Point of View: Unbridle biomedical research from the laboratory cage
Abstract
Many biomedical research studies use captive animals to model human health and disease. However, a surprising number of studies show that the biological systems of animals living in standard laboratory housing are abnormal. To make animal studies more relevant to human health, research animals should live in the wild or be able to roam free in captive environments that offer a natural range of both positive and negative experiences. Recent technological advances now allow us to study freely roaming animals and we should make use of them.
Main text
For over 50 years, biologists have asked how the addition of a few objects to a laboratory cage can change the biology of a laboratory rodent. We have learned that even modest amendments to cages lead to vast changes in the neurobiology, behavior, immune responses, disease resistance and cancer remission of captive mice and rats. This so-called environmental ‘enrichment’ makes research animals more resilient and helps them to recover from many kinds of experimental treatments. These studies often conclude that enrichment serves as a form of therapy, but they overlook an important point. The cage floor areas provided to rodents and primates used in research are much smaller than their natural ranges. The captive environments of research animals also deny them ongoing opportunities to explore and learn from the variety of rewards and challenges that they would normally experience in the wild.
By studying organisms confined to restricted environments that are unresponsive to their actions, biomedical research violates one of its core propositions – that animals used as experimental controls embody healthy biological systems. We now have the technologies to study free-roaming organisms in the wild or in captivity under naturalistic conditions. Advances in remote recording and transmission of data (telemetry) allow us to transfer data wirelessly so we can modulate the physiology of free-roaming animals and record their responses. By using these technologies, we can provide research animals with a greater sense of wellbeing and make animal studies more relevant to human health and disease.
Captivity alters animal development
In the early 1960s, Mark Rosenzweig and his colleagues asked how environmental changes affected the brains of his laboratory rats. Each day, they placed new wooden blocks inside the rats’ cages and let the rats explore mazes that were reconfigured every day. The team discovered that the sensory cortex, a region of the brain that processes all of the senses (sight, sound, touch, smell and taste), was larger in these experimental rats than in the rats living in standard laboratory cages (Rosenzweig et al., 1962).
Since then, researchers have learned that even modest changes to the conditions in a standard laboratory cage – such as the one-time addition of objects, enhanced maternal care, or more frequent handling – lead to changes in the animals’ brains. This enrichment alters the densities and appearance of neurons (Kempermann et al., 1997; Greenough et al., 1973), other brain cells (Szeligo and Leblond, 1977; Viola et al., 2009) and blood vessels (Black et al., 1987). Strikingly, enrichment promotes changes in some regions of the brain, but not others (Szeligo and Leblond, 1977; Faherty et al., 2003). This structural reorganization is mediated by local differences in gene expression (Pham et al., 1999) that can persist throughout life (Thiriet et al., 2008; Meaney, 2010; Mychasiuk et al., 2012).
Laboratory animals are less sensitive to experimental procedures when they have something to do. For example, enrichment can help animals to recover from brain trauma and seizures (Passineau et al., 2001; Koh et al., 2007), reverse brain damage caused by exposure to chemicals (Goldberg et al., 2011; Guilarte et al., 2003; Shih et al., 2012), and make rodents less susceptible to the effects of recreational drugs (Stairs and Bardo, 2009; Solinas et al., 2010). These studies have prompted most researchers to conclude that enrichment serves as a form of therapy (Lahvis, 2016a).
Critically, the term enrichment implies that the conditions imposed by standard laboratory housing are somehow normal. Laboratory cages offer poor environments relative to the natural conditions where our research animals evolved. For example, the floor area provided to a mouse inside a standard cage is 280,000 fold smaller than its natural home range. This difference becomes even more extreme for larger animals used in research such as rhesus macaques (7 million fold) and Anubis baboons (25 million fold; Figure 1).
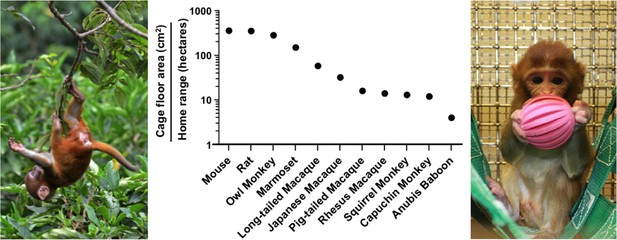
Laboratory cages are much smaller than an animal’s natural home range.
The middle panel shows the ratio of the recommended cage floor area (cm2; Albus, 2012) to the estimated natural home range in hectares (10,000 m2; Nunn and Barton, 2000; Chambers et al., 2000; Bramley, 2014) for eleven species used in research. The left panel shows a juvenile rhesus macaque in a Nepali jungle. The right panel shows a juvenile rhesus macaque in an enriched cage.
IMAGE CREDIT: photo of the caged juvenile macaque courtesy of the University of Wisconsin Primate Center.
An essential role of the brain is to adjust behavior to an unpredictable environment, an everyday experience common to wild animals and humans. By contrast, even enriched cage environments offer none of the temporal variations occurring in nature, such as changes in temperature and humidity, the availability of food and shelter, and risks to survival. These comforts and adversities evoke a wealth of feelings, including pain, fear, hunger, anticipation and pleasure. The caged environment, whether standard or enriched, isolates the research animal from these everyday experiences.
