Induced sensorimotor cortex plasticity remediates chronic treatment-resistant visual neglect
Figures
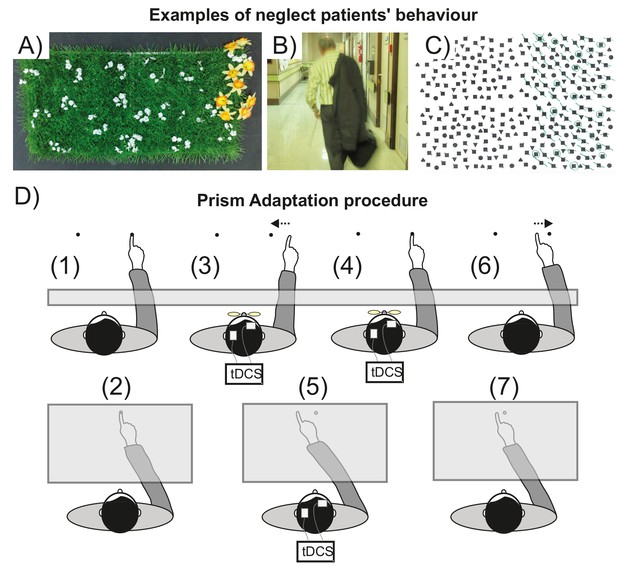
Visuospatial neglect and prism adaptation.
(A–C) Examples of neglect behavior by patients in the present study. (A) Gardening task: arrange the flowers uniformly around the garden; (B) patient has neglected the left of his body; (C) Ota-Girardi task: cancel all targets on the page. (D) Prism Adaptation. (1) Baseline: visual feedback. Participants make rapid centre-out pointing movements to targets located at 10 degrees left or right. Vision of the hand start position is occluded. (2) Baseline: no visual feedback. Participants point at a central target. Vision of the hand is occluded throughout. (3) Adaptation: early prism exposure. Participants repeat (1) wearing 10° right-shifting prisms and initially make large rightward pointing errors. They use error feedback to correct their movements from right to left. (4) Adaptation: late prism exposure. After sustained prism exposure participants successfully realign hand-eye coordination leftward to regain baseline accuracy (i.e. they adapt). (5) Adaptation: prism after-effect. As participants adapt, this induces a leftward bias (prism after-effect), measured as the change from baseline (2). (6) Washout. After prism removal when participants point as in (1), the effect of adaptation is seen as a leftward error that is quickly corrected to restore baseline accuracy. (7) Retention. The magnitude of any remaining prism after-effect is measured as in (2).
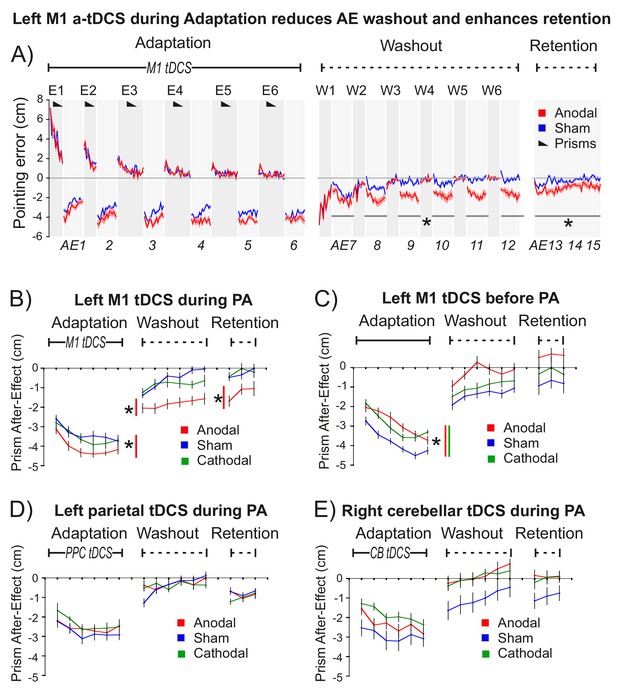
M1 a-tDCS during Prism Adaptation (PA) enhanced consolidation of the prism after-effect.
