A neural circuit for gamma-band coherence across the retinotopic map in mouse visual cortex
Figures
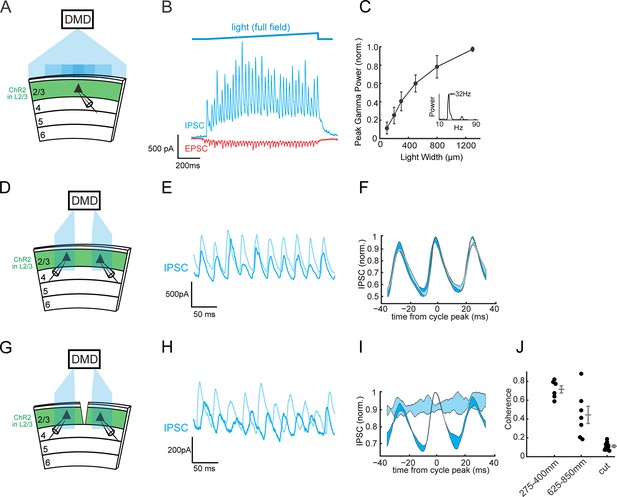
Horizontal circuits recruit local SOM interneurons to synchronize distant gamma generators.
(A) Experimental schematic: A ChR2-negative Pyramidal cell is recorded in L2/3 of V1 while other ChR2-expressing L2/3 neurons are photo-stimulated with different sizes of blue light stimuli using a digital-micromirror-device (DMD). (B) Top: Time course of the light stimulus intensity (final intensity 1.1 mW/mm2, see Materials and methods). Bottom: Example traces of voltage-clamped excitatory postsynaptic current (EPSC, red) and inhibitory postsynaptic current (IPSC, blue) during photo-induced gamma rhythms in V1. (C) Plot of peak gamma power versus the width of the photo-stimulus on L2/3 (n = 8, p<10−4, Kruskal-Wallis ANOVA). Errorbars are s.e.m. (D) Experimental schematic: two ChR2-negative L2/3 pyramidal cells are simultaneously recorded while nearby ChR2-expressing L2/3 PCs are focally activated with separate blue light patches using a digital micro-mirror device (DMD). The distance between the blue light patches ranged from 275 to 850 μm (see Figure 1—figure supplement 1B). (E) Example traces of the voltage-clamped IPSCs from a pair of simultaneously recorded L2/3 PCs during photo-induction of two separate gamma oscillations. (F) Oscillation-triggered average of the IPSCs recorded in the pair in B) (triggered off the oscillations in one of the two cells, labeled in dark blue). Shading represents one standard deviation. (G–I) As in (D–F) but following a transection of L2/3 between the two recorded L2/3 PCs in transfected slices. (J) Scatter plot of the peak coherence of the oscillations in the two recorded neurons between the cut and the two intact conditions. Mean peak coherence with 275–400 μm separation (close): 0.72 ± 0.04, n = 6 pairs; mean peak coherence at 625–850 μm separation (far): 0.44 ± 0.09, n = 7 pairs; mean peak coherence at 275–400 μm with L2/3 cut (cut): 0.11 ± 0.01, n = 11 pairs; p<10−3, Wilcoxon rank sum test between close and cut conditions; p<10−3, Wilcoxon rank sum test between far and cut conditions. Errorbars are s.e.m.
