Amount of fear extinction changes its underlying mechanisms
Figures
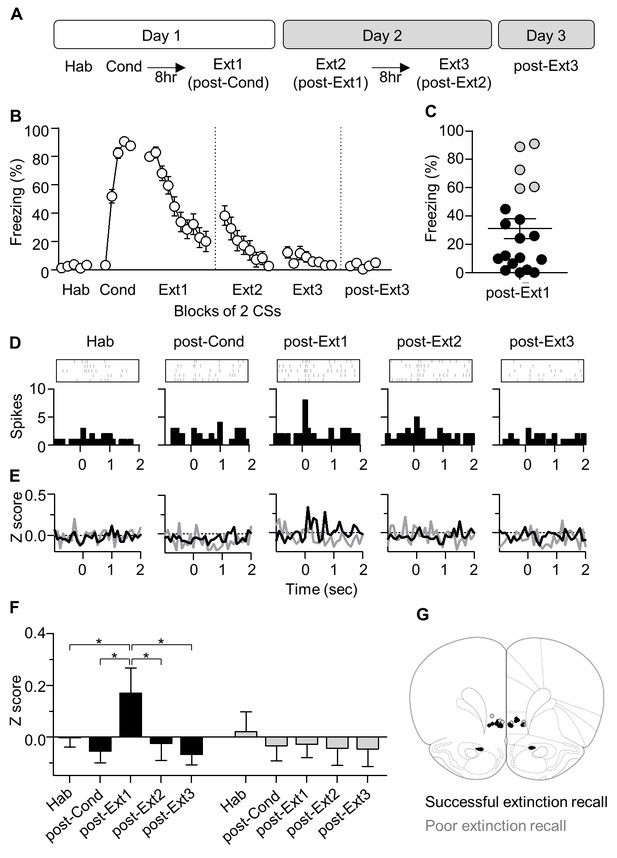
CS-evoked activities of IL neurons appear after single-session extinction-training, but disappear after multiple-session extinction-training.
(A) Experimental design. Hab, habituation; Cond, conditioning; Ext, extinction. (B) CS-induced freezing during conditioning and repeated extinction sessions (n = 19 rats). (C) Rats were divided into two groups according to the percent of time spent freezing in post-Ext1. Successful extinction recall, n = 14 rats, black circle; poor extinction recall, n = 5 rats, gray circle. (D) CS-evoked activities of an example IL neuron. X = 0 indicates the time of tone onset. (E–F) Averaged CS-evoked activities of IL neurons in the successful and poor extinction recall groups. ANOVA revealed a significant change only in the successful extinction recall group (F), for successful extinction recall group, n = 56 cells from 14 rats, F4,220 = 3.346, p=0.0319, *p<0.05 for post-Ext1 vs. the other groups, repeated measures one-way ANOVA followed by Newman-Keuls post-test; for poor extinction recall group, n = 16 cells from 5 rats, F4,60 = 0.2742, p=0.7918]. (G) Histological verification of the electrode placements.25224.
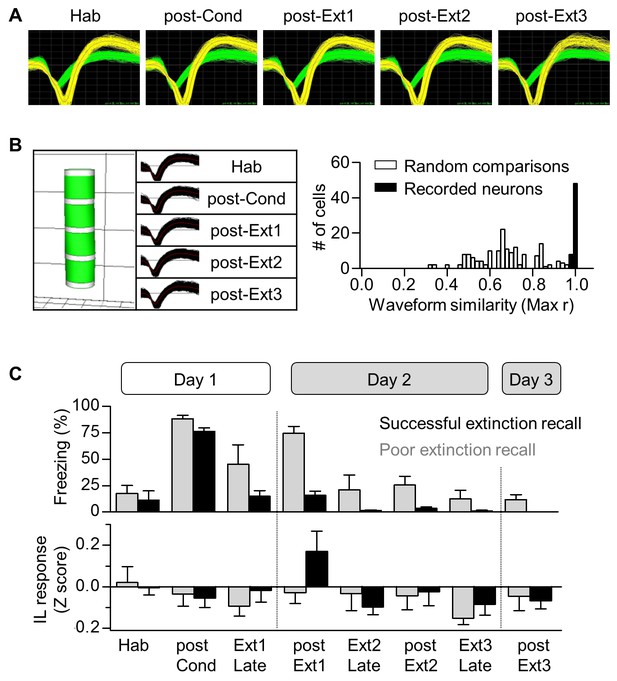
Long-term single unit recordings in the IL.