We know that depriving research animals of physical experiences and visual stimuli decreases their respective activities in the motor and visual cortex (Ostrovsky et al., 2006; Strata et al., 2004). Likewise, it is untenable to assume that a brain deprived of a complex environment of rewards and challenges represents that of a healthy animal or human.
Responding to a responsive environment
By contrast to what happens in a laboratory cage, wild animals behave in ways that aim to optimize energy uptake or minimize the time they spend foraging (Krebs and Davies, 2009). An animal must consume more energy than it expends (Fonseca et al., 2014; Belovsky, 1984) and the balance of this relationship is sensitive to other factors, such as risks of predation (Hoogland, 1981), requirements for nutrients (Belovsky, 1978) or the temporary need for medicinal plants (McPherson, 2013). Optimal foraging models account for an animal’s moment-to-moment decisions of what paths to take, what foods to eat and how much time to spend foraging versus watching for predators.
Humans and other animals make decisions based on our ‘affective’ experiences: we seek rewards and avoid situations that we feel are unpleasant or potentially harmful (Schneirla, 1959). Within a complex and unpredictable natural environment, an organism learns what cues and contexts are associated day-to-day with rewarding or unpleasant situations. These affective experiences inform and respond to ongoing decisions.
Consider, for example, the temporary and patchy distributions of food, such as fruits ripening and decaying, that are thought to have favored the evolution of primate intelligence (Navarrete et al., 2016). A fruit-eating primate must make a cognitive decision, choosing a course of action from various alternatives in the context of its affective experience, such as the desire to taste a particular kind of fruit. In nature, a primate might abstain from eating local food items to follow spatial cues to more nutritious or more flavorful foods at distant locations. As these distant foods decay or become depleted, the primate must then learn that the spatial cues are no longer predictive. Even under simpler foraging conditions, individual animals of many species must learn and relearn which spatial cues predict the locations of food items that are only available in particular places for short periods of time.
Wild animals also consider various factors in decisions not directly related to foraging. These include habitat selection (Raynor et al., 2017), mate selection (Candolin, 2003), cooperation with other individuals (Lahvis, 2016b), and predators (Lagos et al., 2009). Such challenges are common for wild animals (Krebs and Davies, 2009) and resemble the everyday conflicts of human experience. By contrast, laboratory animals are denied opportunities to make decisions within a natural context that offers a breadth of spatial and temporal variety.
Some argue that laboratory mammals, particularly research primates living in open colonies, have opportunities to navigate complex social interactions inside their corrals and thus experience challenges that are akin to those of their wild counterparts. However, conservation biologists find that captive-raised animals reintroduced into the wild – such as California condors, spotted owls and Pacific salmon – fail to express the cognition, motivation and behaviors necessary to survive and reproduce (Reading et al., 2013).
Some argue we need to adhere almost exclusively to studies of caged animals to control irrelevant environmental variables that, if left unchecked, complicate the delicate cause-effect relationships governing development. Yet, we often lack or fail to control many of the most important environmental variables inside laboratory cages, such as food and bedding. Standard housing fosters increased sensitivity to stimuli that researchers have not noticed or find difficult to control. For example, variations in animal feed (Garey et al., 2001; Brown and Setchell, 2001), bedding (Burn et al., 2006; Robinson et al., 2004), the sex of the experimenter (Bohlen et al., 2014; Sorge et al., 2014), or ultrasonic noises (Turner et al., 2005; Milligan et al., 1993) can render behavioral tests difficult to replicate. Indeed, mouse behaviors are difficult to reproduce across laboratories in standard cages (Crabbe et al., 1999; Richter et al., 2010) and are more reproducible when housing conditions are varied (Würbel, 2002; Richter et al., 2009, 2010).
Not just neurobiology
Relative to laboratory animals housed in enriched cages, animals housed in standard cages express greater levels of cortisone, a hormone that affects immune cells and impairs immune responses throughout the body. These changes reduce the ability of a captive animal to mount an effective response to infections (Arranz et al., 2010; Gurfein et al., 2014). Conventional caging also promotes obesity, type II diabetes and high blood pressure (Martin et al., 2010), while decreasing muscle strength and endurance (Sirevaag and Greenough, 1987; Spangenberg et al., 2005; During et al., 2015). Furthermore, standard laboratory housing enhances tumor growth in animal models of several cancers (Cao et al., 2010; Li et al., 2015; Nachat-Kappes et al., 2012). The ‘control’ animals used in such experiments do not represent what biomedical research requires for its premise; they are not healthy individuals.
Few studies have compared the biology of wild versus cage-reared animals. Among caged prairie voles, variations of a gene encoding the receptor for a hormone called vasopressin are highly correlated with sexual fidelity (Okhovat et al., 2015). However, these correlations are not nearly as obvious among voles living in the wild. A different study shows that the structure of the visual cortex in caged Norway rats differs remarkably from that of their free-roaming counterparts (Campi et al., 2011). Wild animals also express lower levels of cholesterol than captive animals (Schmidt et al., 2006; Eades et al., 1963). Recently, a subset of immune cells called T cells that are expressed by humans but not found in laboratory mice, was identified in feral mice. The report stated that feral mice have “immune systems closer to those of adult humans” (Beura et al., 2016).