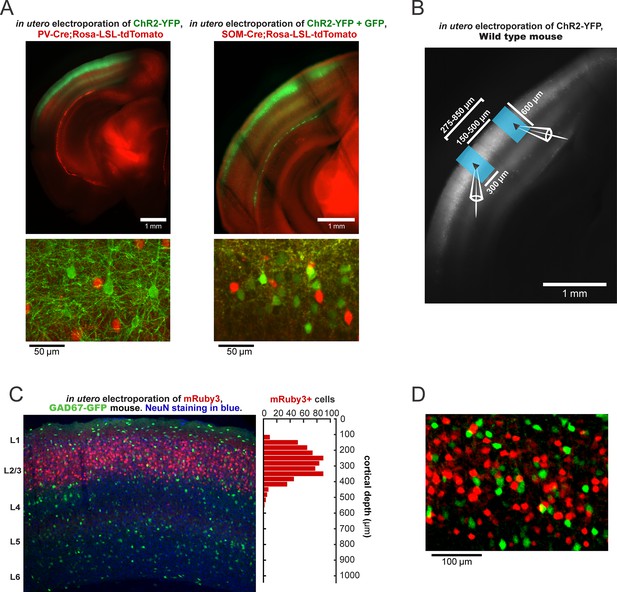
In utero electroporation of ChR2-YFP into SOM-Cre, PV-Cre, and wild-type mice and spatial restriction of ChR2 expression to L2/3.
(A) Top left: Widefield epifluorescent example image of a 400-μm-thick acute slice from a PV-Cre;LSL-tdTomato mouse in utero electroporated with ChR2-YFP at E15.5. Bottom left: Close up confocal image of fixed a 40-μm-thick section. Top Right: Widefield epifluorescent example image of a 400 μm thick acute slice from a SOM-Cre;LSL-tdTomato mouse in utero electroporated with ChR2-YFP and GFP at E15.5. Bottom Right: Close up confocal image from the same slice. (B) A low-magnification image of a slice from a wild-type mouse electroporated with ChR2-YFP with overlays representative of the light stimulus delivered in the experiments seen in Figure 1D–J. (C) Left: Confocal image from V1 of a GAD67-GFP mouse that has been in utero electroporated with the red fluorescent protein mRuby3 (red). The slice was subsequently stained for NeuN (blue). Right: histogram of the counts of mRuby3 +cells as a function of depth. (D) Zoomed in image from C) showing non-overlapping populations. 2/1519 GFP neurons were co-labeled for mRuby3 and GFP in all layers.
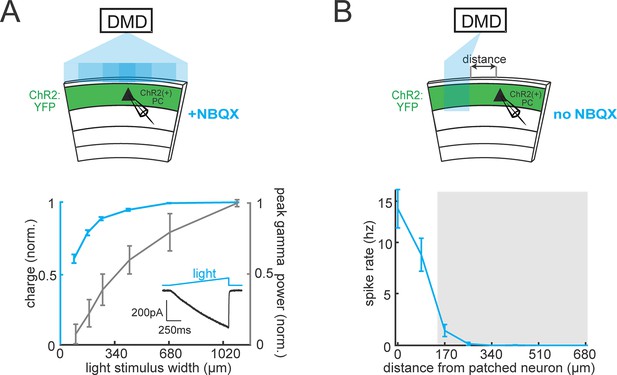
Spatial resolution controls for DMD-based optogenetic activation of PCs.
(A) Top: experimental schematic. A single ChR2-expressing neuron is patched in L2/3 while a patch of blue light of increasing width is projected onto the slice. The slice is perfused with 10 μM NBQX (2,3-dihydroxy-6-nitro-7-sulfamoyl-benzo[f]quinoxaline-2,3-dione) to block synaptic excitatory currents. Bottom: Plot of the average normalized optically induced charge in ChR2-expressing PCs as a function of light stimulus width (blue). Data on the size-dependent gamma power of inhibitory currents (grey) is replotted from Figure 1C for comparison. Inset: Example of a light-induced photo-current in a ChR2-expressing pyramidal cell to a ramp of blue light. (B) Top: experiment schematic. A single ChR2-expressing neuron is patched in L2/3 while a 150 μm slit of blue light is moved across the slice in 100 μm increments. Bottom: Plot of the average normalized optically induced spike rate in ChR2-expressing neurons as a function of the distance of the light stimulus from the patched neuron. The shaded region indicates the range of distances used in Figure 1 between the two photo-stimulation zones (n = 4 cells from two animals). All errorbars are s.e.m.