The x-axis represents baseline accuracy (zero error), with prism after-effect (AE) data representing changes from baseline. For each panel N = 9. Asterisk indicates significant difference between Anodal and Sham (p<0.05). (A) Pointing accuracy in healthy volunteers when anodal (red) or sham (blue) tDCS was applied to M1 during Adaptation (Experiment 1). Black wedges indicate blocks throughout which prisms were worn. During Adaptation (prism exposure, (E1–E6) and Washout (prisms removed, (W1–W6), participants saw the outcome of the trial, so could correct their errors. The AE was measured without visual feedback (AE1-15, shaded light grey). Solid lines show pointing accuracy averaged across participants (shading =±1 SEM). Adaptation and Washout lasted 20 min each. Retention lasted 6 min after 10 min of blindfolded rest. Relative to sham, anodal tDCS increased AE persistence throughout Washout and Retention: note the leftward shift in AE7-15 (no visual feedback), whereas accuracy in interleaved blocks W1-6 is indistinguishable. (B–E) Prism after-effect in different stimulation conditions. Panel (B) summarizes group mean AEs (±1 SEM) for the dataset shown in (A) (AE1-15 only) and also shows data for reversed polarity stimulation (cathodal, green). Other panels plot the same summary AE data for stimulation of: (C) M1 before Adaptation (Experiment 2); (D) right posterior parietal cortex (PPC) during Adaptation (Experiment 3); E) right cerebellum (CB) during Adaptation (Experiment 4).
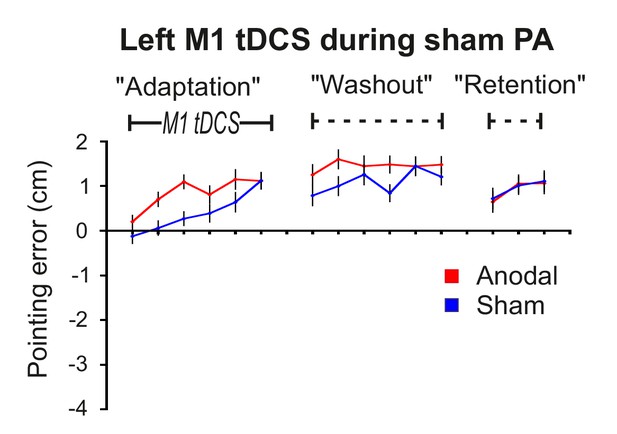
Control experiment 5: M1 a-tDCS alone does not cause a leftward shift in pointing behavior.
Data are group mean open-loop pointing errors (±1 SEM; N = 10) for sham and anodal tDCS conditions in the identical behavioural protocol to that shown in Figure 2 (ie: Adaptation, Washout, Retention) except that sham (not prism) glasses were used (Experiment 5). There is no leftward shift in pointing behavior in either condition. This confirms that M1 a-tDCS alone does not induce the sustained leftward shift in pointing behavior shown in Figure 2A,B and Figure 3.
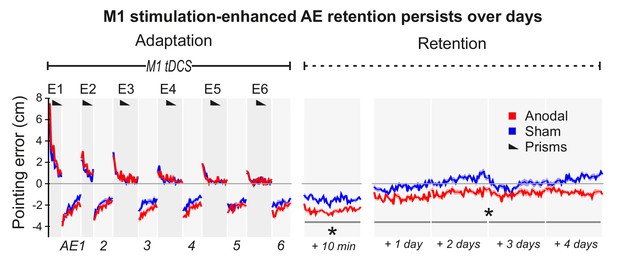
M1 a-tDCS during Adaptation enhanced prism after-effect retention across days.
