Glutamate is required for depression but not potentiation of long-term presynaptic function
Figures
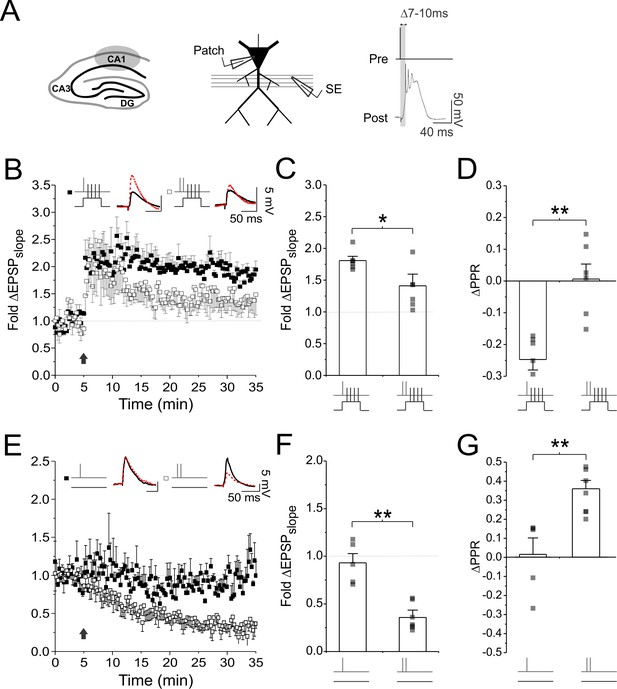
High frequency presynaptic activity inhibits LTPpre and promotes LTDpre.
(A) Experimental setup. CA1 pyramidal neurons were recorded using whole-cell patch electrodes. LTP was induced by causal pairing of presynaptic activity with postsynaptic depolarization in the form of a complex spike. (B,E) Average fold changes of EPSP slope plotted against time. Following baseline recording, 60 presynaptic stimuli were delivered at 5 Hz, either in the presence (paired stimulation) or absence (unpaired stimulation) of postsynaptic depolarization. Presynaptic stimuli were delivered either as single pulses, or high frequency presynaptic bursts consisting of two pulses delivered 5 ms apart. Sample EPSP traces at baseline (black trace) and 30 min after plasticity induction (broken red trace) are shown as an inset above each graph. Average changes in (C,F) EPSP slope and (D,G) PPR measured 25–30 min after plasticity induction. High frequency bursts generated significantly less LTPpre with paired stimulation, and more LTDpre with unpaired stimulation, than did single pulse stimulation. Error bars represent S.E.M. (n = 5–8 cells per condition). Asterisks denotes significance differences between groups (*p<0.05; **p<0.01; Mann-Whitney test).
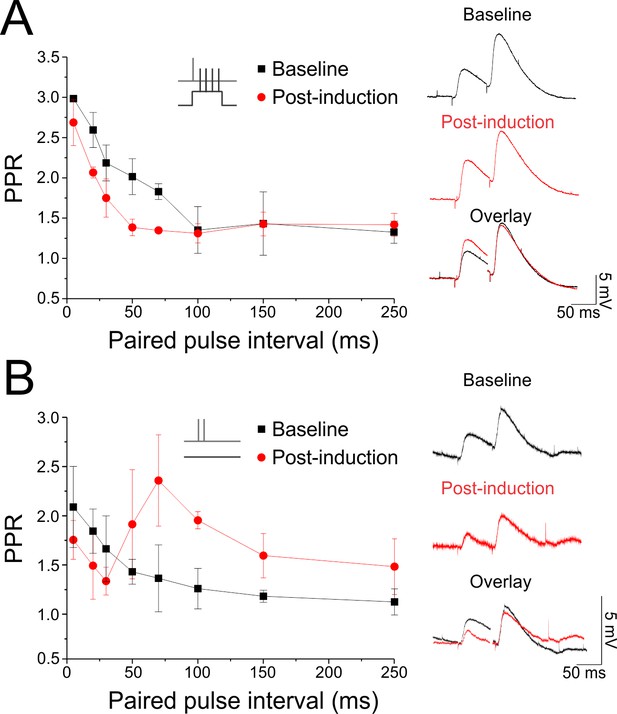
LTPpre and LTDpre induction are associated with changes in paired pulse ratio (PPR) at a range of paired pulse intervals.
Exemplar experiments of PPR changes across a range of paired pulse intervals before and 30 min after (A) LTP induction by paired stimulation (60 pairings at 5 Hz) or (B) LTD induction by unpaired stimulation (2 pulses at 200 Hz repeated 60 times at 5 Hz). LTP and LTD were respectively associated with an increase and decrease in PPR at a range of intervals. Raw sample traces of paired pulse stimulation at 70 ms intervals are shown to the right of each graph for baseline and post-induction periods. From the overlay of these traces it is evident that LTP and LTD preferentially affected the first of two pulses, leading to the observed changes in PPR. Data points represent the average of 2–5 stimulation trials. Error bars represent standard deviation, estimated with jackknifing. Statistical tests were not performed as data came from a single exemplar experiment (n = 1/plasticity condition).
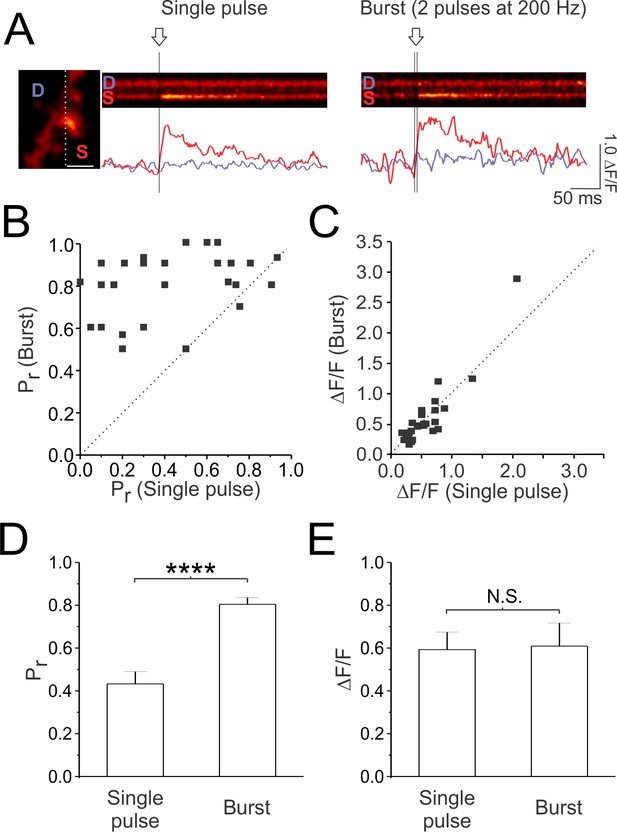
Presynaptic burst stimulation increases Pr.
Ca2+imaging was used to estimate Pr at single synapses during either single pulse or burst (2 pulses at 200 Hz) stimulation. Pr was measured as the fraction of total stimulation trials that successfully elicited a Ca2+ transient (see Materials and methods). (A) Sample line scans of a spine (S) and underlying dendrite (D) showing a successful stimulation trial using single pulse and burst stimulation (vertical lines) (scale bar: 1 µm). The change in fluorescence intensity of the Ca2+ response (ΔF/F) is quantified below for both the spine (red) and underlying dendrite (blue). Ca2+ transients during both stimulation regimes remained localized to the spine head. (B) For each synapse imaged, the Pr measured during single pulse stimulation is plotted against the Pr measured during burst stimulation. The broken diagonal line represents the expected trend if Pr is unchanged by stimulation. Burst stimulation greatly increased Pr. (C) For each synapse imaged, the average amplitude of the Ca2+ responses (ΔF/F) measured in successful trials of single pulse stimulation is plotted against that measured during successful trials of burst stimulation. The broken diagonal line represents the line of equality. Burst stimulation did not increase the amplitude of the Ca2+ response, suggesting that it did not increase the amount of glutamate that was released in a given trial, but instead only increased the probability that glutamate was released. (D) Average Pr. (E) Average Ca2+ signal (ΔF/F). Error bars represent S.E.M. (n = 26 spines per condition). Asterisks denote significant differences (****p<0.0001; Wilcoxon matched pairs signed rank test). N.S. denotes no significant difference.