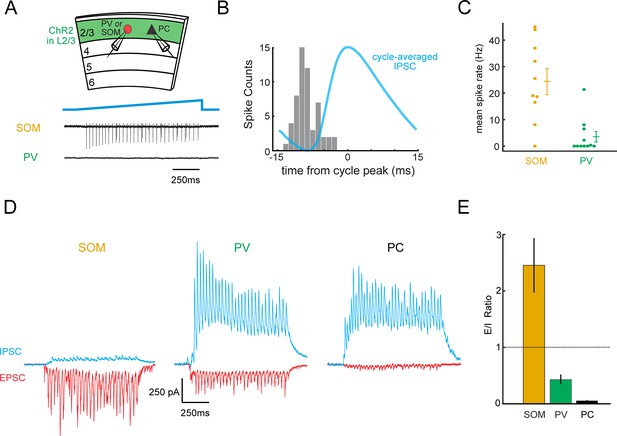
L2/3 gamma rhythms recruit SOM inhibitory neurons.
(A) Top: Schematic of experiment. Loose-patch recordings are made from PV or SOM cells, and whole-cell recordings are made from Pyramidal cells (PC) to correlate to gamma rhythms. Slices expressing ChR2 in L2/3 and tdTomato in PV or SOM cells (see Figure 1—figure supplement 1). Bottom: example loose-patch recordings from a SOM and a PV neuron during photo-induced gamma. (B) Phase histogram of the spikes recorded in a SOM neuron during photo-induced gamma overlaid with the simultaneously recorded cycle-averaged IPSC. (C) Plot of the mean firing rate of PV and SOM neurons during photo-induced gamma (PV: n = 11 cells, SOM n = 10 cells, p<0.01, Wilcoxon rank sum test). Baseline spike rate was zero. (D) Representative traces of synaptic excitation (red) and inhibition (blue) from a SOM, PV, and PC. Baseline synaptic input is near-zero for both EPSCs and IPSCs. (E) Plot of the average E/I ratio in SOMs, PVs, and PCs during gamma activity in brain slices (n = 12 SOM cells, mean E/I ratio: 2.5 ± 0.5; 8 PV cells, mean E/I ratio: 0.43 ± 0.09; 10 pyramidal cells, mean E/I ratio: 0.054 ± 0.010; p<10−3, Wilcoxon rank sum test between SOM and PV; p<10−5, Kruskal-Wallis ANOVA). Error bars are s.e.m.
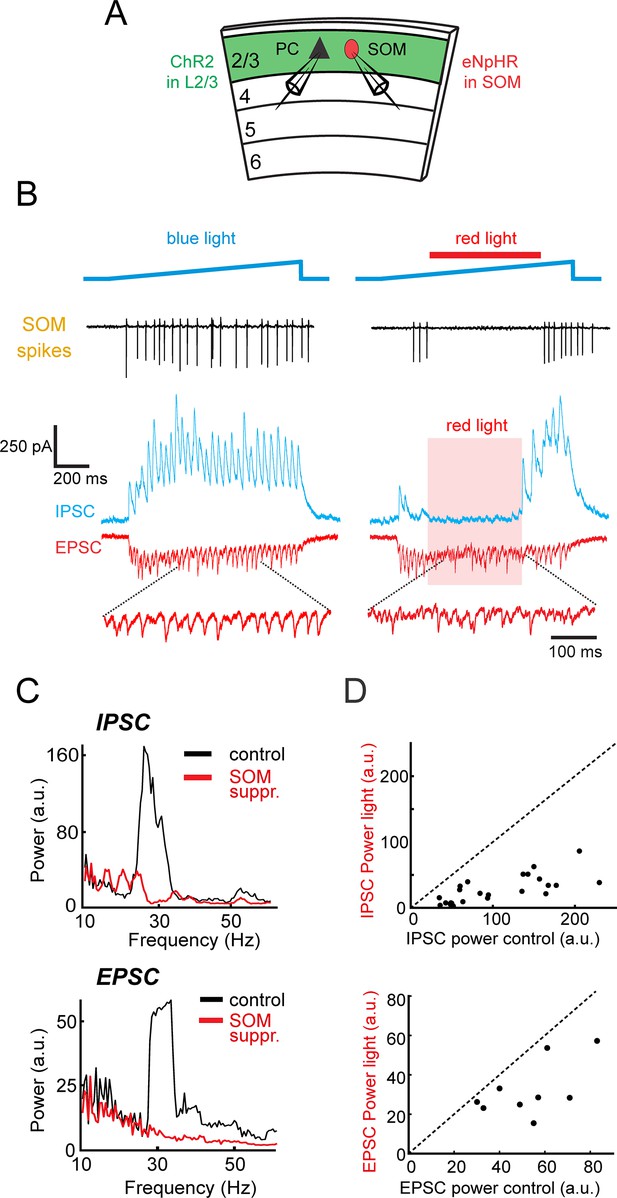
L2/3 gamma rhythms depend on SOM neuron activity.