Research into animal wellbeing
Wellbeing assumes a freedom to engage actively and proactively with responsive surroundings, exploring, problem-solving, and learning to deal skillfully and flexibly with new and existing challenges (Špinka and Wemelsfelder, 2011). Animal welfare scientists assert that non-human animals are intrinsically motivated to be ‘doers’ or ‘authors’ of their own activities. They argue that animals can be motivated to sample their environments, exposing themselves to nominal risks and engaging with obstacles to be overcome (Špinka and Wemelsfelder, 2011).
In support of these ideas, numerous studies suggest that laboratory animals prefer complex environments to predictable housing conditions. For example, animals prefer to spend more time in enriched environments than in standard environments (Bayne et al., 1992; Bevins et al., 2002; Schroeder et al., 2014). Rats press a lever more frequently for food rewards allocated at unpredictable levels than for regular aliquots (Anselme et al., 2013).
Classical conditioning approaches, used to determine whether an animal finds a stimulus rewarding or unpleasant (Bardo and Bevins, 2000; Pellman and Kim, 2016), show that laboratory rats prefer an environment where they have experienced repeated access to new objects over an environment previously paired with familiar objects (Bevins et al., 2002). These preferences are driven by the animal’s affective experiences and, in this case, interest or enjoyment felt inside the environment paired with the new object. These affective experiences are controlled by well-defined reward circuits in the brain (Bevins et al., 2002; Panksepp, 1998).
Subjective experiences are known only to the individuals that have them (Dawkins, 2015). Language can only give us inferences to the subjective experiences of humans. For instance, if we both use the word ‘red’ to describe what we see in a ripe apple, our shared use of the same word suggests a common experience even if we both see a different color when we look at the same ripe apple (Russell, 1912). For humans and other animals, we can sometimes infer subjective experiences from variations in gait, gesture, facial expression and vocal intonation (Darwin, 1872; Panksepp, 1998). We can also make inferences from measures of physiological responses in the brain (e.g. Kelley, 2005), or of systemic responses, such as changes in the level of a stress hormone called cortisol in the body (Detillion et al., 2004). When we use a variety of approaches, we can infer that cages have adverse influences on the subjective experiences of captive animals (Boissy et al., 2007; Yeates and Main, 2008).
We can also have confidence that subjective experiences of humans and other animals lie on a continuum (De Waal, 2016; Panksepp, 1998). For humans, captivity inside a cage is punishment. Just as imprisonment deserves ethical consideration, we have an ethical responsibility to improve the conditions of captivity that we impose on research animals (Gruen, 2014). If these animals were afforded natural levels of environmental complexity, an expectation is that they would rarely attend to the outside boundaries of their cages.
Within a monotonous and predictable captive environment, where confinement is unresponsive to action, laboratory animals are deprived of their natural agency. Instead, the animals are left with boredom and, with time, learned helplessness and depression (Špinka and Wemelsfelder, 2011).
What can we do?
We should replace our study of caged animals with studies of animals living in the wild or under captive but naturalistic conditions. Recent advances in technology allow us to bring traditional biomedical research to the study of freely roaming animals. For many years, wild organisms have been employed as sentinels of the biological effects of unanticipated ‘real-world’ exposures to complex pollutant mixtures (Fox, 2001; Basu et al., 2007; Ramalhinho et al., 2012). With new technologies, we can also learn how genes and gene-by-environment interactions contribute to individual differences in disease susceptibility and resistance.
It is now possible to track free-roaming animals across large areas. We can record their behaviors with low-cost camera traps (O'Connell et al., 2010), lightweight radio frequency identification transponders (König et al., 2015) and integrated applications of accelerometers, magnetometers, and pressure sensors (Sommer et al., 2016). Data can be transmitted by WiFi (Su et al., 2015), GPS (Jawalkar et al., 2017), and ultra-low power sensors (Dressler et al., 2016). We can record the electrical activity of the brain in freely moving animals, such as the mid-flight sleep patterns of frigate birds (Rattenborg et al., 2016) or the activities of neurons in wild unrestrained rats (Szuts et al., 2011).
Miniaturized optics are available to conduct real-time, optical imaging (Yu et al., 2015) and optogenetics can remotely alter neuron activity (Tye and Deisseroth, 2012). We can use optofluidic neural probes to remotely modulate gene expression and deliver viruses, peptides, and drugs to non-human animals (Jeong et al., 2015). It is also possible to remotely monitor heart rate and the concentration of various molecules in tissue (Bazzu et al., 2009; Rutherford et al., 2007). Furthermore, advanced statistical approaches can be used to identify genetic and environmental factors that contribute to individual differences in biology and behavior (de Boer et al., 2017; Nussey et al., 2007).