(A) Representative waveforms of two neurons recorded from a single electrode and observed throughout the behavioral training period. Grid, 55 μV x 100 μs. (B) Verification of long-term stable single unit recordings using principal component space cylinders (Left) and the linear correlation analysis between the template waveforms obtained over the entire set of behavioral sessions (Right). A straight cylinder and higher correlation value suggest the same set of single units was recorded during consecutive behavioral sessions. (C) CS-evoked freezing and IL neural responses for the successful (black) and poor (gray) extinction recall groups. The behavioral and neural responses were averaged for the first 5 CSs or the last 5 CSs.
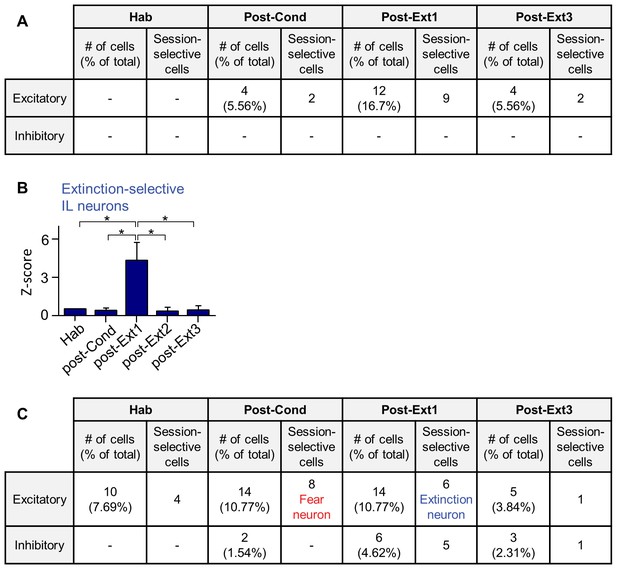
Cell-based analysis of single unit recordings in the IL and Ba.
(A) CS-responses in IL individual neurons throughout the training period. The cell-based analysis was conducted by comparing the largest z-values of 0–400 msec following CS onset (bin size, 100 msec). The number of CS-responsive cells and the percentage of total recorded neurons are indicated. Neurons displaying CS-responses only in one particular session were indicated as session-selective cells. (B) Extinction-selective cells in the IL (post-Ext1, n = 9 cells) shown in A (χ2 = 18.19, p=0.0011, *p<0.05 for post-Ext1 vs. the other groups, Friedman test followed by Dunn’s test). These cells were included in the successful extinction recall group in Figure 1 (n = 56 cells from 14 rats). The averaged CS-response latency of IL extinction neurons was 211.1 msec, whereas Ba extinction neurons responded to the CS with much shorter latencies, averaged at 43.33 msec (U = 0.0000, p=0.0016, Mann-Whitney test). (C) CS-responses of Ba neurons throughout the training. The cell-based analysis was conducted by comparing the significance of the CS-responses within 100 msec following CS onset throughout the training period (bin size, 20 msec). Fear neurons and extinction neurons have been indicated in red and blue, respectively.