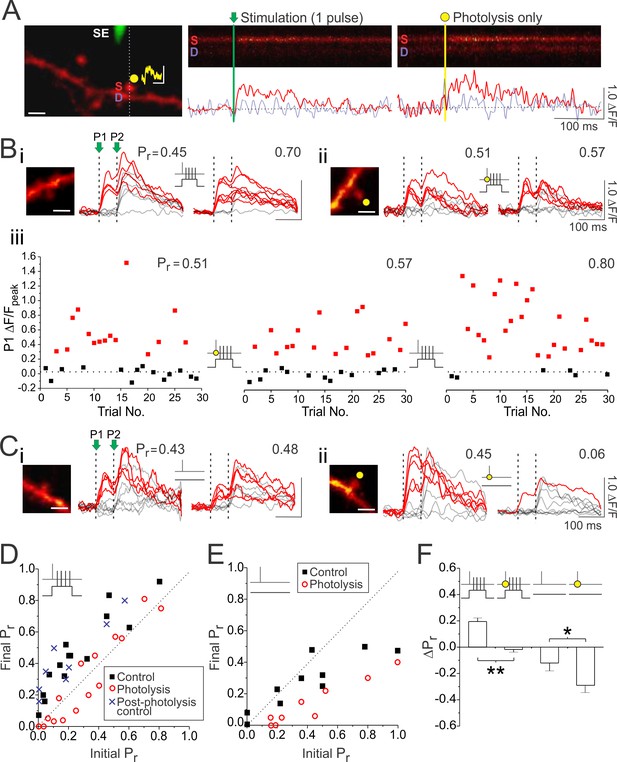
Glutamate photolysis inhibits LTPpre and promotes LTDpre.
(A) Left, an image of a CA1 neuronal dendrite loaded with Oregon Green BAPTA-1 Ca2+-sensitive dye. A stimulating electrode (SE; green) was placed close to the dendrite in order to activate spines within the vicinity (scale bar: 2 µm). A spine responsive to stimulation was located and then targeted for glutamate photolysis (yellow spot). An example of an uncaging-evoked synaptic potential is shown above the imaged spine (scale bar: 1 mV by 100 ms). During stimulation and photolysis, evoked Ca2+ transients were rapidly imaged by restricting laser scanning to a line across the spine head and underlying dendrite (broken line). Right, samples of these Ca2+ transients in both the spine (labelled S) and dendritic (labelled D) are shown. Below each line scan image are traces quantifying the fluorescence change (ΔF/F) for the spine (red trace; raw) and dendrite (purple trace; raw). Photolysis laser power was adjusted to elicit spine Ca2+ transients comparable to that induced by single presynaptic stimuli. (Bi and Bii) Samples of 10 superimposed Ca2+ traces evoked in imaged spines (white scale bar: 2 µm) by paired pulse stimulation (P1 and P2 were delivered 70 ms apart and are represented by vertical broken lines); red traces depict successful release events to the first (P1) of the two pulses. Ca2+ traces are shown during baseline and 25–30 min following paired stimulation, delivered in either the (Bi) absence or (Bii) presence of glutamate photolysis (yellow circle). Pr was calculated as the proportion of total stimulation trials in which the first pulse (P1) resulted in a successful release event. (Biii). For the experiment in (Bii), the peak ΔF/F in the spine head is plotted for the first pulse (P1) across 30 stimulation trials given at baseline, 30 min following paired stimulation with photolysis, and 30 min following a subsequent round of paired stimulation in the absence of photolysis. Red squares denote fluorescent increases above noise. Increases in Pr could only be induced by paired stimulation delivered in the absence of glutamate photolysis. (Ci and Cii) As in (B) except for unpaired stimulation (60 pulses at 5 Hz), which alone had a negligible effect on low Pr synapses (Pr <0.5). Glutamate photolysis promoted greater decreases in Pr than unpaired stimulation alone. (D,E) The final Pr measured 25–30 min following stimulation is plotted against the initial Pr measured at baseline for each imaged synapse. The broken diagonal line represents the expected trend if Pr is unchanged by stimulation. The post-photolysis control group consisted of 8 synapses from the photolysis group that underwent a second round of paired stimulation, but in the absence of glutamate photolysis (see Biii for example). (F) Average changes in Pr. Error bars represent S.E.M. (n = 9–14 spines per condition). Asterisks denotes significance differences between groups (*p<0.05; **p<0.01; Mann-Whitney test).
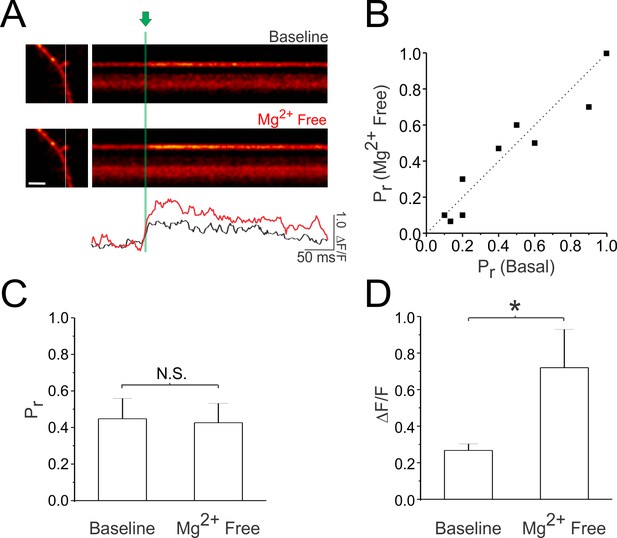
Augmenting spine Ca2+signalling does not alter Pr estimates.
Ca2+ imaging was used to estimate Pr at single synapses under baseline conditions (2 mM Mg2+) and following baseline removal of Mg2+ in order to maximize the Ca2+ signal in the spine. (A) Sample line scan of a spine and underlying dendrite stimulated with a presynaptic pulse (green vertical line) under baseline conditions and after removal of Mg2+ from the bath (scale bar: 2 µm). The change in fluorescence intensity of the Ca2+ response (ΔF/F) is quantified below (black trace obtained at baseline; red trace obtained following removal of Mg2+). Removal of Mg2+ increases the magnitude of the Ca2+ response. (B) For each synapse imaged, the Pr measured under basal conditions is plotted against the Pr measured following removal of Mg2+. The broken diagonal line represents the expected trend if Pr is unchanged across conditions. (C) Average Pr. (D) Average change in Ca2+ signal (ΔF/F). Removal of Mg2+ more than doubles the Ca2+ signal on average, but does not change Pr estimates. Error bars represent S.E.M. (n = 9 spines per condition). Asterisks denote significant differences (*p<0.05; Wilcoxon matched pairs signed rank test). N.S. denotes no significant difference.

Induction of LTPpre in glutamate receptor blockade.
(A) Experimental setup. EPSPs were recorded from two independent Schaffer-collateral pathways. LTP was induced in a full glutamate receptor blockade (100 µM D-AP5, 10 µM NBQX, 500 µM R,S-MCPG, and 10 µM LY341495) by paired stimulation. Only one pathway (black box) was active during paired stimulation, the other pathway served as a control (white boxes). (B) Average fold change in the EPSP slope plotted against time for both the control and paired pathways. Sample EPSP traces shown are averages of 10 traces from the paired (solid line) and control (broken red line) pathway taken at four time points (1-4) from a single experiment (scale bar: 4.0 mV by 40 ms for paired pathway EPSP, 4. 9 mV by 40 ms for control pathway EPSP). Stimulation artifacts have been removed for clarity. (C) Average fold change in EPSP slope plotted for control experiments in which glutamate receptor antagonists were applied alone, in the absence of paired stimulation (drugs only group). Note that drug washout was incomplete. Group data and averages plotted for (D) fold changes in EPSP slope and (E) changes in PPR across experiments as measured 30 min following paired stimulation and drug washout. EPSP slope was higher in the paired pathway than in the control pathway, and was associated with a decrease in PPR, suggesting that LTP had been induced in the paired pathway, and had a presynaptic locus of expression. Error bars represent S.E.M (n = 5–7 cells per condition). Asterisks denotes significance differences between groups (**p<0.01; *p<0.05; Wilcoxon matched pairs signed rank test or Kruskal-Wallis with post-hoc Dunn’s test). N.S. denotes no significant differences between groups.