Other advances include developing smaller sensors that are less invasive when implanted into animals and applying non-invasive imaging tools to research on small animals (Jang, 2013). These advances, along with the proposed improvements to environmental conditions, might be sufficient to improve the wellbeing of research animals and also provide relevant insight to a range of biological processes.
Some experiments would be difficult to conduct under wild conditions, such as manipulations that cause illness. In these cases, enclosed naturalistic environments would be necessary to give researchers the ability to rapidly identify and retrieve sick animals. Captivity would also be necessary for gene-targeted animal models that, if released, may pose a threat to the environment.
In the figure, calculations of the collapse of space available to a laboratory rat or mouse were based upon estimates of their natural home ranges (Figure 1). However, feral mice and rats often live adjacent to humans where food resources are concentrated. For mice, a ‘naturalistic’ captive environment might entail simulated barn-like arenas. Studies of rodents living in complex environments date back to experiments by Peter Crowcroft that were conducted in large unheated enclosures containing hundreds of small objects and wooden housing structures (Crowcroft, 1966). A more contemporary solution to naturalistic captivity would be expanded versions of the visible burrow system (Pobbe et al., 2010). In this regard, biomedical researchers should collaborate with behavioral ecologists to develop captive environments that offer captive animals sufficient complexity and agency resembling that of their free-roaming counterparts. For species that naturally travel long distances, captivity may never adequately provide for their needs.
Another possible solution is to study smaller organisms that are less capable of realizing the limits of their captive environments. Though these organisms live in small-scale environments, natural conditions may be no less critical. For instance, zebrafish development is highly sensitive to the environmental complexity of their tanks (Spence et al., 2011), and mutants of the worm C. elegans that live twice the age of normal worms in the laboratory die earlier than their controls in natural soil (Van Voorhies et al., 2005). In these cases, biomedical researchers would benefit by collaborating with ecologists.
Alternatives to the use of caged animals also include ‘human-on-a-chip’ models, combinations of human cell types or ‘organoids’ that simulate cellular and molecular interactions and provide insight into many biological processes not otherwise obtainable (Kilic et al., 2016; Dauth et al., 2017). These alternatives are particularly attractive for many animal welfare advocates and they hold great promise. However, cell-based models do not always serve as adequate replacements for intact animals. For instance, we cannot learn about the complex relationships between brain activity and behavior, or between immune cell activity and disease resistance, if we only study cell cultures.
Conclusions
If our goals to improve human health do justify our means of using research animals, then we should be very concerned that animals raised in captivity do not fare well in their natural environments and are hypersensitive to experimental manipulations. These inadvertent effects may impose huge costs for biomedical research as laboratory animals are often sensitive to drug treatments that are later found to be ineffective in human trials (Pound et al., 2004; Hackam and Redelmeier, 2006; Tsilidis et al., 2013; Lee and Feng, 2005). To improve our understanding of human health, we must attend to the wellbeing of our animal models.
Giving research animals agency within naturalistic environments poses difficult challenges for scientists, who have traditionally studied animals living inside cages, pens and corrals. This paradigm shift also poses difficulties for those animal welfare advocates who believe that compassionate care should minimize all temporary discomforts. Motivations for rewards can rebound after their access has been denied (Panksepp et al., 2008). The health and wellbeing of a research animal require risks and discomforts, just as for humans.
In a sense, research animals confined inside our conventional research environments resemble prisoners in the Allegory of the Cave, experiencing shadows of the real-life conditions in which they evolved (and what we attempt to model). By studying captive animals exclusively, we constrain our own abilities to understand the problems we aim to investigate. We also have a responsibility to interject the scientific process into a swelling public debate regarding the compassionate treatment of research animals. Incumbent upon us, we must identify the aspects of environmental complexity necessary for the wellbeing of our animal models, and their relevance to human health, lest we also risk attending to shadows on a cave wall.
References
-
BookGuide for the Care and Use of Laboratory Animals, Vol. 463 (8th edn)Washington (DC): National Academies Press.
-
Reward uncertainty enhances incentive salience attribution as sign-trackingBehavioural Brain Research 238:53–61.https://doi.org/10.1016/j.bbr.2012.10.006
-
Mink as a sentinel species in environmental healthEnvironmental Research 103:130–144.https://doi.org/10.1016/j.envres.2006.04.005
-
Evaluation of the preference to and behavioral effects of an enriched environment on male rhesus monkeysLaboratory Animal Science 42:38–45.
-
Diet optimization in a generalist herbivore: the mooseTheoretical Population Biology 14:105–134.https://doi.org/10.1016/0040-5809(78)90007-2
-
Summer diet optimization by beaverAmerican Midland Naturalist 111:209.https://doi.org/10.2307/2425316
-
Complex experience promotes capillary formation in young rat visual cortexNeuroscience Letters 83:351–355.https://doi.org/10.1016/0304-3940(87)90113-3
-
Assessment of positive emotions in animals to improve their welfarePhysiology & Behavior 92:375–397.https://doi.org/10.1016/j.physbeh.2007.02.003
-
Home ranges and interactions of kiore (Rattus exulans) and Norway rats (R. norvegicus) on Kapiti Island, New ZealandNew Zealand Journal of Ecology 38:328–334.