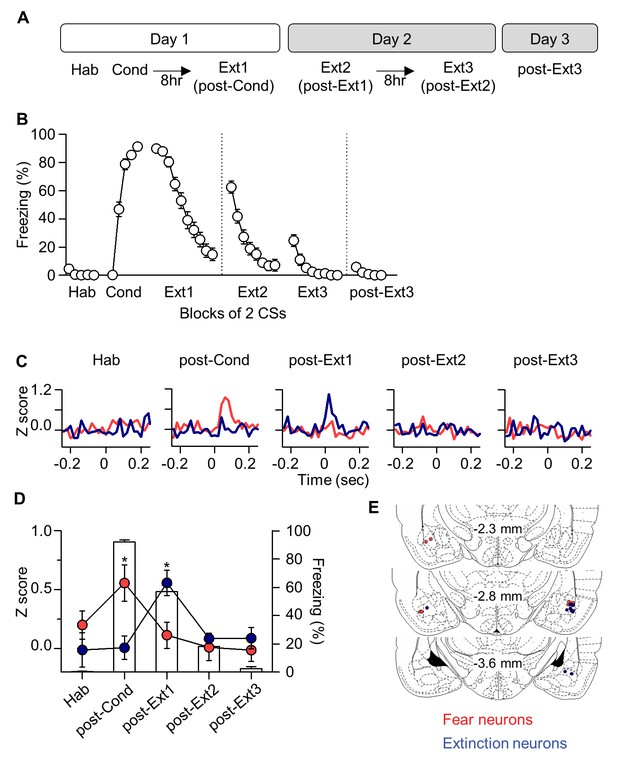
‘Extinction neurons’ in the Ba, which appear after single-session extinction-training, disappear after multiple-session extinction-training.
(A) Experimental design. (B) CS-induced freezing throughout the entire behavioral training (n = 27 rats). (C) Two distinct neuronal subpopulations were identified. Fear neurons, n = 8 cells from 6 rats, red; extinction neurons, n = 6 cells from 6 rats, blue. (D) Averaged CS-evoked activities of fear and extinction neurons were plotted against freezing behavior. Friedman test revealed a significant change in neuronal and behavioral responses (Fear neurons, χ2 = 14, p=0.0073, *p<0.05 for post-Cond versus the other groups, Friedman test followed by Dunn’s test; Extinction neurons, χ2 = 10.67, p=0.0306, *p<0.05 for post-Ext1 vs. the other groups, Friedman test followed by Dunn’s test; Freezing behavior, χ2 = 39.26, p<0.0001, p<0.01 for all designated pairs except Hab vs. post-Ext3, Friedman test followed by Dunn’s test). (E) Histological verification of the electrode placements.
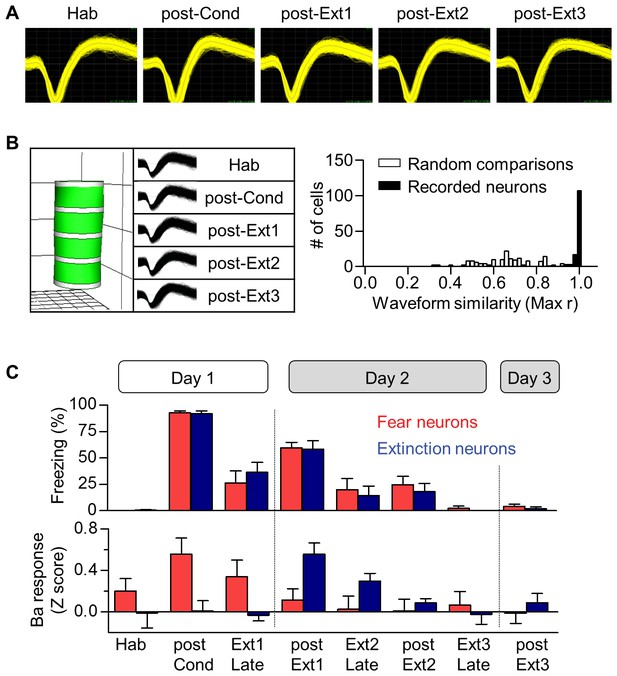
Long-term single unit recordings in the Ba.
(A) Representative waveforms of a neuron recorded from a single electrode and observed throughout the behavioral training period. Grid, 55 μV x 100 μs. (B) Verification of long-term stable single unit recordings as previously described (Herry et al., 2008). (C) CS-evoked freezing of rats that fear (red, n = 6 rats) and/or extinction (blue, n = 6 rats) neurons were recorded from (upper) and averaged neural responses of fear (red, n = 8 cells) and extinction (blue, n = 6 cells) neurons in the Ba (bottom) throughout the training. The behavioral and the neural responses were averaged either for the first 5 CSs or the last 5 CSs.
