Necdin shapes serotonergic development and SERT activity modulating breathing in a mouse model for Prader-Willi syndrome
Figures
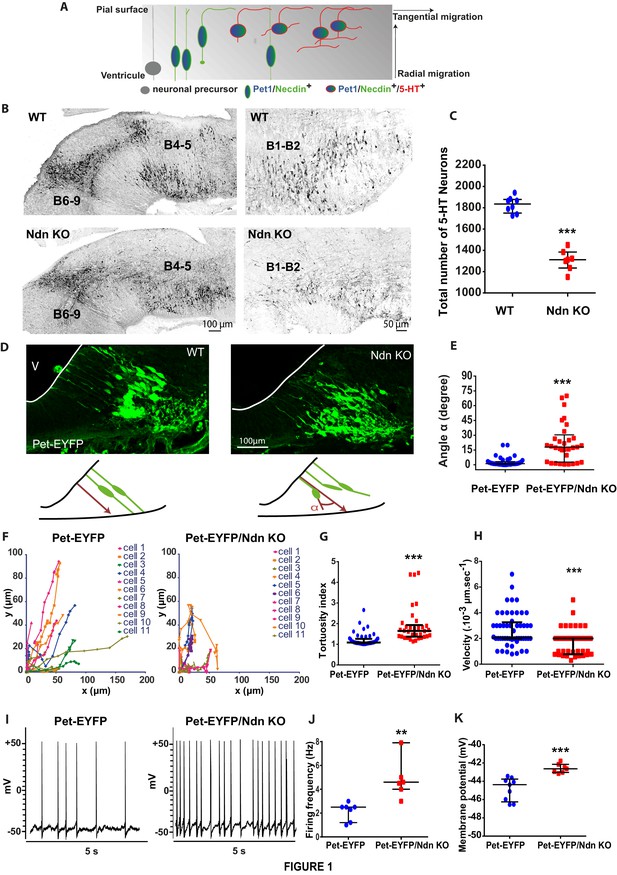
Necdin expression in 5-HT neurons and alterations of 5-HT neuronal development and activity in Ndn-KO mice.
(A) Scheme adapted from (Hawthorne et al., 2010) representing expression profiles of Necdin (green), Pet1 (blue) and 5-HT (red) throughout embryonic development of 5-HT neurons as soon as the progenitors become post-mitotic and start their radial migration by successive waves between E11.5 and E13.5. (B–C) 5-HT immunolabelling of brainstem sagittal sections of WT and Ndn-KO at E16.5. (B) 5-HT nuclei: B4 to B9 (left panels) or B1-B2 (right panels) are abnormal in Ndn-KO compared to WT. (C) Quantification of 5-HT neurons in the B1-B2 raphe nuclei (WT: 1836 (1751, 1878), n = 8; Ndn-KO: 1312 (1234, 1384), n = 7; MW, p=0.0003). (D–E) (D) Brainstem coronal sections of Pet-EYFP neurons from E11.5 WT and Ndn-KO mice illustrating radial migration from the ventricular zone (V) to the pial surface. (E) Quantification of nonlinear migration by measuring the α angle (10 cells/mouse) between the ventricular process and a virtual axis crossing the two opposing points from which neurites extend from the soma: (Angle (°): WT: 1.1(0.5, 3.5), n = 4; Ndn-KO: 18(2.8, 93.5), n = 3; MW, p<0.0001). (F–H) Confocal time-lapse analyses of cell migration of Pet-EYFP and Pet-EYFP/Ndn-KO neurons. (F) Plots representing the coordinates of individual cell bodies over time illustrate different cell migration patterns in WT (n = 4) and Ndn-KO (n = 3) Pet-EYFP neurons (11 cells/mouse). (G) Tortuosity index was increased by 52% in Ndn-KO compared to WT mice (WT: 1.08(1.01, 1.26); Ndn-KO: 1.65(1.36, 1.93); MW, p=0.0005). (H) Velocity was decreased by 37% in Ndn-KO compared to WT (Velocity (µm.s−1): WT: 2.50 10−3 (2.00, 2.93); Ndn-KO: 1.57 10−3 (1.09, 2.00); MW, p<0.0001). (I–K) Current clamp recordings of Pet-EYFP neurons (2 cells/slice) in WT (n = 3) and Ndn-KO (n = 3) brain slices. (I) Spontaneous discharge pattern of Pet-EYFP neurons; (J–K) Firing rate (J) and resting membrane potential (K) in Ndn-KO cells and aged-matched WT controls. Frequency (Hz): WT: 2.50(1.20, 2.50); Ndn-KO (4.60(4.00, 7.90); MW, p=0.0025; Voltage (mV): WT: −44.37(-46.25, –43.76); Ndn-KO: −42.64(-43.03, –42.55); MW test, p=0.0002.