-
Comparison of area 17 cellular composition in laboratory and wild-caught rats including diurnal and nocturnal speciesBrain, Behavior and Evolution 77:116–130.https://doi.org/10.1159/000324862
-
The use of multiple cues in mate choiceBiological Reviews 78:575–595.https://doi.org/10.1017/S1464793103006158
-
BookThe Expression of the Emotions in Man and AnimalsEkman P, editors. New York: Oxford University Press.
-
Neurons derived from different brain regions are inherently different in vitro: a novel multiregional brain-on-a-chipJournal of Neurophysiology 117:1320–1341.https://doi.org/10.1152/jn.00575.2016
-
Chapter two-animal welfare and the paradox of animal consciousnessAdvances in the Study of Behavior 47:5–38.https://doi.org/10.1016/bs.asb.2014.11.001
-
Cognitive continuity: a kingdom full of special capacitiesInternational Journal of Psychology 51:11–12.
-
Social facilitation of wound healingPsychoneuroendocrinology 29:1004–1011.https://doi.org/10.1016/j.psyneuen.2003.10.003
-
From radio telemetry to ultra-low-power sensor networks: tracking bats in the wildIEEE Communications Magazine 54:129–135.https://doi.org/10.1109/MCOM.2016.7378438
-
Effect of indoor housing conditions on serum cholesterol levels of white carneau pigeonsExperimental Biology and Medicine 114:515–517.https://doi.org/10.3181/00379727-114-28719
-
A Golgi-Cox morphological analysis of neuronal changes induced by environmental enrichmentDevelopmental Brain Research 141:55–61.https://doi.org/10.1016/S0165-3806(02)00642-9
-
Exercising for food: bringing the laboratory closer to natureJournal of Experimental Biology 217:3274–3282.https://doi.org/10.1242/jeb.108191
-
Wildlife as sentinels of human health effects in the Great Lakes--St. Lawrence basinEnvironmental Health Perspectives 109 Suppl 6:853–861.https://doi.org/10.2307/3454647
-
MicroSPECT and MicroPET Imaging of small animals for drug developmentToxicological Research 29:1–6.https://doi.org/10.5487/TR.2013.29.1.001
-
Intelligent wildlife tracking using ubiquitous technological suiteInternational Journal of Synthetic Emotions 8:44–59.https://doi.org/10.4018/IJSE.2017010104
-
BookNeurochemical networks encoding emotion and motivation: an evolutionary perspectiveIn: Fellous JM, Arbib MA, editors. Who Needs Emotions? The Brain Meets the Robot. New York: Oxford University Press. pp. 29–77.https://doi.org/10.1093/acprof:oso/9780195166194.003.0003
-
Flight initiation distance is differentially sensitive to the costs of staying and leaving food patches in a small-mammal preyCanadian Journal of Zoology 87:1016–1023.https://doi.org/10.1139/Z09-089
-
BookRodent models of autism, epigenetics, and the inescapable problem of animal constraintIn: Gewirtz JC, Kim YK, editors. Animal Models of Behavior Genetics. New York: Springer Nature. pp. 265–301.https://doi.org/10.1007/978-1-4939-3777-6_9
-
BookSocial reward and empathy as proximal contributions to altruism: the camaraderie effectIn: Wöhr M, Krach S, editors. Social Behavior From Rodents to Humans. Springer International Publishing. pp. 127–157.https://doi.org/10.1007/7854_2016_449
-
Randomized phase II designs in cancer clinical trials: current status and future directionsJournal of Clinical Oncology 23:4450–4457.https://doi.org/10.1200/JCO.2005.03.197
-
Sound levels in rooms housing laboratory animals: an uncontrolled daily variablePhysiology & Behavior 53:1067–1076.https://doi.org/10.1016/0031-9384(93)90361-I
-
Parental enrichment and offspring development: modifications to brain, behavior and the epigenomeBehavioural Brain Research 228:294–298.https://doi.org/10.1016/j.bbr.2011.11.036
-
The coevolution of innovation and technical intelligence in primatesPhilosophical Transactions of the Royal Society B: Biological Sciences 371:20150186.https://doi.org/10.1098/rstb.2015.0186
-
Allometric slopes and independent contrasts: a comparative test of Kleiber’s law in primate ranging patternsThe American Naturalist 156:519–533.https://doi.org/10.1086/303405
-
The evolutionary ecology of individual phenotypic plasticity in wild populationsJournal of Evolutionary Biology 20:831–844.https://doi.org/10.1111/j.1420-9101.2007.01300.x
-
BookCamera Traps in Animal Ecology: Methods and AnalysesSpringer Science & Business Media.
-
Vision following extended congenital blindnessPsychological Science 17:1009–1014.https://doi.org/10.1111/j.1467-9280.2006.01827.x
-
Differential entrainment of a social rhythm in adolescent miceBehavioural Brain Research 195:239–245.https://doi.org/10.1016/j.bbr.2008.09.010
-
What can ethobehavioral studies tell us about the brain's fear system?Trends in Neurosciences 39:420–431.https://doi.org/10.1016/j.tins.2016.04.001
-
Expression of social behaviors of C57BL/6J versus BTBR inbred mouse strains in the visible burrow systemBehavioural Brain Research 214:443–449.https://doi.org/10.1016/j.bbr.2010.06.025
-
Physiological damage in Algerian mouse Mus spretus (Rodentia: muridae) exposed to crude oilJournal of BioScience & Biotechnology 1:.