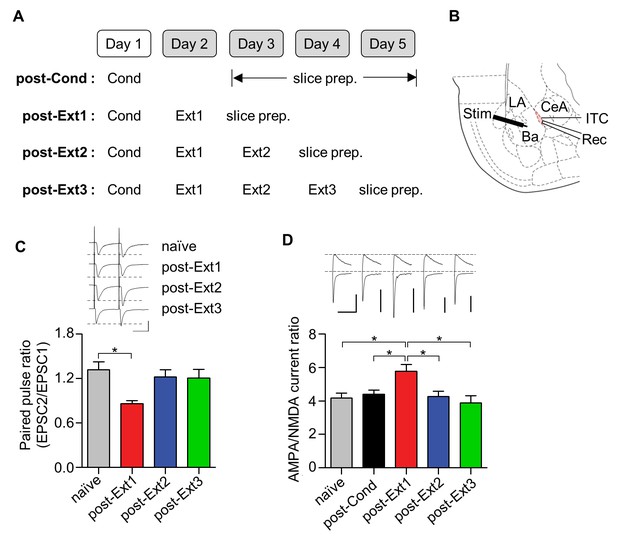
Ba-ITC synaptic efficacy increases after single-session extinction-training and returns to baseline after multiple-session extinction-training.
(A) Experimental design. (B) Placement of recording and stimulating electrodes. LA, lateral amygdala; Ba, basal amygdala; CeA, central amygdala; Rec, recording; Stim, stimulation. (C) Paired pulse ratio of Ba-ITC synapses. A significant decrease in the post-Ext1 group was observed (χ2 = 8.419, p=0.0381, *p<0.05 for post-Ext1 vs. naïve, Kruskal-Wallis test followed by Dunn’s test). Scale bars, 100 pA x 30 ms. (D) AMPA/NMDA current ratio of Ba-ITC synapses. Kruskal-Wallis test revealed a significant increase in the post-Ext1 group (χ2 = 14.25, p=0.0065, *p<0.05 for post-Ext1 vs. other groups, Kruskal-Wallis test followed by Dunn’s test). Scale bars, 100 pA and 100 ms.
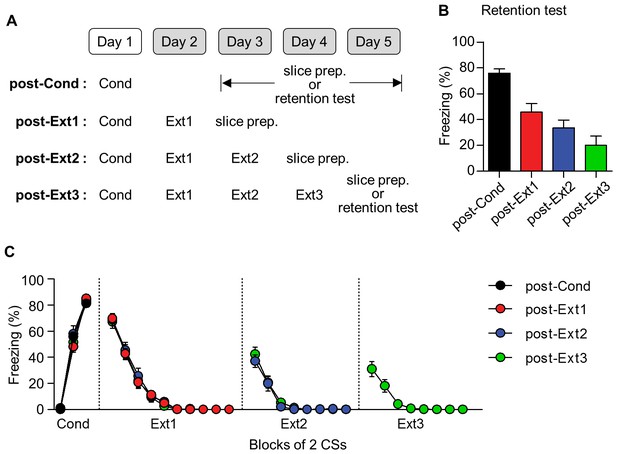
The behavioral results for the electrophysiological experiments shown in Figures 3–5.
An additional set of rats was used to monitor conditioned freezing for the retention test. (A) Experimental design. One set of rats was killed to prepare brain slices for the electrophysiological experiment (the number of rats; post-Cond = 61, post-Ext1 = 52, post-Ext2 = 25, post-Ext3 = 21), and another set of rats was used for the retention test (the number of rats; post-Cond = 16, post-Ext3 = 6). (B) Summary of conditioned freezing during the retention test for all behavioral groups. The retention test in the post-Ext1 and post-Ext2 groups represented the average of the first two data points in Ext2 and Ext3, respectively. (C) The freezing responses from consecutive behavioral sessions for all behavioral groups.
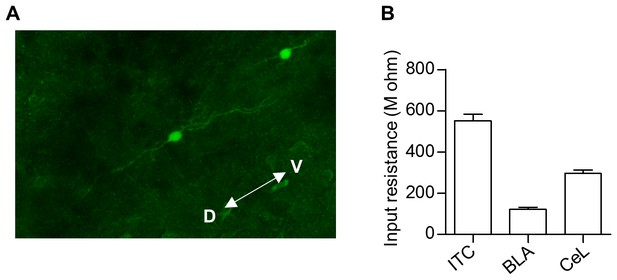
Identification of ITC neurons.