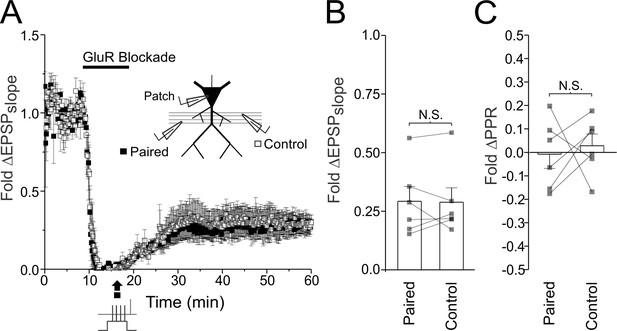
Anti-causal pairing of pre- and postsynaptic activity fails to induce LTPpre in full glutamate receptor blockade.
EPSPs were recorded from two independent Schaffer-collateral pathways. LTP was induced in full glutamate receptor blockade (100 µM D-AP5, 10 µM NBQX, 500 µM R,S-MCPG, and 10 µM LY341495) by anti-causal pairing of single presynaptic stimuli and postsynaptic depolarization in the form of a complex spike. Pairings were delivered 60 times at 5 Hz. Only one pathway (black box) was active during paired stimulation, the other pathway served as a control (white boxes). (A) Average fold change in the EPSP slope plotted against time for both control and paired pathways. (B) Average fold change in EPSP slope in the paired and control pathways 30 min following paired stimulation and drug washout. (C) Average change in PPR for paired and control pathways. When compared to the control pathway, anti-causal pairings failed to elicit changes in the EPSP slope. No changes in PPR were observed in any of the pathways. Error bars represent S.E.M. (n = 6 cells per condition). N.S. denotes no significant differences (Wilcoxon matched pairs signed rank test).
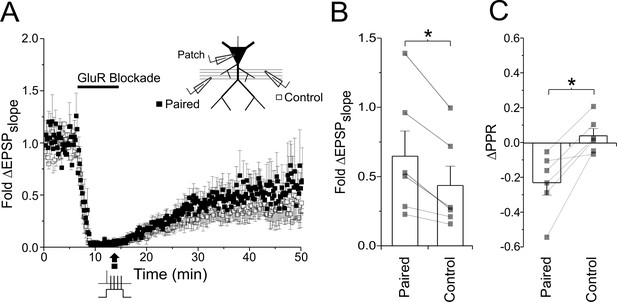
Induction of LTPpre under full glutamate receptor blockade in acute hippocampal slices.
EPSPs were recorded from two independent Schaffer-collateral pathways in acute hippocampal slices. LTP was induced in full glutamate receptor blockade (100 µM D-AP5, 10 µM NBQX, 500 µM R,S-MCPG, and 10 µM LY341495) by causal pairings of single presynaptic stimuli and postsynaptic depolarization in the form of a complex spike (60 times at 5 Hz). Only one pathway (black box) was active during paired stimulation, the other pathway served as a control (white boxes). (A) Average fold change in the EPSP slope plotted against time for both control and paired pathways. (B) Average fold change in EPSP slope in the paired and control pathways 30 min following paired stimulation and drug washout. (C) Average change in PPR for paired and control pathways. EPSP slope was higher in the paired pathway than in the control pathway, and was associated with a decrease in PPR, suggesting that LTP had been induced in the paired pathway, and had a presynaptic locus of expression. Error bars represent S.E.M. (n = 6 cells per condition). (*p<0.05; Wilcoxon matched pairs signed rank test).
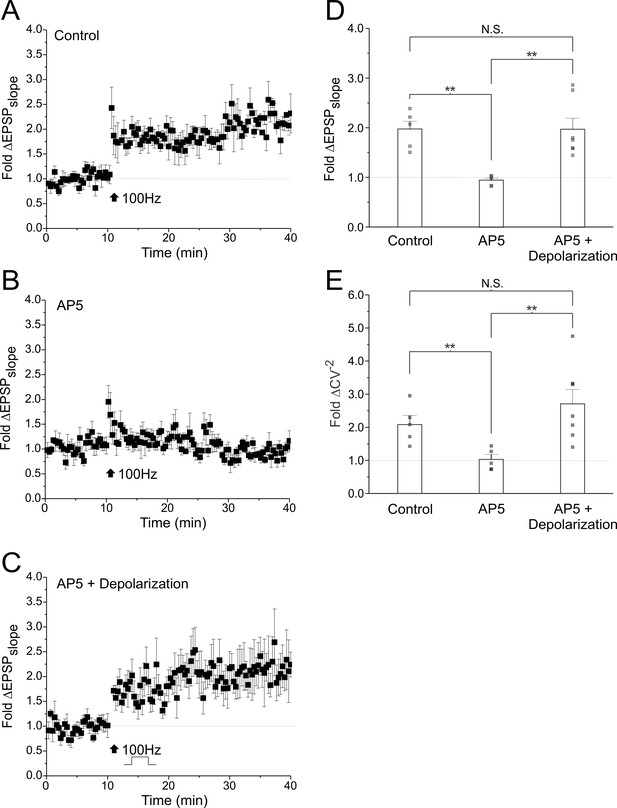
Membrane depolarization rescues LTP induction in NMDAR blockade.
EPSPs were recorded from CA1 neurons using sharp microelectrodes (80–120 MΩ). After baseline recording, tetanic stimulation (black arrow; 3 trains of 20 pulses at 100 Hz delivered 10 s apart) was delivered under (A) control conditions, (B) in the presence of AP5 (100 µM), and (C) in the presence of AP5 and membrane depolarization but in conjunction with of a 10–20 mV membrane depolarization from resting potential (−60 mV) induced by somatic current injection. Membrane depolarization during tetanic stimulation was able to rescue the induction of LTP in AP5. (D) Average fold change in the EPSP slope. (E) Long baseline recordings (i.e. 10 min in duration), enabled by the use of sharp microelectrodes, allowed for calculation of the co-efficient of variation (CV). Average fold change in CV−2 are shown (Faber and Korn, 1991). Values over one indicate that LTP induction was associated with a presynaptic component of expression. PPR was not measured in these experiments. Error bars represent S.E.M. (n = 5–7 cells per condition). Asterisks denotes significance differences between groups (**p<0.01; Kruskal-Wallis with post-hoc Dunn’s test). N.S. denotes no significant differences between groups.
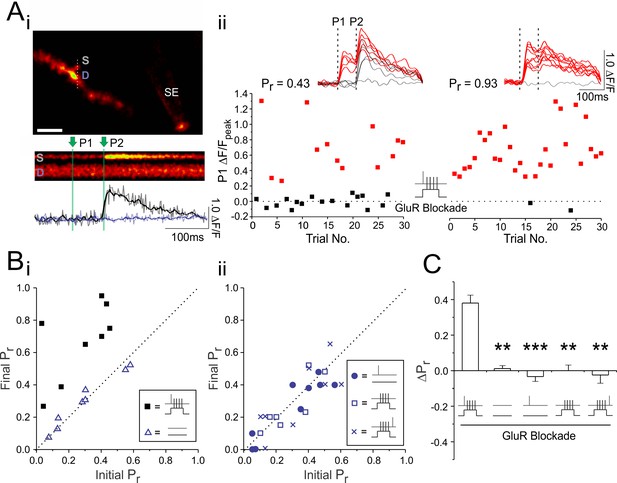
LTP induction in glutamate receptor blockade is associated with an increase in Pr.