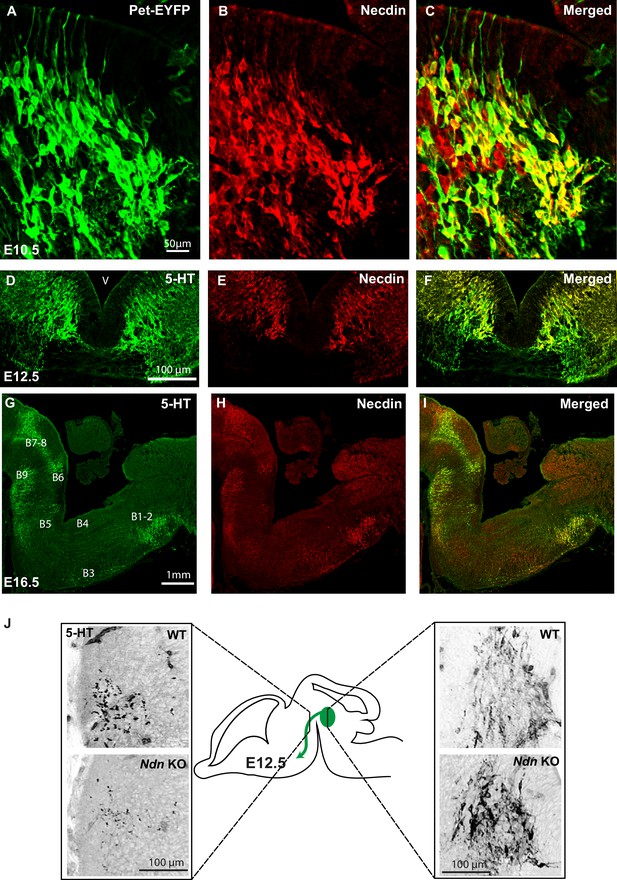
Necdin expression compared with Pet-1 and 5-HT expression throughout embryonic development and alteration of 5-HT projections in Ndn-KO embryos.
(A–I) Co-expression in WT brainstem of Necdin (B, E, H) with Pet-1 (A) and 5-HT (D,G) at E10.5 (A–C), E12.5 (D–F) and E16.5 (G–I). (J) 5-HT IHC on coronal brainstem sections at E12.5 showing 5-HT somas close to the ventricle (right panels) and their axonal projections in the mesencephalon (left panels). Increased somatic labeling concomitant with reduced labeling of the projections is observed in Ndn-KO embryos compared with WT.
Two-photon timelapse video showing somal translocation on organotypic slice cultures of Pet-EYFP neurons in WT embryos (E12.5).
https://doi.org/10.7554/eLife.32640.005Two-photon timelapse video showing somal translocation on organotypic slice cultures of Pet-EYFP neurons in Ndn-KO embryos (E12.5).
https://doi.org/10.7554/eLife.32640.006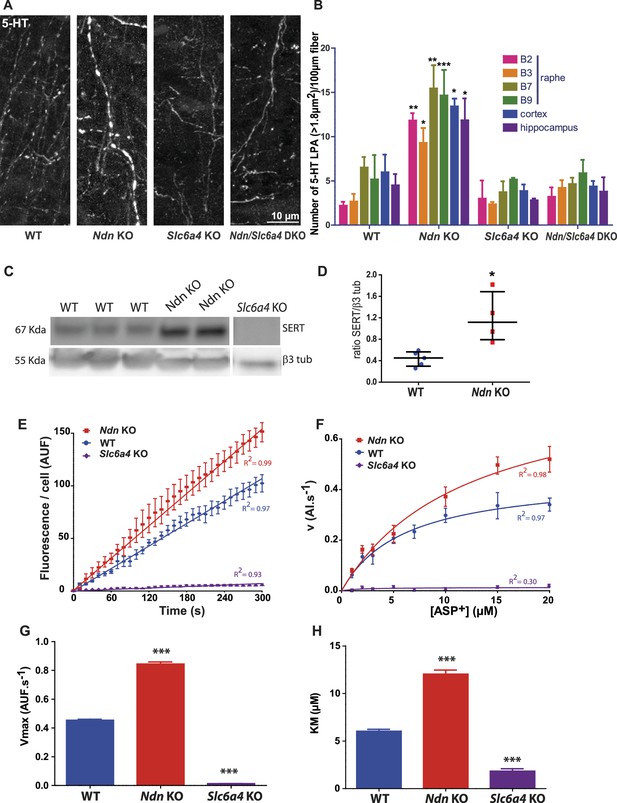
Large punctiform axonal 5-HT staining (5-HT LPAs) results from an increase in SERT expression and activity in Ndn-KO mice.