(A) Representative image of ITC neurons dialyzed with Lucifer Yellow fluorescence dye. Twenty neurons out of the twenty-one neurons tested (95.2%) were determined to be ITC neurons from their morphology and electrophysiological characteristics. (B) The input resistance of ITC neurons (552.5 ± 30.0 MΩ, n = 20 cells), BLA neurons (121.4 ± 10.0 MΩ, n = 9) and CeL neurons (295.6 ± 16.3 MΩ, n = 10). The ITC neurons had significantly higher input resistance than those of the other groups (χ2 = 31.79, p<0.0001, p<0.05 for ITC versus the other groups, Kruskal-Wallis test followed by Dunn’s test). The input resistance was measured with potassium-based internal solution, which contains (in mM) 120 potassium gluconate, 0.2 EGTA, 10 HEPES, 5 NaCl, 1 MgCl2, 2 Mg-ATP and 0.3 Na-GTP with the pH adjusted to 7.2 with KOH and the osmolarity adjusted to approximately 297 mmol/kg with sucrose. BLA, basolateral amygdala, CeL, lateral part of central amygdala. We targeted a visually identifiable cluster of small neurons (diameter ≤ 10 µm) located between the BLA and the CeL. The ITC neurons were defined as cells showing a typical morphology with bipolar elongation of neurites alongside D-V axis and high input resistance (>450 MΩ) as previously described (Amano et al., 2010).
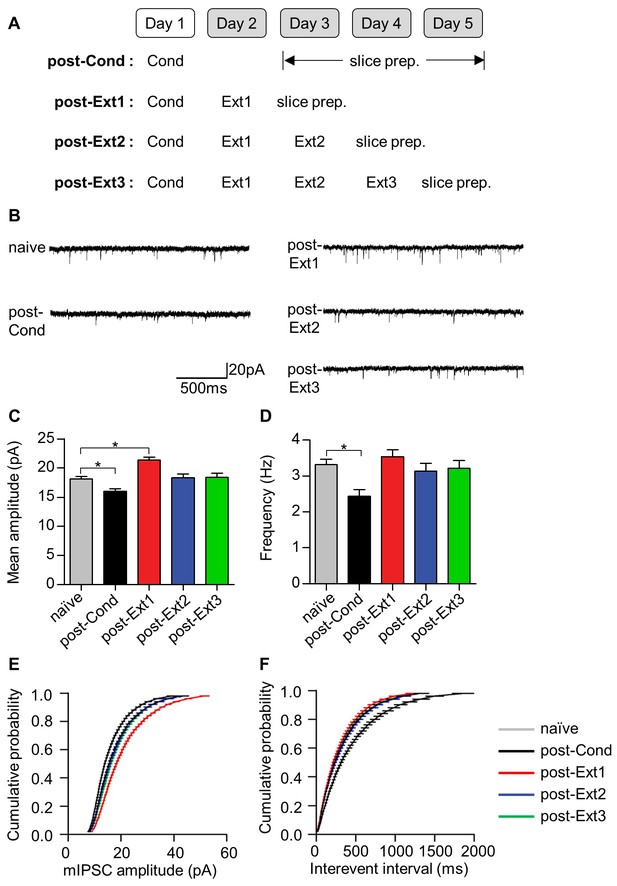
Local inhibitory tone in the LA increases after single-session extinction-training and returns to baseline after multiple-session extinction-training.
(A) Experimental design. (B) Representative traces of mIPSCs for each behavioral group. (C–D) Changes in mIPSC amplitude (C) and frequency (D) with fear conditioning and subsequent extinction training. Significant intergroup differences were detected for the mean amplitudes (χ2 = 46.92, p<0.0001, p<0.05 for naïve vs. post-Cond and post-Ext1, Kruskal-Wallis test followed by Dunn’s test) and the frequencies (χ2 = 17.93, p=0.0013, *p<0.05 for post-Cond versus naïve, Kruskal-Wallis test followed by Dunn’s test). (E–F) The cumulative histograms of mIPSC amplitude (E) and frequency (F). Significant intergroup differences were detected for mIPSC amplitude (D > 0.1, p<0.0001 for post-Cond, post-Ext1 vs. naïve, p>0.05 for post-Ext2, post-Ext3 vs. naïve, Kolmogorov-Smirnov test) and mIPSC frequency (D > 0.1, p<0.0001 for post-Cond vs. naïve, Kolmogorov-Smirnov test).