Pointing errors in healthy volunteers (N = 10) when anodal (red) or sham (blue) stimulation was applied during Adaptation (Experiment 6). Compared to the experiment of Figure 2A, there was no active washout phase. Instead, retention was measured daily over 5 days after adaptation. Solid lines show pointing accuracy averaged across participants (±1 SEM, shading). As in the experiment shown in Figure 2A, the AE persisted throughout the measured retention period only when M1 a-tDCS had been applied during Adaptation. Asterisks indicate significantly larger AEs in the anodal versus sham condition throughout Retention (p<0.05).
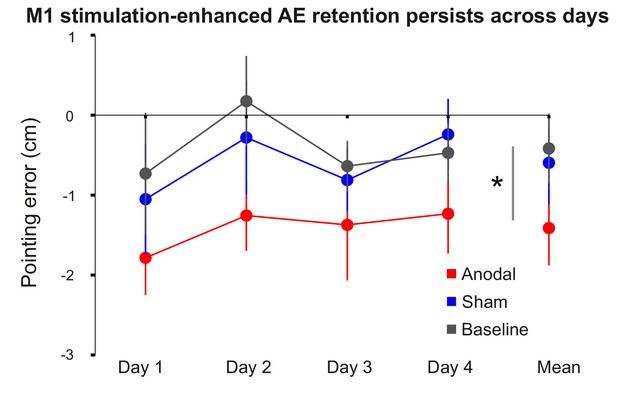
M1 stimulation-enhanced retention persists across days.
Figure shows group mean open-loop pointing errors (±1 SEM) over 4 days prior to prism adaptation (baseline), and 4 days after PA +sham tDCS (blue) or anodal tDCS (red). Pointing is significantly left-shifted in the 4 days after M1 anodal tDCS compared to both the sham and baseline conditions. Asterisk reflects p<0.05.
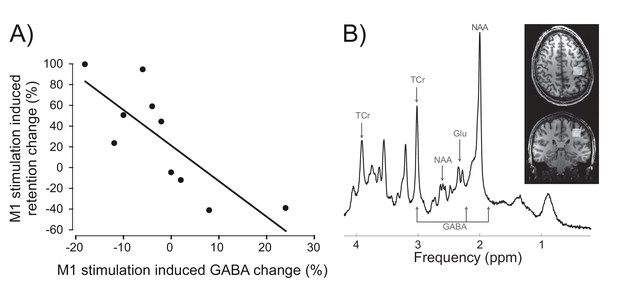
Inter-individual differences in neurochemical response to stimulation (M1 GABA change) co-varied with changes in prism after-effect retention caused by M1 a-tDCS.
(A) Experiment 7: X-axis shows individuals' percent change in GABA concentration after M1 a-tDCS (post-pre), quantified as ratios of total creatine. Y-axis shows individuals' percent change in AE retention 24 hr after PA + tDCS (anodal-sham). Correlation (N = 10) shows quantitative covariation between the behavioural and neurochemical effects of M1 a-tDCS. Note the data pass through the origin. (B) Representative image for a single participant showing voxel placement in the hand knob region of M1 and spectral quality. Frequency spectrum labels (ppm = parts per million) indicate peaks for GABA, Glutamate (Glu), total Creatine (TCr = creatine + phosphocreatine) and N-acetylaspartate (NAA).
-
Figure 4—source data 1
Individual participants' magnetic resonance spectroscopy data for M1 and occipital cortex before and after stimulation.
Table shows data from Experiment 7: estimated metabolite concentrations for GABA and Glutamate, as ratios of total creatine (Cr), as analysed by LC Model, with their associated uncertainty estimates (CRLB, cramer-rao lower bound scores), and spectral quality measures of signal-to-noise ratio and linewidth for each scan, together with estimates of grey matter, white matter and cerebrospinal fluid in each measurement voxel, and partial volume-corrected metabolite estimates. The change (post-pre tDCS) in partial volume-corrected metabolite estimates was used to test for inter-individual correlation with tDCS-induced change (anodal-sham) in percent AE retention.