(Ai) Example experiment. Top, an image of a CA1 neuronal dendrite loaded with Oregon Green BAPTA-1 Ca2+-sensitive dye with a stimulating electrode (SE) placed close to the dendrite in order to activate synapses within the vicinity (scale bar: 5 µm). Example of Ca2+ transients were evoked by two stimulation pulses (P1 and P2; green vertical lines) are shown in the spine head (S) and underlying dendrite (D). Below the line scan are raw (grey) and smoothed (colored) traces quantifying fluorescence changes (ΔF/F) in the spine (black trace) and dendrite (purple trace). (Aii) The peak ΔF/Fs in the spine head following the first of the two stimulation pulses were plotted across 30 paired pulse stimulation trials given at baseline and 30 min following paired stimulation, which was delivered in full glutamate receptor blockade (50 µM D-AP5, 10 µM NBQX, 500 µM R,S-MCPG, and 10 µM LY341495). Red squares denote fluorescent increases above noise. Smoothed Ca2+ traces from the last 10 trials are shown above each graph. Pr was calculated as the proportion of total stimulation trials in which the first pulse resulted in a fluorescent increase above noise. (B) Group data. For each experiment, the imaged synapse’s initial Pr is plotted against its final Pr, calculated 30 min following one of five different stimulation paradigms. The broken diagonal line represents the expected trend if Pr is unchanged across conditions. Only causal pairing of pre- and postsynaptic activity generated increases in Pr. (C) Average change in Pr. Error bars represent S.E.M. (n = 8–10 spines per condition). Asterisks denotes significance differences between the first group in the graph (**p<0.01; ***p<0.001; Kruskal-Wallis with post-hoc Dunn’s test).
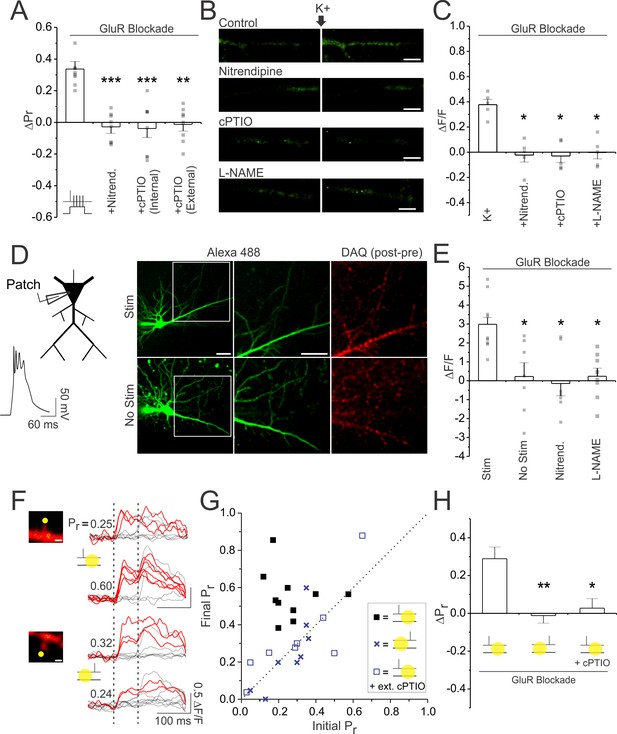
Postsynaptic depolarization increases Pr by promoting dendritic release of NO in a manner dependent on L-VGCCs.
(A) Average changes in Pr. Paired stimulation was delivered in full glutamate receptor blockade (50 µM D-AP5, 10 µM NBQX, 500 µM R,S-MCPG, and 10 µM LY341495), under control conditions, following treatment with the L-VGCC antagonist nitrendipine (20 µM), or following either the bath (100 µM) or intracellular (5 mM bolus-loaded) application of the NO scavenger cPTIO. (B) Images of CA1 apical dendrites loaded with NO-sensitive dye DAF-FM (250 µM bolus-loaded), prior to and following K+ (45 mM) mediated depolarization (scale bar: 5 µm) in control conditions, or in the presence of nitrendipine, cPTIO, or the NO synthesis inhibitor L-NAME (100 µM; pre-incubation). K+ stimulation evoked NO-sensitive and L-VGCC dependent increases in DAF-FM fluorescence. (C) Average K+ induced fluorescence change (ΔF/F) of DAF-FM in apical dendrites. (D) Slices were preloaded with the NO-sensitive dye DAQ. Right. Example images of CA1 pyramidal neurons patched and loaded with Alexa Fluor 488 are shown, along with the associated change in DAQ fluorescence (post-pre stimulation images) recorded either following stimulation with 600 complex spikes (Stim) or following no stimulation (No stim). Images in white box are magnified in the adjacent image (scale bar: 5 µm). Left. Example of the complex spikes used during stimulation. (E) Average K+ induced fluorescence change (ΔF/F) of DAF-FM in apical dendrites. Stimulation evoked NO-sensitive and L-VGCC dependent increases in DAQ fluorescence. (F) Samples of 10 superimposed Ca2+ traces evoked in imaged spines (scale bar: 1 µm) by paired pulse stimulation (broken vertical bars); red traces depict successful release events to the first of the two pulses. Samples are taken from baseline and 25–30 min following a stimulation paradigm. The paradigm consisted of delivering presynaptic stimuli either (top) 7–10 ms before or (bottom) 7–10 ms after NO photolysis at the synapse (yellow circle); photolysis occurred in glutamate receptor blockade and in the absence of postsynaptic depolarization. Pr was calculated as the proportion of total stimulation trials in which the first pulse resulted in a successful release event. In some experiments NO photolysis was conducted in cPTIO. Only causal pairings of presynaptic activity and NO release led to increases in Pr. (G) Final Pr measured 25–30 min following the stimulation paradigm plotted against the initial Pr for each synapse. The broken diagonal line represents the expected trend if Pr is unchanged across conditions. (H) Average change in Pr. Error bars represent S.E.M. (n = 5–13 per condition). Asterisks denote significant differences from the control group (***p<0.001; **p<0.01; *p<0.05; Kruskal-Wallis with post-hoc Dunn’s test).
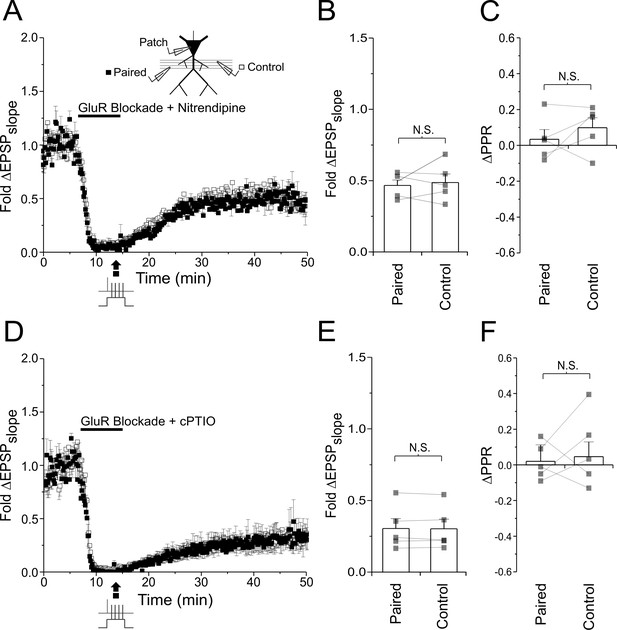
Paired stimulation fails to induce LTPpre in acute hippocampal slices when L-VGCC or NO signalling is blocked.
EPSPs were recorded from two independent Schaffer-collateral pathways in acute hippocampal slices. LTP was induced in full glutamate receptor blockade (100 µM D-AP5, 10 µM NBQX, 500 µM R,S-MCPG, and 10 µM LY341495) by causal pairings of single presynaptic stimuli and postsynaptic depolarization in the form of a complex spike (60 times at 5 Hz). Only one pathway (black box) was active during paired stimulation, the other pathway served as a control (white boxes). Pairing occurred in the presence of either the L-VGCC inhibitor nitrendipine (A–C) or the NO scavenger cPTIO (D–F). (A,D) Average fold change in the EPSP slope plotted against time for both control and paired pathways. (B,E) Average fold change in EPSP slope in the paired and control pathways 30 min following paired stimulation and drug washout. (C,F) Average change in PPR for paired and control pathways. Under L-VGCC or NO signalling blockade, paired stimulation failed to produce increases in EPSP slope and decreases in PPR. Error bars represent S.E.M. (n = 6 cells per condition). N.S. denotes no significant difference (Wilcoxon matched pairs signed rank test).