(A) Schematic of experiment. Whole-cell recordings are made from L2/3 Pyramidal cells (PC) in slices containing ChR2 in L2/3 and eNpHR3.0 in SOM cells. In some experiments a SOM cell was simultaneously recorded. (B) Left: loose-patch recording of spiking in an example eNphR3.0 expressing-SOM cell (black, top trace) and the photo-induced excitatory (red) and inhibitory (blue) currents in a representative PC. Right: Same cells, but with the addition of red light (red bar) to suppress SOM cells. Bottom: expanded excitatory currents. (C) Power spectra of the recorded inhibition (top) and excitation (bottom) in the PC in B) under control conditions (black) and during photo-suppression of SOM cells (red). (D) Scatter plots of the peak gamma power of inhibition (top) and excitation (bottom) with and without photo-suppression of SOM cells (Inhibition: n = 24, p<10−5, Wilcoxon signed rank test) (Excitation: n = 9, p<0.01, Wilcoxon signed rank test).
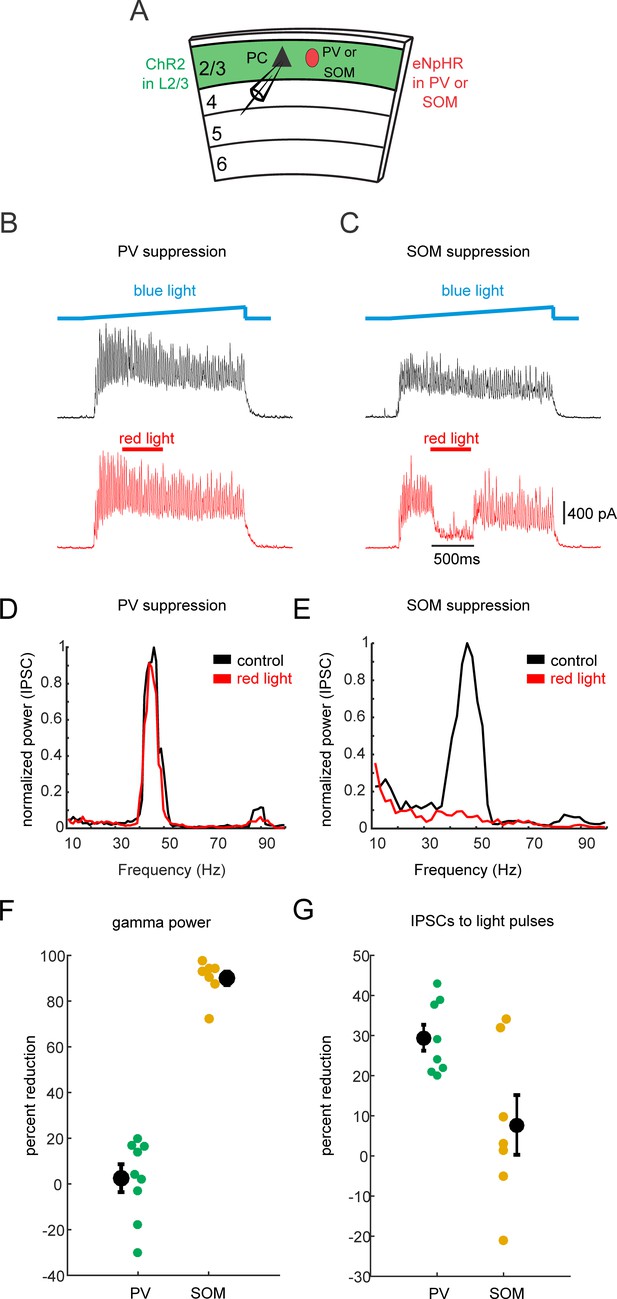
PV-neuron suppression does not significantly affect gamma oscillations.