-
Evidence that birds sleep in mid-flightNature Communications 7:12468.https://doi.org/10.1038/ncomms12468
-
Complex variation in habitat selection strategies among individuals driven by extrinsic factorsEcology and Evolution 7:1802–1822.https://doi.org/10.1002/ece3.2764
-
The value of enrichment to reintroduction successZoo Biology 32:332–341.https://doi.org/10.1002/zoo.21054
-
Effects of environmental complexity and training on brain chemistry and anatomy: a replication and extensionJournal of Comparative and Physiological Psychology 55:429.https://doi.org/10.1037/h0041137
-
Chronic second-by-second measures of L-glutamate in the central nervous system of freely moving ratsJournal of Neurochemistry 102:712–722.https://doi.org/10.1111/j.1471-4159.2007.04596.x
-
Cholesterol values in free-ranging gorillas (Gorilla gorilla gorilla and Gorilla beringei) and bornean orangutans (Pongo pygmaeus)Journal of Zoo and Wildlife Medicine 37:292–300.https://doi.org/10.1638/05-040.1
-
Nebraska Symposium on MotivationAn evolutionary and developmental theory of biphasic processes underlying approach and withdrawal, Nebraska Symposium on Motivation, University of Nebraska Press.
-
Prevention and treatment of drug addiction by environmental enrichmentProgress in Neurobiology 92:572–592.https://doi.org/10.1016/j.pneurobio.2010.08.002
-
From the lab into the wild: design and deployment methods for multi-modal tracking platformsPervasive and Mobile Computing 30:1–17.https://doi.org/10.1016/j.pmcj.2015.09.003
-
Animal WelfareEnvironmental challenge and animal agency, Animal Welfare, Wallingford, CAB International.
-
Neurobehavioral effects of environmental enrichment and drug abuse vulnerabilityPharmacology Biochemistry and Behavior 92:377–382.https://doi.org/10.1016/j.pbb.2009.01.016
-
A wearable wireless system for olfactory neural recording in freely moving rats based on Wi-Fi technologySensors and Actuators B: Chemical 213:457–464.https://doi.org/10.1016/j.snb.2015.02.111
-
A wireless multi-channel neural amplifier for freely moving animalsNature Neuroscience 14:263–269.https://doi.org/10.1038/nn.2730
-
Hearing in laboratory animals: strain differences and nonauditory effects of noiseComparative Medicine 55:12–23.
-
Optogenetic investigation of neural circuits underlying brain disease in animal modelsNature Reviews Neuroscience 13:251–266.https://doi.org/10.1038/nrn3171
-
The longevity of Caenorhabditis elegans in soilBiology Letters 1:247–249.https://doi.org/10.1098/rsbl.2004.0278
-
Behavioral phenotyping enhanced--beyond (environmental) standardizationGenes, Brain and Behavior 1:3–8.https://doi.org/10.1046/j.1601-1848.2001.00006.x
-
Assessment of positive welfare: a reviewThe Veterinary Journal 175:293–300.https://doi.org/10.1016/j.tvjl.2007.05.009
Decision letter
-
Sarah ShailesReviewing Editor; eLife, United Kingdom
In the interests of transparency, eLife includes the editorial decision letter and accompanying author responses. A lightly edited version of the letter sent to the authors after peer review is shown, indicating the most substantive concerns; minor comments are not usually included.
Thank you for submitting your article "Unbridle Biomedical Research from the Laboratory Cage" to eLife for consideration as a Feature Article. Your article has been reviewed by 3 peer reviewers, and the evaluation has been overseen by a Reviewing Editor and Stuart King as the Senior Editor.
The reviewers have discussed the reviews with one another and I have drafted this decision to help you prepare a revised submission.
The following individuals involved in review of your submission have agreed to reveal their identity: Francoise Wemelsfelder and John Gluck.
Summary:
In this feature article, Garet Lahvis brings to our attention an important issue regarding biomedical research in animals. He presents a clear and startling array of evidence, carefully referenced, that animals kept in laboratory cages do not behave normally, and that free-ranging (wild) animals would be more representative models to work with. Lahvis proposes nothing less than a revolution of scientific thought, made possible by technological advances, where animal subjects are monitored and measured under conditions not of maximum control by humans, but of maximum feasible freedom and control by the animals themselves.
The article is timely, coming as it does in the context of attempts to understand the failure of many preclinical animal studies to translate into humans and the so-called reproducibility crisis. It also details what is missing in the experiences of captive lab animals that contributes to these failures and distorts attempts to improve captive animal welfare.