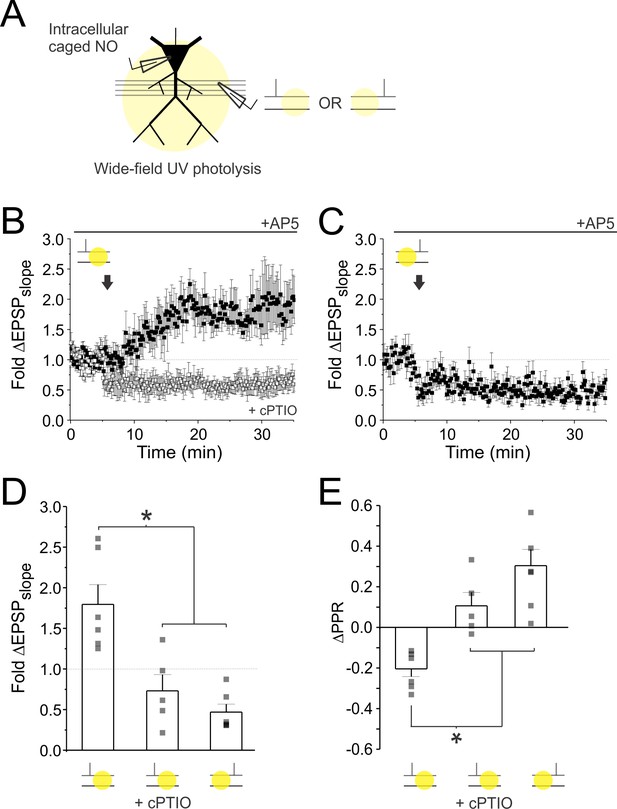
Causal pairing of NO photolysis with presynaptic stimulation induces LTPpre of EPSPs recorded in acute slices.
(A) Experimental setup. CA1 pyramidal neurons in acute slices were patched and intracellularly loaded with caged NO. AMPAR-mediated EPSPs were recorded in AP5 (50 µM D-AP5). A UV flash lamp was used to trigger rapid wide-field uncaging (large yellow circle) either 7–10 ms before or after presynaptic stimulation (60 pulses at 5 Hz). (B) Average fold change in EPSP slope plotted against time at baseline and following casual pairing of presynaptic activity with NO photolysis (arrow), either in the presence or absence of cPTIO. (C) Average fold changes in EPSP slope plotted against time at baseline and following anti-causal pairing of presynaptic activity with NO photolysis. (D) Average fold change in EPSP slope. (E) Average change in PPR. Only casual pairings of presynaptic activity and NO photolysis induced LTPpre. Error bars represent S.E.M. (n = 5–6 cells per condition). Asterisks denotes significance differences between groups (*p<0.05; Kruskal-Wallis with post-hoc Dunn’s tests).
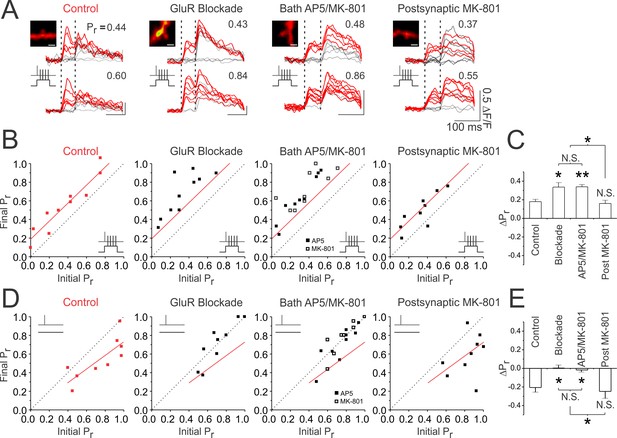
Inhibition of presynaptic NMDARs prevents decreases in Pr induced by glutamate release.
(A) Samples of 10 superimposed Ca2+ traces evoked in imaged spines (white scale bar: 1 µm) by paired pulse stimulation (2 stimuli delivered 70 ms apart; represented by the vertical broken lines); red traces depict successful release events to the first of the two pulses. Pr was calculated as the proportion of total stimulation trials in which the first pulse resulted in a successful release event. For each spine, sample Ca2+ traces are shown during baseline and 25–30 min following paired stimulation. Experiments were also conducted with unpaired stimulation, in which presynaptic activity (60 pulses at 5 Hz) was delivered in the absence of postsynaptic depolarization. (B, D) Paired or unpaired stimulation was delivered in control conditions or following: full glutamate receptor blockade (50 µM D-AP5, 10 µM NBQX, 500 µM R,S-MCPG, and 10 µM LY341495), bath application of AP5 (50 µM) or MK-801 (20 µM), or intracellular application of MK-801 postsynaptically. For each experiment, the final Pr measured 25–30 min following (B) paired or (E) unpaired stimulation is plotted against the initial Pr measured at baseline. Red trendlines have been fitted to control data to guide visual comparison. The broken diagonal line represents the expected trend if Pr is unchanged by stimulation. Inhibition of presynaptic NMDARs by full glutamate receptor blockade or by bath application of AP5 or MK-801 augmented increases in Pr produced by paired stimulation and prevents decreases in Pr produced by unpaired stimulation. (C,E) Average changes in Pr. Changes in Pr were always more positive under conditions in which presynaptic NMDARs are blocked, suggesting that glutamate release drives decreases in Pr via presynaptic NMDAR signalling. Error bars represent S.E.M. (n = 9–18 spines per condition). Asterisks denote significant differences (**p<0.01; *p<0.05; Kruskal-Wallis with post-hoc Dunn’s test). N.S. denotes no significant difference. All comparisons were made against control data unless otherwise specified.
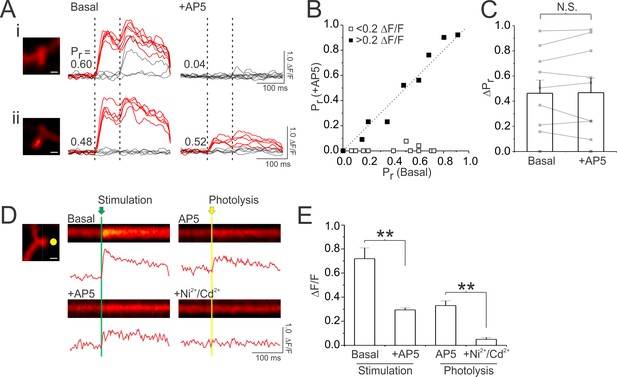
Spine Ca2+transients in NMDAR blockade are mediated by VGCCs, and can be used to accurately measure Pr.
(A) NMDAR blockade does not impede measurements of Pr. Samples of 10 superimposed Ca2+ traces evoked in imaged spines (white scale bar: 1 µm) by paired pulse stimulation (broken vertical lines show 2 stimuli delivered 70 ms apart); red traces depict successful release events to the first of the two pulses. Pr was calculated as the proportion of total stimulation trials in which the first pulse resulted in a Ca2+ response in the dendritic spine. (Ai,ii) For two imaged spines, sample Ca2+ traces are shown prior to and following bath application of AP5 (50 µM). AP5 abolished Ca2+ transients (ΔF/F < 0.2) in only one of the two spines. (B) For each synapse imaged, the Pr measured under basal conditions is plotted against the Pr measured during bath application of AP5. The broken diagonal line represents the expected trend if Pr is unchanged across conditions. For about 50% of synapses, a significant Ca2+ transient remained in AP5 (black boxes; ΔF/F > 0.2); at these sites, Pr measured under basal conditions was unchanged by AP5. (C) Group data depicted no significant difference between Pr measured under basal conditions and in AP5 for synapses for which an AP5-insensitive Ca2+ transient could be detected (ΔF/F > 0.2). (D) Residual Ca2+ influx in AP5 is mediated by VGCCs. Images of sample Ca2+ responses evoked by stimulation or glutamate photolysis at a single spine under various pharmacological manipulations. Below each image are traces quantifying the change in fluorescence intensity of the Ca2+ response (ΔF/F). At the spine shown, AP5 reduced, but did not abolish, Ca2+ responses evoked by stimulation. Inhibition of VGCCs by Ni2+ (100 µM) and Cd2+(100 µM) abolished the AP5-insensitive component of the Ca2+ transient; since Ni2+ and Cd2+ inhibit transmitter release, glutamate release was simulated via photolysis under these conditions. (E) Average peak amplitude of evoked Ca2+ responses (ΔF/F) across experimental conditions. Ca2+ responses evoked by glutamate photolysis did not significantly differ from those evoked by electrical stimulation, and was significantly reduced by Ni2+ and Cd2+ application. Error bars represent S.E.M. (n = 5–12 spines per condition). Asterisks denote significant differences (**p<0.01; Mann-Whitney or Wilcoxon matched pairs signed rank tests). N.S. denotes no significant difference.