(A) Schematic of experiment. Whole-cell recordings are made from L2/3 Pyramidal cells (PC) in slices containing ChR2 in L2/3 and eNpHR3.0 in PV or SOM cells. (B) Example IPSC traces from a L2/3 PC during photo-induced gamma oscillations. Black trace: blue light ramp only. Red: with the addition of 500 ms of red light. ChR2 is expressed in L2/3 PCs, and eNpHR3.0 is expressed in PV neurons. (C) Same as in A), but eNpHR3.0 is expressed in SOM cells. (D) Normalized power spectra of example IPSC traces in A) and B) under control conditions (black) and when red light is used to suppress PV cells. (E) As in C), but eNpHR3.0 is expressed in SOM cells. F) Scatter plot of the percent reduction in the peak gamma power in IPSCs when PV cells (green) or SOM cells (yellow) are optogenetically suppressed. (G) Scatter plot of the percent reduction in the peak amplitude of an IPSC measured in response to a brief pulse of blue light to photo-stimulate L2/3 PCs when either PV cells (green) or SOM cells (yellow) are optogenetically suppressed. All error bars are s.e.m.
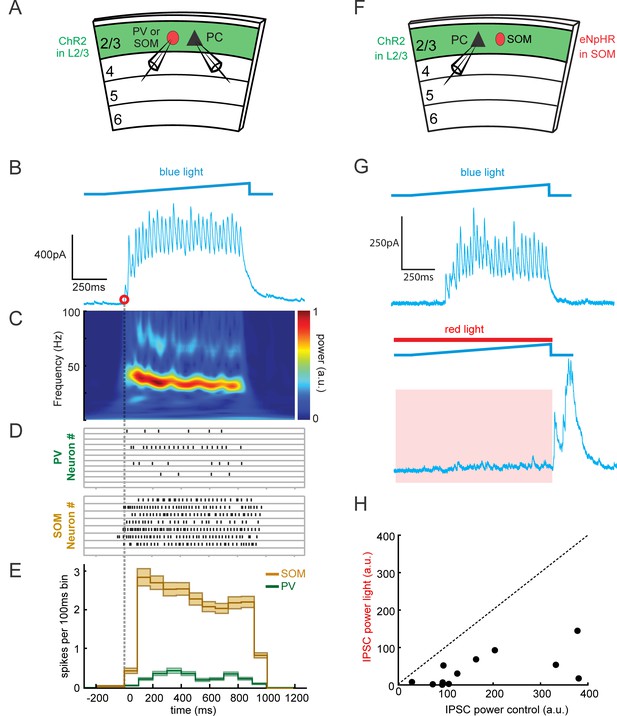
SOM neurons mediate both initiation and maintenance of gamma oscillations.
(A) Schematic of the experiment (as in Figure 2). Loose-patch recordings are made from PV or SOM cells, and whole-cell recordings are made from Pyramidal cells (PC) during photo-induced gamma rhythms in slices expressing ChR2 in L2/3 and tdTomato in PV or SOM cells (see Figure 1—figure supplement 1). (B) Example traces of light-evoked inhibitory currents. The onset time of the IPSCs was defined as the initial rise in IPSC amplitude indicated by the red circle. (C) Wavelet spectrogram of the example trace in B). (D) Raster plots of spike times relative to IPSC onset time recorded in the trial from PV (top) and SOM (bottom) neurons. (E) Peri-onset time histogram of spike times in D), in 100 ms bins. (B-E shared time axis relative to onset of IPSCs). (F) Schematic of the experiment (as in Figure 3). Whole-cell recordings are made from L2/3 Pyramidal cells (PC) in slices containing ChR2 in L2/3 and eNpHR3.0 in SOM cells. (G) Example traces of inhibitory oscillations when blue light is delivered alone (top), and when red light is delivered prior to the onset of the blue light (bottom). (H) Scatter plots of the peak gamma power of inhibition with and without photo-suppression of SOM cells (n = 12 Pyramidal cells, p<10−3, Wilcoxon signed rank test).