Essential revisions:
1) Please delete the first paragraph of the section entitled "Responding to a Responsive Environment", or rephrase to make the main points clearer. Additional comments from reviewer #1 "Yes, enrichment should be to scale with cognitive ability. Adding a tube to a mouse cage is not to scale with adding a tube to a monkey cage. Adding a tree to a monkey enclosure, for example, would be more akin to adding a tube to a mouse cage, allowing the animal to climb it, perch in it, or sit in its shade, each experience affording the monkey a unique 360-degree visual experience. Also, visual experience is perhaps not the best example for a mouse, given its poor eyesight; 'enrichment' affording unique thigmotaxic, olfactory or auditory experience would be more appropriate."
2) Please add the following reference to the section entitled "Not just Neurobiology": Martin et al., 2010. "Control" laboratory rodents are metabolically morbid: Why it matters. PNAS 107(14): 6127-6133.
3) In the section “Research (on) animal well-being”, please broaden the discussion to include other references. It may also be helpful to also (briefly) mention and discuss the recent revival of interest in, and acceptance of, the notions of animal sentience and positive welfare. See for example reviews by Boissy et al. (2007) and Yeates and Main (2008). It seems these widely accepted developments support the author's call for greater recognition of the nature of animal lives.
4) Telemetry and Solutions: This section is the culmination of the article's argument and should be expanded.
How could traditional biomedical research (e.g. modulating gene expression) be conducted in the field using these technologies?
How would using these technologies affect the wild animals involved (e.g. sensor implantation, experimental manipulations)?
Are alternatives to uses of animals in research (e.g. tissues on a chip) likely to be useful in overcoming the validity and reliability issues intrinsic to captive animal use?
At the very least, some references where some of these technologies are described should be provided so that readers can seek further information. This section should also include some discussion of experiments that would be difficult/impossible to do in wild animals due to ethical and biological concerns (e.g. deliberately exposing the animals to a disease to trial a new drug candidate).
5) Please clarify the sentence "A benefit of using standard laboratory mice and rats is that they have evolved to be commensal with humans and this commensalism has collapsed their home range". What is meant by standard, evolved (over what time frame?), and how does that relate to their home range being 280,000 fold smaller in a laboratory (is that before or after the collapse)?
6) Is the article arguing that caged animals should no longer be studied, or that they could be if we expanded our notions of good welfare to include negative experiences? Please clarify your position from the beginning of the article and make necessary changes in the manuscript to keep your position on this central issue clear. Note that many animal welfare scientists do suggest that good welfare includes exposing animals to manageable threats, i.e. challenges that they can overcome. This gives animals agency and an opportunity to exert control over the environment; this ties into issues described in the section “Research animal well-being”.
https://doi.org/10.7554/eLife.27438.001Author response
Essential revisions:
1) Please delete the first paragraph of the section entitled "Responding to a Responsive Environment", or rephrase to make the main points clearer. Additional comments from reviewer #1 "Yes, enrichment should be to scale with cognitive ability. Adding a tube to a mouse cage is not to scale with adding a tube to a monkey cage. Adding a tree to a monkey enclosure, for example, would be more akin to adding a tube to a mouse cage, allowing the animal to climb it, perch in it, or sit in its shade, each experience affording the monkey a unique 360-degree visual experience. Also, visual experience is perhaps not the best example for a mouse, given its poor eyesight; 'enrichment' affording unique thigmotaxic, olfactory or auditory experience would be more appropriate."
This paragraph has been deleted.
2) Please add the following reference to the section entitled "Not just Neurobiology": Martin et al., 2010. "Control" laboratory rodents are metabolically morbid: Why it matters. PNAS 107(14): 6127-6133.
This reference has been added. See subsection “Not just neurobiology”, first paragraph.
3) In the section “Research (on) animal well-being”, please broaden the discussion to include other references. It may also be helpful to also (briefly) mention and discuss the recent revival of interest in, and acceptance of, the notions of animal sentience and positive welfare. See for example reviews by Boissy et al. (2007) and Yeates and Main (2008). It seems these widely accepted developments support the author's call for greater recognition of the nature of animal lives.
I added the suggested references and expanded the discussion to include a more thorough description of the kinds of conditioning experiments that I believe give us the greatest insight to non-human subject experience. I also incorporated ideas from a few philosophers. Scientists tend to trust inferences about private subjective experiences based on human language over animal behavior, a point raised by Marilyn Dawkins. I added an argument by the philosopher Bertrand Russell, who reminds us why we should not trust language for making inferences regarding human subjective states. I also referenced a book of thoughtful essays on the ethics of captivity edited by Lori Gruen. See subsection “Research into animal wellbeing”, fourth paragraph.
4) Telemetry and Solutions: This section is the culmination of the article's argument and should be expanded.
How could traditional biomedical research (e.g. modulating gene expression) be conducted in the field using these technologies?
This section is now expanded to include more technical details. See subsection “What can we do”.
How would using these technologies affect the wild animals involved (e.g. sensor implantation, experimental manipulations)?
The essay now mentions that sensor implantation and experimental manipulations often require surgery, sedatives, and anesthetics. However, the harm of these procedures is diminishing See subsection “What can we do”.
Are alternatives to uses of animals in research (e.g. tissues on a chip) likely to be useful in overcoming the validity and reliability issues intrinsic to captive animal use?