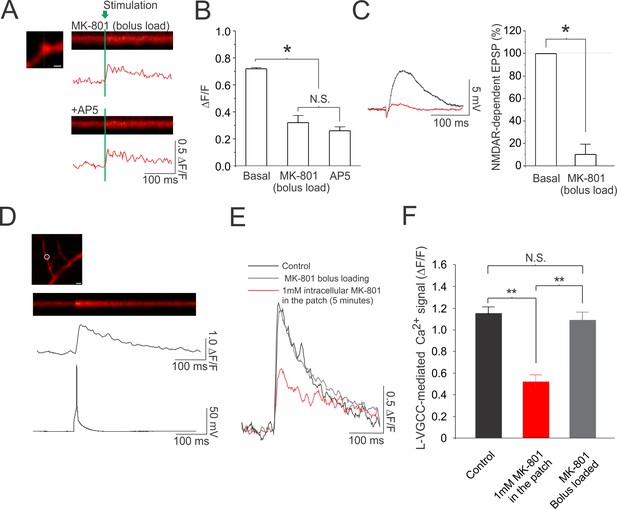
Bolus loading of MK-801 intracellularly blocks NMDARs without affecting L-type voltage-gated Ca2+channels.
MK-801 bolus loading consisted of transiently patching CA1 neurons (60 s) with glass electrodes (4–8 MΩ) containing 5 mM MK-801. The drug was given approximately 20 min to diffuse and take effect before starting experiments. (A) Images of sample spine Ca2+ responses evoked by single presynaptic stimuli taken 20 min after bolus loading of MK-801, and following the bath application of AP5 (scale bar: 1 µm). Below each image are traces quantifying the change in fluorescence intensity of the Ca2+ response (ΔF/F). Bolus loading of MK-801 occluded the effects of AP5 on spine Ca2+ signals. (B) Average peak Ca2+ fluorescence amplitude (ΔF/F) evoked by electrical stimulation in control conditions, and following either bolus loading of MK-801 or bath application of AP5 (50 µM); response amplitudes recorded in AP5 and MK-801 did not differ, and were significantly lower than those recorded in control conditions, in which NMDARs were not blocked. (C) Left. Sample trace of NMDAR-dependent EPSPs recorded in AMPAR blockade (10 µM NBQX) prior to and following bolus loading of MK-801 in the recorded neuron. Bolus loading of MK-801 abolished the NMDAR-dependent EPSP. Right. Average NMDAR-dependent EPSP recorded prior to and following bolus loading of MK-801. Bolus-loading of MK-801 does not affect L-VGCC currents. (D) Sample image of a L-VGCC dependent dendritic Ca2+ signal evoked by an action potential (scale bar: 5 µm). Ca2+ transients were recorded in a cocktail of VGCCs antagonists (10 µM mibefradil, 0.3 µM SNX-482, and 1 µM ɯ-conotoxin-MVIIC) in order to isolate L-VGCC-mediated Ca2+ transients (Bloodgood and Sabatini, 2007). Below the image is a trace quantifying the change in fluorescence intensity of the Ca2+ response (ΔF/F), and a trace showing the simultaneously recorded membrane potential. (E) Sample of L-VGCC mediated Ca2+ signals recorded from a patched CA1 neuron under control conditions, following bolus-loading of MK-801, and following traditional MK-801 loading with a patch electrode containing 1 mM MK-801 for 5 min. To ensure dilution of postsynaptic factors did not confound experimental results, for control and bolus-loading conditions, neurons were re-patched for 5 min with pipettes containing standard internal solution prior to imaging. Bolus-loading of MK-801 did not affect L-VGCC Ca2+ transients triggered in response to single action potentials, whereas prolonged exposure to 1 mM MK-801 in the patch pipette did. (F) Average changes in the L-VGCC mediated Ca2+ signal. Error bars represent Error bars represent S.E.M. (n = 5–12 spines/cells per condition). Asterisks denote significant differences (*p<0.05; Wilcoxon matched pairs signed rank test, Mann-Whitney test, or Kruskal-Wallis with post-hoc Dunn’s test). N.S. denotes no significant difference.
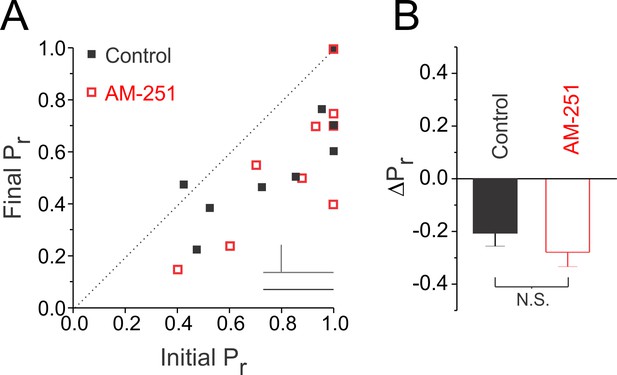
LTDpre does not require endocannabinoid signalling.
(A) Ca2+ imaging was used to determine Pr. LTDpre was induced by delivering 60 presynaptic stimuli at 5 Hz at high Pr synapses either in the presence or absence of the endocannabinoid receptor 1 antagonist AM-251 (2 µM). The final Pr measured 25–30 min following LTDpre induction is plotted against the initial Pr measured at baseline for each imaged synapse. The broken diagonal line represents the expected trend if Pr is unchanged by stimulation. LTDpre was induced in both conditions. Control data is from Figure 6D. (B) Average changes in Pr. AM-251 did not impair LTDpre induction. Error bars represent S.E.M. (n = 9 spines per condition). N.S. denotes no significant difference (Mann-Whitney test).
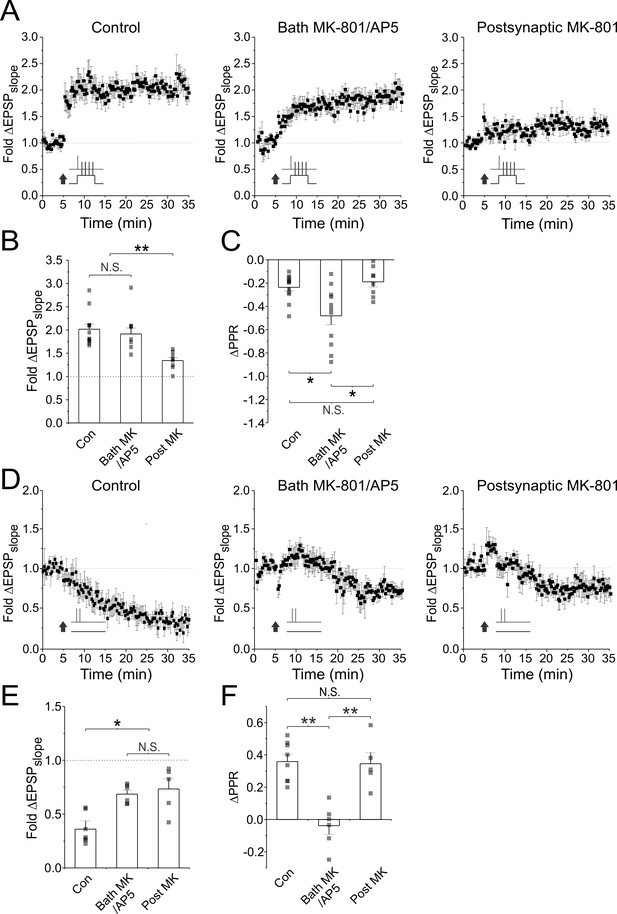
Inhibition of presynaptic NMDARs augments LTPpre and abolishes LTDpre.
