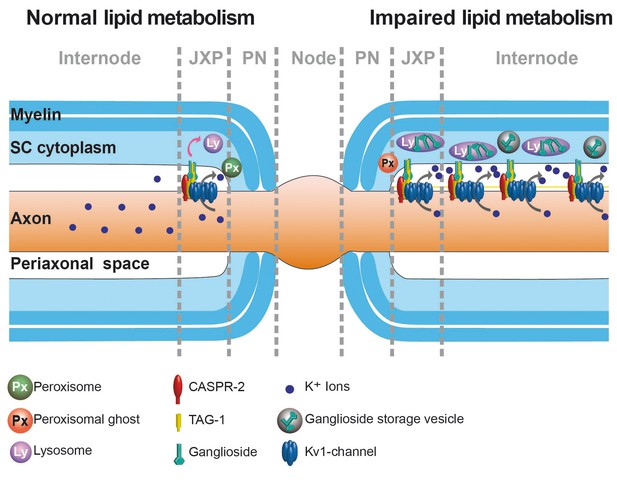
Hypothetical model for lipid turnover of the juxtaparanodal anchoring complex of Kv1-channels.
Myelin TAG-1 is stabilized by gangliosides (GS, light green) within juxtaparanodes (JXP). For normal turnover (left) of JXP domains, SC require lysosomes (purple) and peroxisomes (dark green) that degrade GS (indicated by curved pink arrow). When GS degradation is perturbed (right), indicated by impaired peroxisomes (red), GS accumulate within enlarged lysosomes (purple), storage vesicles (gray), and likely within JXP. Excess GS within JXP stabilize the protein complex containing TAG-1 (yellow), CASPR2 (red) and Kv1.1 (blue) proteins, which thereby escape normal turnover, leading to domains breaking-off and drifting into internodes. Extra clusters possibly allow more K+ (blue dots) efflux (curved gray arrow).