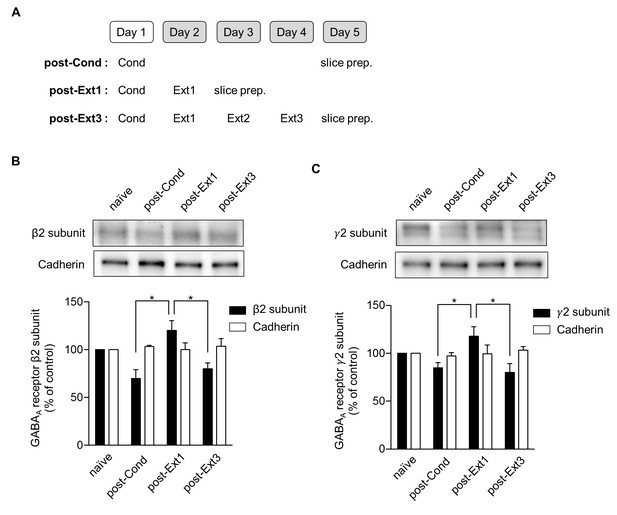
Surface expression of the β2 and γ2 subunits of γ-aminobutyric acid (GABA)A receptors increases after single-session and decreases after multiple-session extinction training.
(A) Experimental design. (B) Surface expression of the β2 GABAA receptor subunit and cadherin in the naïve, post-Cond, post-Ext1 and post-Ext3 groups. Surface expression levels of the β2 GABAA receptor subunit after behavioral training (post-Cond, 69.76 ± 9.260%, n = 4 from 12 rats; post-Ext1, 120.2 ± 10.25%, n = 4 from 12 rats; post-Ext3, 80.07 ± 5.919%, n = 4 from 12 rats; χ2 = 10.37, p=0.0030, *p<0.05 for post-Ext1 vs. post-Cond and post-Ext3, Kruskal-Wallis test followed by Dunn’s test) and cadherin (post-Cond, 103.2 ± 1.164%, n = 4 from 12 rats; post-Ext1, 100.1 ± 7.018%, n = 4 from 12 rats; post-Ext3, 103.7 ± 7.733%, n = 4 from 12 rats;χ2 = 1.164, p=0.7887, Kruskal-Wallis test) were expressed as a percentage of the expression in naïve controls. (C) Surface expression of the γ2 GABAA receptor subunit and cadherin in the naïve, post-Cond, post-Ext1 and post-Ext3 groups. Surface expression levels of the γ2 GABAA receptor subunit after behavioral training (post-Cond, 84.87 ± 5.364%, n = 4 from 12 rats; post-Ext1, 117.8 ± 10.09%, n = 4 from 12 rats; post-Ext3, 80.04 ± 9.232%, n = 4 from 12 rats; χ2 = 8.149, p=0.0253, *p<0.05 for post-Ext1 vs. post-Cond and post-Ext3, Kruskal-Wallis test followed by Dunn’s test) and cadherin (post-Cond, 97.10 ± 3.576%, n = 4 from 12 rats; post-Ext1, 99.38 ± 9.383%, n = 4 from 12 rats; post-Ext3, 103.4 ± 3.761%, n = 4 from 12 rats; Kruskal-Wallis test, χ2 = 0.6045, p=0.9109) levels were expressed as a percentage of the levels in the naïve controls.
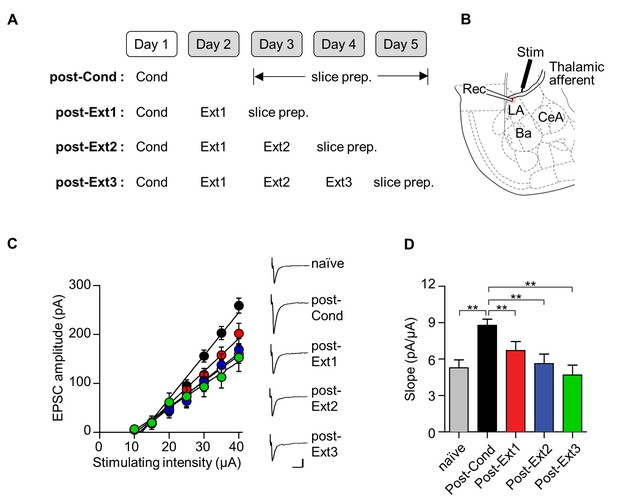
Conditioning-induced synaptic potentiation at T-LA is depotentiated after single- or multiple-session extinction-training.