(A,D) Average fold change in EPSP slopes plotted against time for plasticity induction under control conditions, or either in the presence of NMDAR blockers in the bath (MK-801 or AP5; results from both were similar), or in the postsynaptic neuron (MK-801). LTP was induced using paired stimulation. For LTD induction, since our standard unpaired stimulation protocol (60 pulses at 5 Hz) only triggered LTDpre at high Pr synapses (Pr >0.5; Figures 2E and 6D) and did not have a measurable effect on the recorded EPSP (Figure 1E), we used our unpaired, high-frequency burst protocol (2 pulses at 200 Hz repeated 60 times at 5 Hz; as in Figure 1E), which produced more robust depression of recorded EPSPs. (B,E) Average EPSP slope changes across experiments. LTP was induced regardless of whether NMDARs were blocked. This is not surprising given that our pairing protocol was capable of producing LTP of the EPSP in full glutamate receptor blockade (Figure 3). LTD was also induced across conditions, but was reduced with either intra- or extracellular NMDAR blockade. The initial time course of LTD also varied across experiments, with some cells showing no change or even a transient potentiation of the EPSP in the first 5–10 min following induction. However, all cells showed LTD by 30 min following induction. To examine specifically the presynaptic component of LTP and LTD expression we compared PPR changes 30 min post-induction across groups. (C,F) Average PPR changes across experiments. Bath application of AP5/MK-801, but not intracellular application of MK-801, augmented decreases in PPR produced by paired stimulation, and abolished increases in PPR produced by unpaired stimulation. This suggests inhibition of presynaptic NMDAR signalling augmented LTPpre and abolished LTDpre. Error bars represent S.E.M. (n = 5–12 cells per condition). Asterisks denote significant differences from (*p<0.05;**p<0.01; Kruskal-Wallis with post-hoc Dunn’s test). N.S. denotes no significant differences.
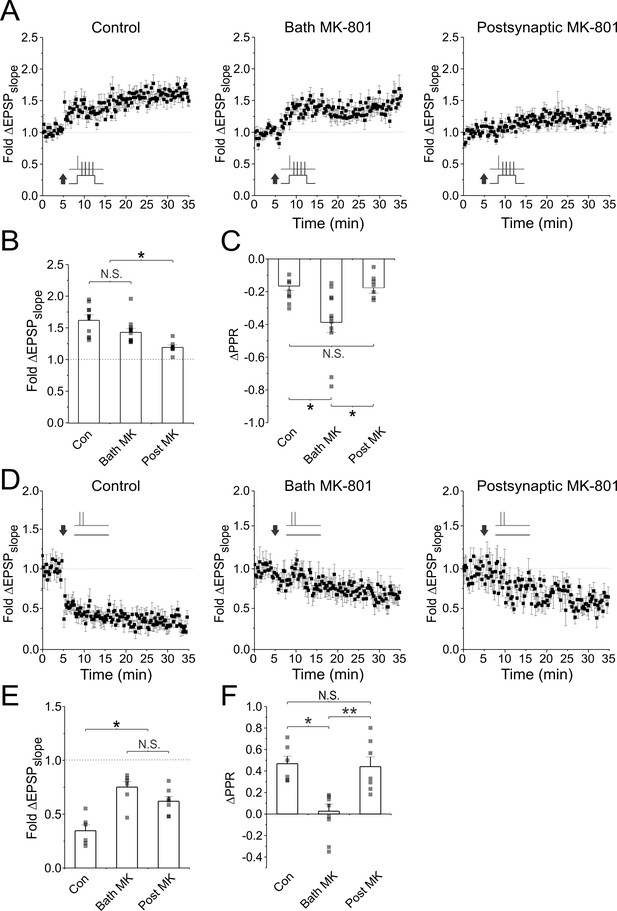
Inhibition of presynaptic NMDARs augments LTPpre and abolishes LTDpre in acute slices.
CA1 pyramidal cells in acute slices were recorded using whole-cell patch clamp. (A,D) Average fold change in EPSP slopes plotted against time for plasticity induction under control conditions, or either in the presence of NMDAR blockers in the bath (MK-801), or in the postsynaptic neuron (MK-801). Plasticity was induced either using paired stimulation to induce LTP or unpaired stimulation (2 pulses at 200 Hz repeated 60 times at 5 Hz) to induce LTD. (B,E) Average EPSP slope changes across experiments. (C,F) Average PPR changes across experiments. Bath application of MK-801, but not intracellular application of MK-801, augmented decreases in PPR produced by paired stimulation, and abolished increases in PPR produced by high frequency presynaptic stimulation alone. This suggests that inhibition of presynaptic NMDAR signalling augmented LTPpre and abolished LTDpre. Error bars represent S.E.M. (n = 6–11 cells per condition). Asterisks denote significant differences from (*p<0.05;**p<0.01; Kruskal-Wallis with post-hoc Dunn’s test). N.S. denotes no significant differences.
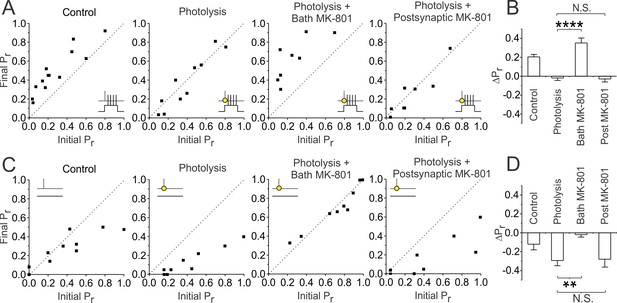
Inhibition of presynaptic NMDARs rescues the effects of glutamate photolysis on presynaptic plasticity.
(A,C) Photolysis experiments conducted in Figure 2 are shown, in which paired or unpaired stimulation (60 pulses at 5 Hz) was delivered in the presence or absence of glutamate photolysis. Experiments were repeated following either the bath (20 µM) or intracellular application of MK-801. For each experiment, the final Pr measured 25–30 min following (A) paired or (C) unpaired stimulation is plotted against the initial Pr measured at baseline. The broken diagonal line represents the expected trend if Pr is unchanged by stimulation. Bath, but not intracellular, application of MK-801 prevented photolysis-induced inhibition of LTPpre and photolysis-induced augmentation of LTDpre, suggesting that the effects of photolysis are mediated by presynaptic NMDAR signalling. (B,D) Average change in Pr. Error bars represent S.E.M. (n = 8–13 spines per condition). Asterisks denote significant differences from (****p<0.0001; **p<0.01; Kruskal-Wallis with post-hoc Dunn’s test). N.S. denotes no significant differences.
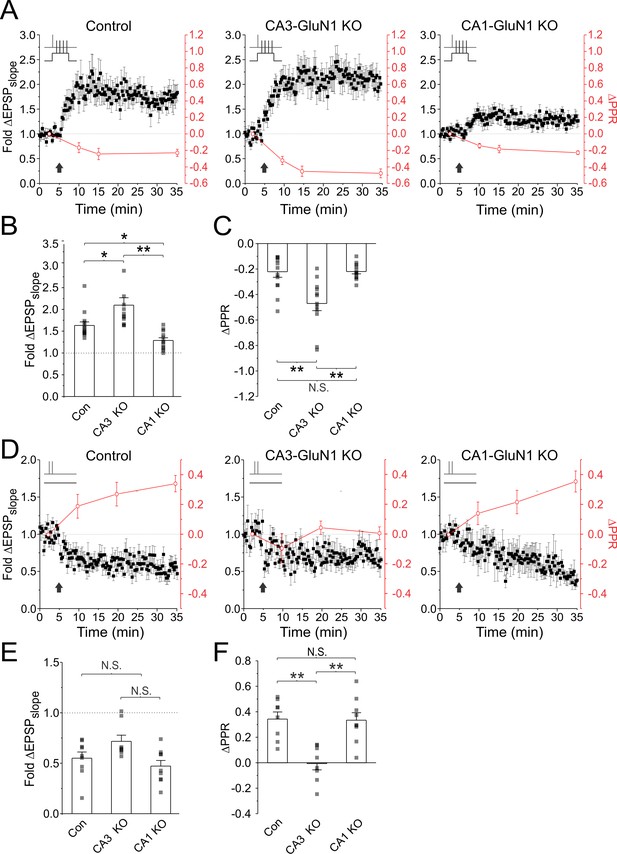
Genetic knockout of presynaptic NMDARs augments LTPpre and abolishes LTDpre.