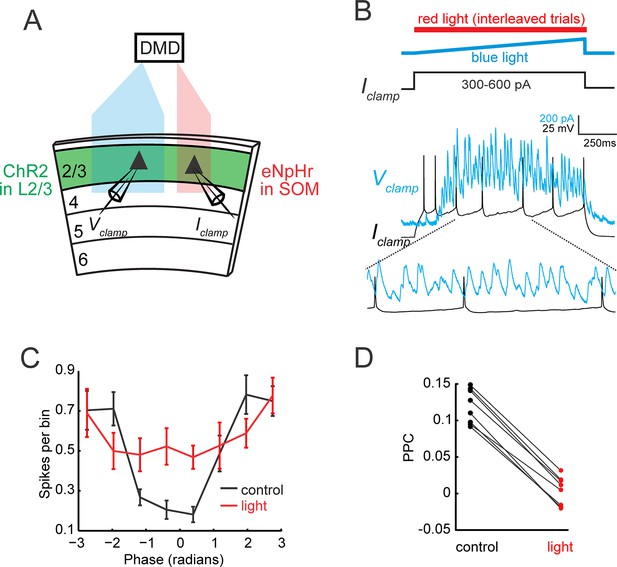
Gamma band synchronization across the retinotopic axis depends on local SOM neuron activity.
(A) Schematic of experiment: One area of the slice is photo-stimulated with blue light to generate a local gamma oscillation, while the second distal site is simultaneously illuminated with red light to suppress local SOM activity focally (see Figure 4—figure supplement 1C). (B) Example traces from a simultaneously recorded pair of L2/3 PCs. The cell in the blue light zone is recorded in voltage clamp (blue, IPSC), while the cell in the red light zone is recorded in current clamp and stimulated with a current injection to evoke action potentials (gray). Current injections ranged between 300 and 600 pA. Bottom: Expanded trace showing spikes occurring at the toughs of inhibition. (C) Population phase histogram of the spikes of the current injected L2/3 PC in the red light zone under control conditions (blue light only) and during photo-suppression of local SOM cells relative to the phase of the gamma oscillation recorded in the second PC in the blue light zone. Error bars are s.e.m. (D) Scatter plot of the pairwise phase consistency of the L2/3 PC recorded in the red light zone relative to the oscillation recorded in the L2/3 PC in the blue light zone (p<10−7, n = 8 pairs, Wilcoxon signed-rank test).
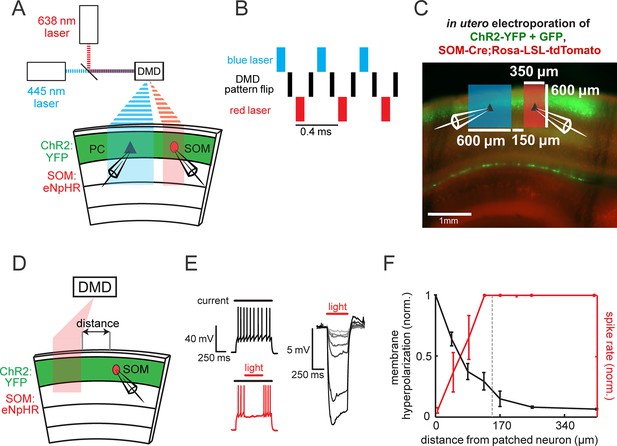
Spatial resolution controls for DMD-based optogenetic suppression of SOM neurons.