(A) Experimental design. (B) Placement of recording and stimulating electrodes. (C, D) Input-output curves (C) and slopes (D) show that synaptic strength at T-LA synapses was depotentiated after multiple-session extinction training as well as single-session extinction training (naïve, 5.28 ± 0.65 pA/μA, n = 21 cells; post-Cond, 8.79 ± 0.5 pA/μA, n = 47 cells; post-Ext1, 6.71 ± 0.73 pA/μA, n = 34 cells; post-Ext2, 5.63 ± 0.78 pA/μA, n = 22 cells; post-Ext3, 4.68 ± 0.82 pA/μA, n = 12 cells; χ2 = 24.84, p<0.0001, **p<0.005 for post-Ext1 vs. the other groups, Kruskal-Wallis test followed by Dunn’s test). Representative current traces are an average of 4–5 consecutive responses with input stimulations of 35 μA. Scale bars, 50 pA x 20 ms.
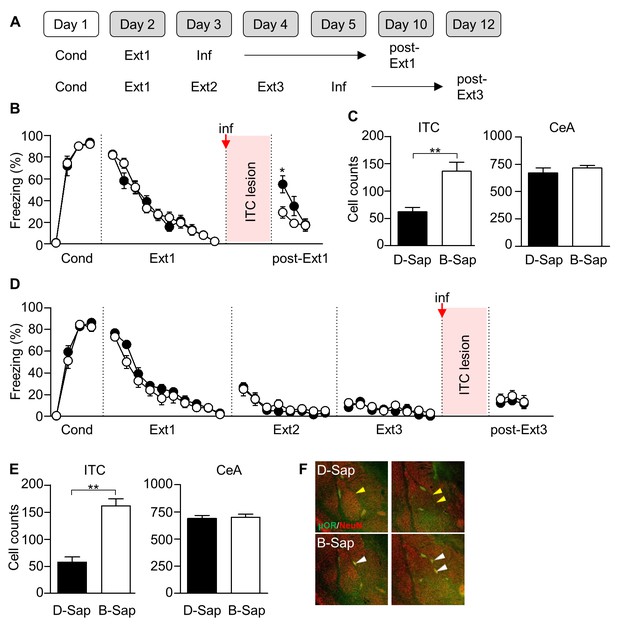
ITC lesions impair extinction recall after single-session extinction-training, but not after multiple-session extinction-training.
(A) Experimental design. Inf, infusion. (B) CS-induced freezing in ITC-lesioned rats (D-Sap, n = 13 rats, black) and controls (B-Sap, n = 13 rats, white). ITC lesion prevented extinction recall after single-session extinction (U = 34.5, p=0.009, Mann-Whitney U test). *p<0.05. ITC, intercalated amygdala neurons. (C) Number of NeuN-positive cells in ITC (D-Sap, 62.25 ± 7.549, n = 12 rats; B-Sap, 136.6 ± 16.31, n = 10 rats; U = 11, p=0.0006, Mann-Whitney U test) and CeA (D-Sap, 671.3 ± 45.51, n = 6 rats; B-Sap, 717.3 ± 23.41, n = 6 rats; U = 11, p=0.3095, Mann-Whitney U test). **p<0.001. CeA, central amygdala. (D) CS-induced freezing in ITC-lesioned rats (D-Sap, n = 19 rats, black) and controls (B-Sap, n = 15 rats, white). ITC lesion did not affect extinction recall after multiple-session extinction (U = 141.5, p=0.9793, Mann-Whitney U test). (E) Number of NeuN-positive cells in ITC (D-Sap, 57.93 ± 9.42, n = 15 rats; B-Sap, 161.6 ± 13.18, n = 10 rats; U = 11, p=0.0006, Mann-Whitney U test) and CeA (D-Sap, 689.0 ± 27.80, n = 6 rats; B-Sap, 700.8 ± 28.10, n = 6 rats; U = 15, p=0.6991, Mann-Whitney U test). **p<0.001, Mann-Whitney U test. (F) Staining of μORs (green) and neurons (NeuN, red) in rats infused with D-Sap (upper) or B-Sap (bottom). White arrow heads indicate non-affected ITC clusters in rats that received the B-Sap infusion, whereas yellow arrow heads indicate reduced ITC clusters in the adjacent site to the infusion.