- https://doi.org/10.7554/eLife.26602.010
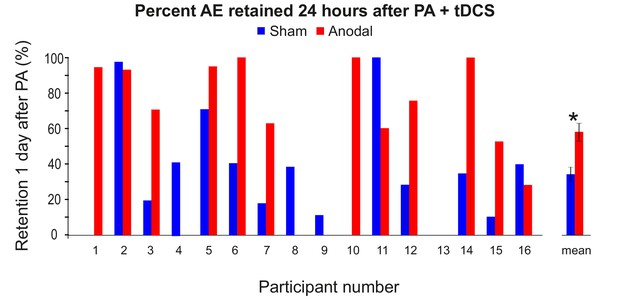
AE retention scores (%) 24 hr after PA was combined with anodal or sham tDCS in Experiment 7.
Bars represent individuals and group mean ±1 SEM (N = 16). Apparently missing bars represent zero retention. Participants 1–10 also had usable MRS data and are shown in the behavior-neurochemistry correlation in Figure 4. Figure 4 plots the anodal-sham difference in retention ratios.
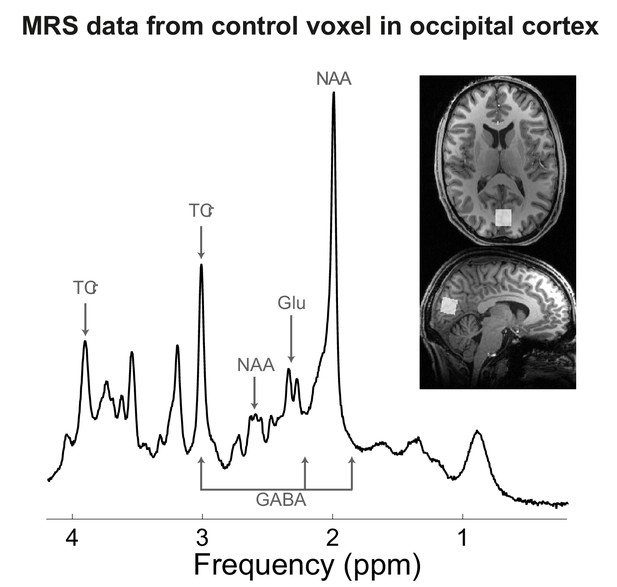
MRS data from occipital cortex voxel in Experiment 7.
Representative image for a single participant showing voxel placement in the occipital cortex (control region). Frequency spectrum labels (ppm = parts per million) indicate peaks for GABA, Glutamate (Glu), total Creatine (TCr = creatine + phosphocreatine) and N-acetylaspartate (NAA).
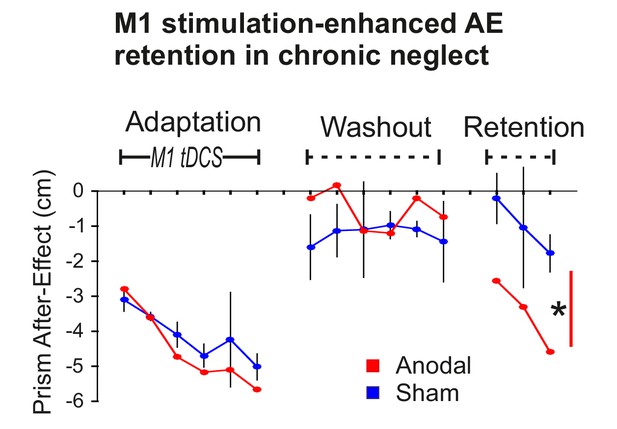
Stimulation-enhanced retention in chronic neglect.
The prism after-effect over time for anodal (red) and sham (blue) stimulation of M1 during Adaptation in a single case neglect patient (Patient 1). Data are displayed as described for Figure 2B except that sham data are the mean (±95% confidence intervals) across 3 sessions of the protocol, and anodal data are from a single session. AE retention was significantly enhanced by stimulation in this neglect patient, similar to the healthy volunteers shown in Figure 2A,B. Asterisk indicates significantly greater retention for the planned contrast of anodal versus sham in Retention blocks 1 and 3 (*p<0.001).