(A) Schematic of the optical configuration for the temporal multiplexing experiment of red and blue light in Figure 4. (B) Pulse sequences used for temporally multiplexing blue and red light patterns on the DMD for simultaneous but spatially separated optogenetic activation of ChR2 and eNpHR3.0. (C) A low-magnification image of a slice from a SOM-tdTomato mouse electroporated with ChR2-YFP with overlays representative of the light stimulus delivered in the experiments seen in Figure 4. (D) Schematic of experiment. A single eNpHR3.0-expressing SOM neuron is patched in L2/3 while a 150 μm slit of red light is incrementally moved across the slice. (E) Left: Example traces of a SOM cell given current injection to spike (top), and with red light delivered to suppress spiking (bottom). Right: Example traces of a SOM cell’s membrane potential upon red light stimulation at different distances from the cell (darkest is nearest). (F) Plot of the normalized spike rate to a current injection evoking an average spike rate of 10 Hz (red) or hyperpolarization (grey) as a function of the distance between the red light patch and recorded neurons (n = 4 cells from two animals for both conditions). The vertical dashed line indicates the distance used in Figure 4 between the photo-stimulation and photo-suppression zones. All error bars are s.e.m.
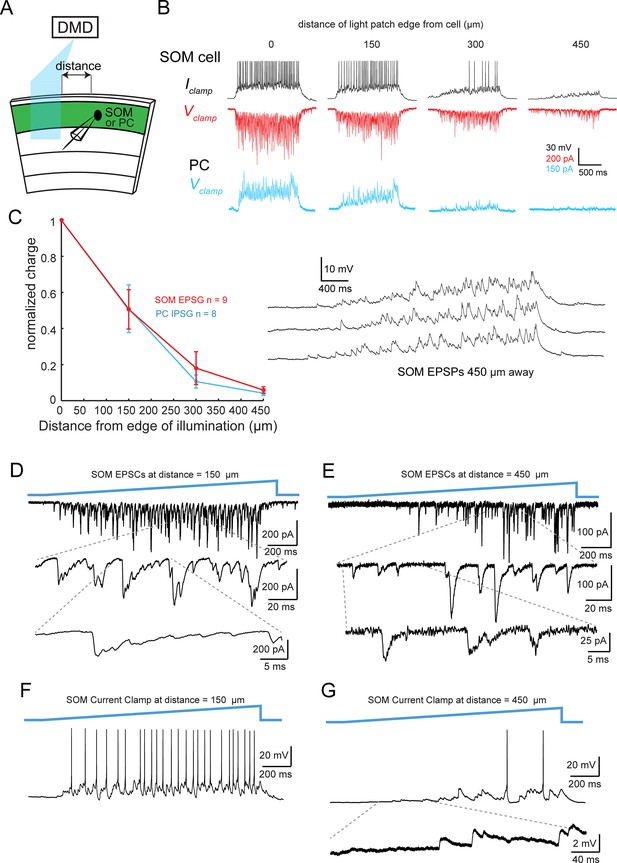
Horizontal input to SOM neurons and PCs.
(A) Experiment schematic. (B) Example traces of membrane voltage and current in SOM neurons and PCs during photo-stimulation of ChR2-expressing PCs at increasing distances. The indicated distance is from the edge of the blue light patch to the recorded neuron. Top: (black) Membrane potential recording of a representative SOM neuron. Middle (red): voltage clamp recording of EPSCs from the same SOM neuron. Bottom (blue): voltage clamp recording of light-evoked IPSCs in a PC. (C) Left: Plot of the normalized excitatory synaptic charge in SOM neurons and inhibitory synaptic charge in PCs as a function of distance between the edge of the blue light patch and the recorded neuron. Right: Three example membrane potential recordings for a SOM neuron when photo-stimulating PCs 450 μm away, showing no evidence of spikelets. (D) Top: EPSCs measured in a SOM cell near the blue light patch. Middle and bottom: expanded traces showing the shapes of synaptic conductances. (E) Same cell as D), but at 450 μm away. (F,G) As in D,E), but at 450 μm away.
Additional files
-
Transparent reporting form
- https://doi.org/10.7554/eLife.28569.012