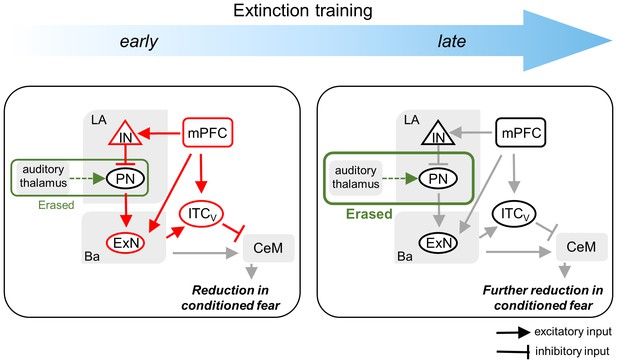
Schematic diagram for the changes in extinction mechanisms as extinction training proceeds.
Please note that green and red lines represent the inhibition and erasure mechanisms, respectively. As extinction training proceeds from the early to the late phase, the inhibition mechanism disappears whereas the erasure mechanism persists. The erasure mechanism at the early phase is depicted as smaller than at the late phase in order to show that relative contribution of the erasure mechanism to extinction is less at the early phase than at the late phase.
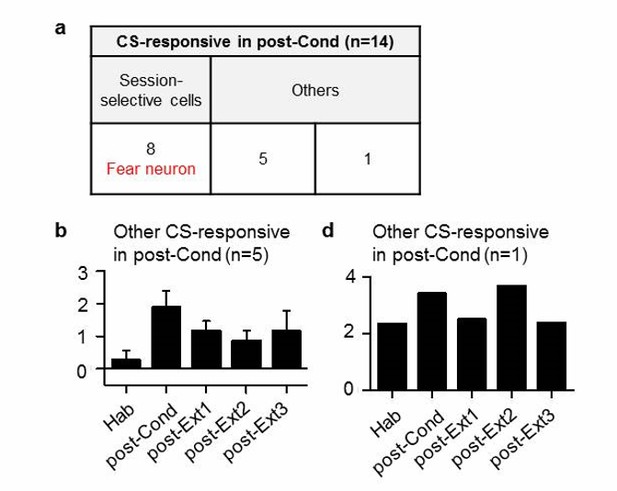
Cell-based analysis of Ba neurons which were CS-responsive in post-Cond session.
https://doi.org/10.7554/eLife.25224.015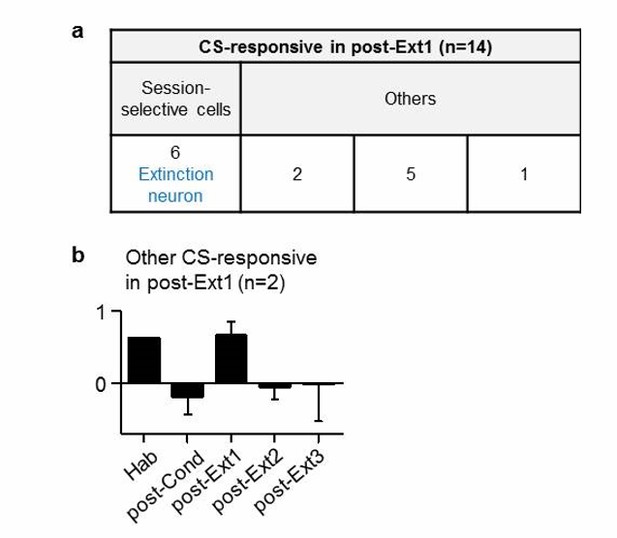