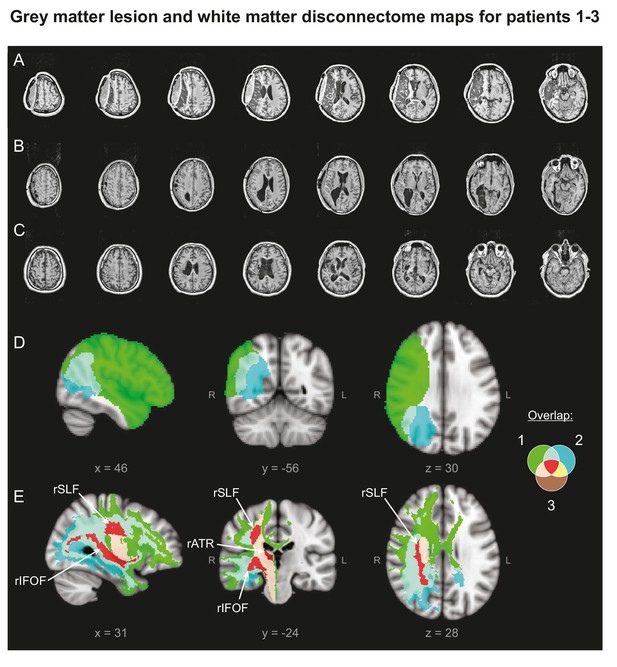
Patients' Lesion Anatomy.
(A–C) T1-weighted structural scans of Patients 1–3, respectively. (D) Grey matter lesion overlap between the three patients in group mean MNI space. (E) ‘Disconnectome map’ shows white matter damage overlap between the three patients. Red indicates white matter damage overlap in all three patients in the right inferior fronto-occipital fasciculus (rIFOF), the right superior longitudinal fasciculus (rSLF) and the right anterior thalamic radiation (rATR).
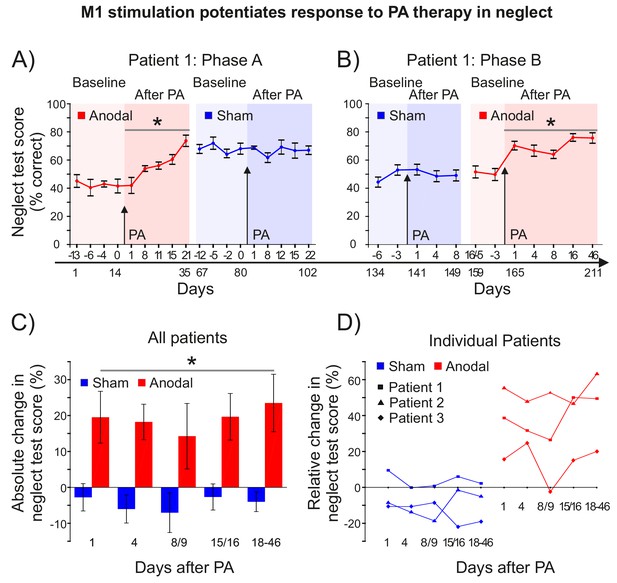
M1 stimulation during PA leads to lasting improvements in neglect.
(A) Behavioural measures of neglect in a single case chronic neglect patient (Patient 1) before and after PA sessions combining M1 anodal (red) and sham (blue) stimulation during adaptation. Datapoints are mean % neglect score ±1 SEM. A score of ~50% indicates the patient completed the rightmost half of the six tests in the battery. (B) As (A), but with a different battery of 10 neglect test measures, reversal of the order of anodal and sham stimulation, and no Washout phase during prism adaptation. (C, D) Percentage changes in performance on a battery of neglect tests over time after PA +M1 a-tDCS, averaged across all three patients (±1 SEM) (C), and for each individual case (D). Asterisks indicate significant improvement in neglect score after PA +M1 a-tDCS compared to sham (p<0.05).
-
Figure 7—source data 1
Individual patients' percent correct scores on neglect batteries before and after PA + tDCS at each timepoint and test phase.