Cre recombinase was injected either into the CA3 or CA1 region of hippocampal slices cultured from floxed Grin1 mice (Grin1fx/fx) in order to selectively knockout pre- or postsynaptic NMDARs at Schaffer-collateral synapses. Slices were recorded electrophysiologically. (A,D) Average fold change in EPSP slopes (black) and PPR (red) plotted against time for plasticity induction across conditions. Plasticity was induced either using paired stimulation to induce LTP, or unpaired stimulation (2 pulses at 200 Hz, repeated 60 times at 5 Hz) to induce LTD. (B,E) Average EPSP slope changes across experiments 30 min post induction. (C,F) Average PPR changes across experiments 30 min post induction. Knockout of pre-, but not post-, synaptic NMDARs augmented decreases in PPR produced by paired stimulation, and abolished increases in PPR produced by unpaired stimulation alone. Knockout of presynaptic NMDAR signalling therefore augmented LTPpre and abolished LTDpre. Error bars represent S.E.M. (n = 8–14 cells per condition). Asterisks denote significant differences from (**p<0.01; *p<0.05; Kruskal-Wallis with post-hoc Dunn’s test). N.S. denotes no significant differences.
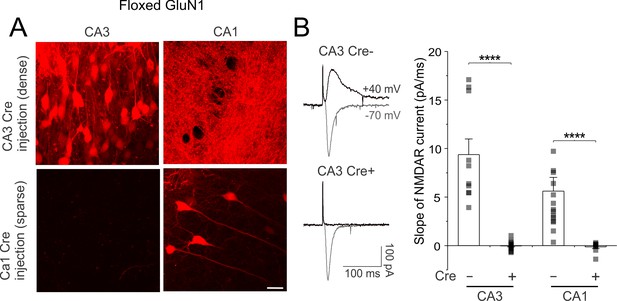
Selective knockout of pre- and postsynaptic NMDARs.
CA3 or CA1 regions of hippocampal slices cultured from floxed Grin1 mice (Grin1fx/fx) were injected with Cre recombinase and floxed tdTomato to aid visualization. (A) Sample images from CA3 and CA1 regions that were injected with or without Cre. (Top) High titre Cre injections into the CA3 led to dense and selective labelling of CA3 cells, and their processes in CA1. (Bottom) Lower titre Cre injections in CA1 led to sparse and selective labelling of CA1 cells. (B) Left. Sample AMPAR and NMDAR currents in two exemplar cells. AMPAR currents were recorded at −70 mV, NMDAR currents were recorded at +40 mV in the presence of NBQX and picrotoxin. Right. Quantification of NMDAR currents for CA3 and CA1 cells receiving Cre or control injections. Cre +cells lacked NMDAR currents by 15 days post-injection. Error bars represent S.E.M. (n = 9–19 cells per condition). Asterisks denote significant differences (****p<0.0001; Mann-Whitney test).
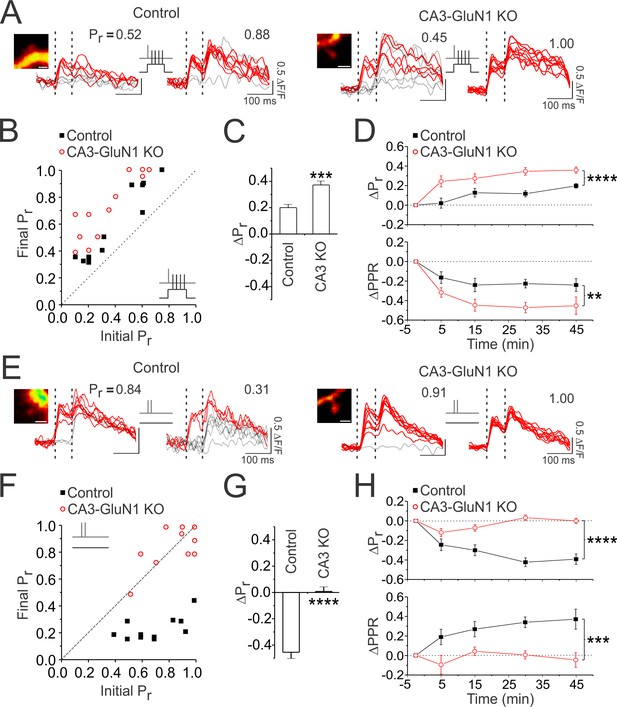
Selective knockout of presynaptic NMDARs prevents decreases in Pr induced by glutamate release.
(A,E) Samples of 10 superimposed Ca2+ traces evoked in imaged spines (white scale bar: 1 µm) by paired pulse stimulation (2 stimuli delivered 70 ms apart; represented by the vertical broken lines); red traces depict successful release events to the first of the two pulses. Pr was calculated as the proportion of total stimulation trials in which the first pulse resulted in a successful release event. For each spine, sample Ca2+ traces are shown during baseline and 30–45 min following (A) paired or (E) unpaired stimulation (2 pulses at 200 Hz repeated 60 times at 5 Hz). (B, F) Group data. For each experiment, the final Pr following (B) paired or (F) unpaired stimulation is plotted against the initial Pr measured at baseline. The broken diagonal line represents the expected trend if Pr is unchanged by stimulation. Knockout of presynaptic NMDARs augmented increases in Pr produced by paired stimulation and prevented decreases in Pr produced by unpaired stimulation. (C,G) Average changes in Pr 30–45 min following (C) paired or (G) unpaired stimulation. (D,H) Average changes in Pr at baseline, and 5, 15, 30, and 45 min following (D) paired and (H) unpaired stimulation. Changes in Pr were time matched with changes in PPR recorded in electrophysiological experiments (Figure 7); both measures show good agreement of presynaptic changes. Changes in Pr typically evolved over time, and were always more positive when presynaptic NMDARs were knocked out, suggesting that presynaptic NMDAR signalling was driving decreases in Pr. Error bars represent S.E.M. (n = 11–12 spines per condition). Asterisks denote significant differences (****p<0.0001; ***p<0.0001; **p<0.01; *p<0.05; Mann-Whitney test). All comparisons were made against control data unless otherwise specified.
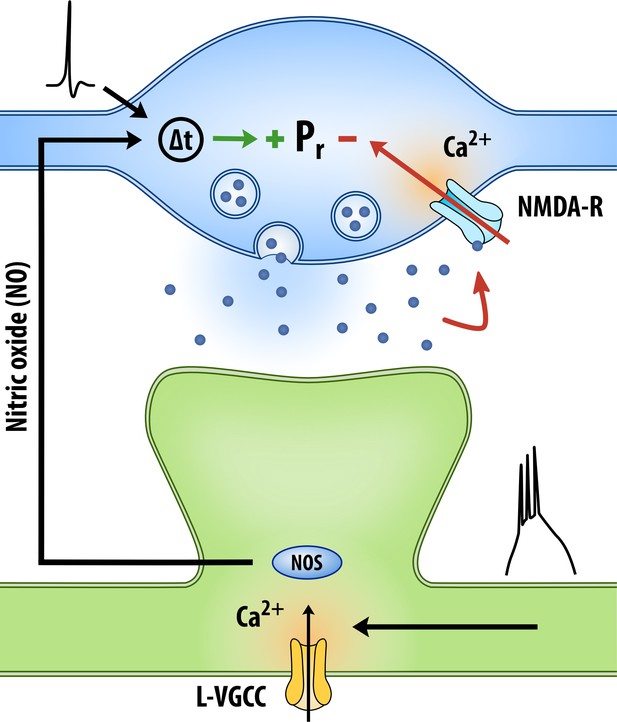
Proposed model of presynaptic plasticity.
Changes in Pr at active presynaptic terminals are determined by two opponent processes. (1) Increases in Pr are driven by Hebbian activity, during which strong postsynaptic depolarization triggers the release of NO from neuronal dendrites. NO synthesis is dependent on NO synthase (NOS), activation of which is triggered by Ca2+ influx through L-VGCCs. Importantly, NO drives an increase in Pr, but only at presynaptic terminals whose activity precedes its release. The detection of such an event requires an unidentified Hebbian detector (Δt) that is sensitive to the relative timings of NO release and presynaptic activity. (2) Decreases in Pr are driven by glutamate release, via presynaptic NMDAR signalling. Net changes in Pr depend on the strength of each process, and therefore on the amount of Hebbian signalling and glutamate release present during synaptic activity.
Additional files
-
Transparent reporting form
- https://doi.org/10.7554/eLife.29688.027