The essay now addresses the value and limitations of tissues-on-a-chip. I was surprised to learn how advanced these in vitro technologies have become. Also mentioned is that in vitro studies have their limitations. We cannot, for example, learn how a particular change in the biology of a brain affects a certain behavior without an intact organism. See subsection “What can we do”.
At the very least, some references where some of these technologies are described should be provided so that readers can seek further information. This section should also include some discussion of experiments that would be difficult/impossible to do in wild animals due to ethical and biological concerns (e.g. deliberately exposing the animals to a disease to trial a new drug candidate).
Studies that entail manipulations causing illness to animal subjects would require high levels of monitoring and the capacity for rapid retrieval. These studies would be better suited for captivity within naturalistic environments. Captivity would also be necessary for gene-targeted animals that may pose a threat to the environment. See subsection “What can we do”.
5) Please clarify the sentence "A benefit of using standard laboratory mice and rats is that they have evolved to be commensal with humans and this commensalism has collapsed their home range". What is meant by standard, evolved (over what time frame?), and how does that relate to their home range being 280,000 fold smaller in a laboratory (is that before or after the collapse)?
To clarify the sentence regarding commensalism, the essay now more clearly states that mice and rats can live in close proximity to humans at higher population densities and that the comparison shown in the figure was based upon estimations of the natural home ranges of mice and rats. See subsection “What can we do”, sixth paragraph.
6) Is the article arguing that caged animals should no longer be studied, or that they could be if we expanded our notions of good welfare to include negative experiences? Please clarify your position from the beginning of the article and make necessary changes in the manuscript to keep your position on this central issue clear. Note that many animal welfare scientists do suggest that good welfare includes exposing animals to manageable threats, i.e. challenges that they can overcome. This gives animals agency and an opportunity to exert control over the environment; this ties into issues described in the section “Research animal well-being”.
My position is now clarified throughout. In consideration of their wellbeing and more relevant findings in biomedical research, animal models should be either studied in the wild or studied as captive subject under naturalistic conditions. See main text, first paragraph, subsection “Research into animal wellbeing”, last paragraph, and subsection “Conclusions, first paragraph).
https://doi.org/10.7554/eLife.27438.002Article and author information
Author details
Funding
No external funding was received for this work.
Publication history
- Received:
- Accepted:
- Accepted Manuscript published:
- Version of Record published:
- Version of Record updated:
Copyright
© 2017, Lahvis et al.
This article is distributed under the terms of the Creative Commons Attribution License, which permits unrestricted use and redistribution provided that the original author and source are credited.
Metrics
-
- 4,046
- views
-
- 330
- downloads
-
- 33
- citations
Views, downloads and citations are aggregated across all versions of this paper published by eLife.
Download links
Downloads (link to download the article as PDF)
Open citations (links to open the citations from this article in various online reference manager services)
Cite this article (links to download the citations from this article in formats compatible with various reference manager tools)
Further reading
-
- Ecology
For the first time in any animal, we show that nocturnal bull ants use the exceedingly dim polarisation pattern produced by the moon for overnight navigation. The sun or moon can provide directional information via their position; however, they can often be obstructed by clouds, canopy, or the horizon. Despite being hidden, these bodies can still provide compass information through the polarised light pattern they produce/reflect. Sunlight produces polarised light patterns across the overhead sky as it enters the atmosphere, and solar polarised light is a well-known compass cue for navigating animals. Moonlight produces an analogous pattern, albeit a million times dimmer than sunlight. Here, we show evidence that polarised moonlight forms part of the celestial compass of navigating nocturnal ants. Nocturnal bull ants leave their nest at twilight and rely heavily on the overhead solar polarisation pattern to navigate. Yet many foragers return home overnight when the sun cannot guide them. We demonstrate that these bull ants use polarised moonlight to navigate home during the night, by rotating the overhead polarisation pattern above homing ants, who alter their headings in response. Furthermore, these ants can detect this cue throughout the lunar month, even under crescent moons, when polarised light levels are at their lowest. Finally, we show the long-term incorporation of this moonlight pattern into the ants’ path integration system throughout the night for homing, as polarised sunlight is incorporated throughout the day.
-
- Ecology
Climatic warming can shift community composition driven by the colonization-extinction dynamics of species with different thermal preferences; but simultaneously, habitat fragmentation can mediate species’ responses to warming. As this potential interactive effect has proven difficult to test empirically, we collected data on birds over 10 years of climate warming in a reservoir subtropical island system that was formed 65 years ago. We investigated how the mechanisms underlying climate-driven directional change in community composition were mediated by habitat fragmentation. We found thermophilization driven by increasing warm-adapted species and decreasing cold-adapted species in terms of trends in colonization rate, extinction rate, occupancy rate and population size. Critically, colonization rates of warm-adapted species increased faster temporally on smaller or less isolated islands; cold-adapted species generally were lost more quickly temporally on closer islands. This provides support for dispersal limitation and microclimate buffering as primary proxies by which habitat fragmentation mediates species range shift. Overall, this study advances our understanding of biodiversity responses to interacting global change drivers.