Table shows percent correct scores for each patient on the subtests of the neglect batteries at each timepoint and experimental phase. The higher the score, the more leftward the performance. For Patient 1 Phase B, the two Anodal pre-test sessions (asterisked) also served as late post-test sessions for the sham phase. For Patient 1 Phase A the seven underlined datapoints were missing and have been replaced by the average of the two nearest timepoints for the same experimental phase (eg: for Anodal pre −13 days, the average of Anodal pre −6 and −4 was used).
- https://doi.org/10.7554/eLife.26602.015
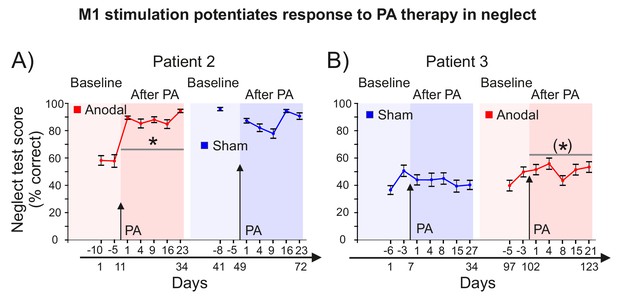
M1 stimulation during PA leads to lasting improvements in neglect.
(A) Behavioural measures of neglect in Patient 2 before and after PA sessions combining M1 anodal (red) and sham (blue) stimulation during adaptation. Data are mean % neglect score ±1 SEM. A baseline score of ~60% indicates the patient completed the rightmost two thirds of the 10 tests in the battery. One of the sham baseline scores is missing for Patient 2 as he forgot to attend testing. Asterisk indicates significant improvement in neglect score after PA +M1 a-tDCS compared to sham (p<0.05). (B) As (A), but for Patient 3, who underwent the reversed order of PA +anodal versus sham stimulation. Bracketed asterisk indicates significant improvement in neglect score after PA +M1 a-tDCS, but the contrast of anodal versus sham was not significant.
Additional files
-
Supplementary file 1
Summary of effect sizes for all a priori predictions in the paper.
A > S refers to the predicted greater effect of M1 anodal tDCS (A) compared to sham (S). Each row describes the relevant figure, sample, 1-tailed hypothesis, statistical significance level, result (F, t, p) and effect size in both raw measurement units (mean difference, standard error of the mean, SEM, Spearman's r) and standardized units (partial eta squared η2p, Cohen's d), alongside published benchmark interpretations of the magnitude (Sawilowsky, 2009) and practical significance (Wolf, 1986) of Cohen's d effect sizes.
- https://doi.org/10.7554/eLife.26602.016
-
Supplementary file 2
Individual patients' reach kinematics before and after PA + tDCS.
Patients 1 and 2 performed the task with the right arm once each in the days before and after PA was combined with M1 anodal or sham tDCS. Patient 3 underwent the anodal condition only, but with both the non-paretic (right) arm and the recovered paretic (left) arm. Table lists individual trial data plus means and standard deviation for all three patients. Accuracy data show the number of trials of the total (11) that were correct, were missed (no response to the 'go' cue), were too slow (outliers = RT and/or MT ± 2 SD of the patient's mean for that condition), or in which the patient subsequently placed the ball in the wrong basket (i.e. the left following a right pre-cue, and vice versa). Only the centre-out phase of the reach was analysed. PA combined with anodal tDCS (compared to sham) speeded centre-out reach movement time, regardless of whether the central pre-cue instructed a subsequent leftward or rightward movement after grasping the ball. Kinematic parameters characterize both the transport component [Reaction time (RT), Movement Time (MT), Peak Velocity (PV), Time to Peak Velocity (TPV), Maximal Height of Wrist and Elbow (MHW, MHE)] and the grasp component [Maximum Grip Aperture (MGA), Time of Grip Aperture Onset (TGA), Time of Maximum Grip Aperture (TMGA)] of the centre-out reaching movement required to grasp the tennis ball.
- https://doi.org/10.7554/eLife.26602.017
-
Transparent reporting form
- https://doi.org/10.7554/eLife.26602.018