(A–B) (A) Axonal 5-HT immunoreactivity illustrating 5-HT LPAs in the raphe of WT, Ndn-KO, Slc6a4-KO and Ndn/Slc6a4-DKO neonates (P1). (B) 5-HT LPAs were counted for all different genotypes (n = 3/genotype) in the raphe nuclei (B1–B2, B3, B7, B9), cortex and hippocampus.: Raphe B1-B2: WT: 2.2 ± 0.4; Ndn-KO: 11.8 ± 0.8, p=0.003; Raphe B3: WT: 2.6 ± 0.8; Ndn-KO: 9.3 ± 1.6, p=0.01; Raphe B7: WT: 6.5 ± 1.2; Ndn-KO: 15.5 ± 2.6, p=0.07; Raphe B9: WT: 5.1 ± 2.72; Ndn-KO: 14.6 ± 2.9, p=0.0001; Cortex: WT: 5.9 ± 2.0; Ndn-KO: 13.4 ± 0.8, p=0.01; Hippocampus: WT: 4.5 ± 1.2; Ndn-KO: 11.8 ± 2.5, p=0.01. p-values determined by two-way ANOVA followed by Bonferroni post-hoc test. DKO: double KO. Bar graphs represent mean ±SEM. (C–D) (C) Western blot analysis of SERT protein expression in brainstem collected from WT, Ndn-KO and Slc6a4-KO (negative control) neonates (P1). (D) Quantification of SERT expression normalized to β3 tubulin expression: WT: 0.45 (0.29, 0.56), n = 5; Ndn- KO: 1.11 (0.75, 1.68), n = 4; MW, p=0.016). Scatter dot plots, report Q2 (Q1, Q3). (E–H) Real time and single living cell analyses of SERT uptake activity using the fluorescent substrate ASP+, a fluorescent substrate of SERT. (E) Kinetic experiment recordings of accumulation of ASP+ over time (5 min recording). Coefficient of Determination R2: WT = 0.97; Ndn KO = 0.99; Slc6a4-KO = 0.93. Mean velocity (v) of ASP+ accumulation obtained by linear regression analyses of the slopes: (AUF.s−1): WT: 0.36 ± 0.01, n = 18; Ndn-KO: 0.51 ± 0.01, n = 18, covariance (ANCOVA), p<0.0001. Non-specific accumulation of ASP+ fluorescence was evaluated in Slc6a4-KO neurons and found to be strongly low (0.02 ± 0.01 (n = 6 cells). (F) Saturation experiments using gradual concentration of APS+. Non-linear curve-fitting yielded a one-phase exponential association, with a Vmax (G) and Km (H): Vmax (AUF.s−1): WT: 0.45 ± 0.05, n = 64; Ndn-KO: 0.84 ± 0.12, n = 67; Slc6a4-KO: 0.01 ± 0.03, n = 37, p<0.0001; Km (µM): WT: 6.03 ± 1.60, n = 64; Ndn-KO: 12.03 ± 3.55, n = 67; Slc6a4-KO: 1.83 ± 1.60, n = 37, p<0.0001. AUF: arbitrary unit of fluorescence. p-values determined by K-W test, followed by Dunn post-hoc test. Bar graphs represent mean ±SEM. *p<0.05; **p<0.01; ***p<0.001. Scatter dot plots, report Q2 (Q1, Q3). **p<0.01; ***p<0.001.
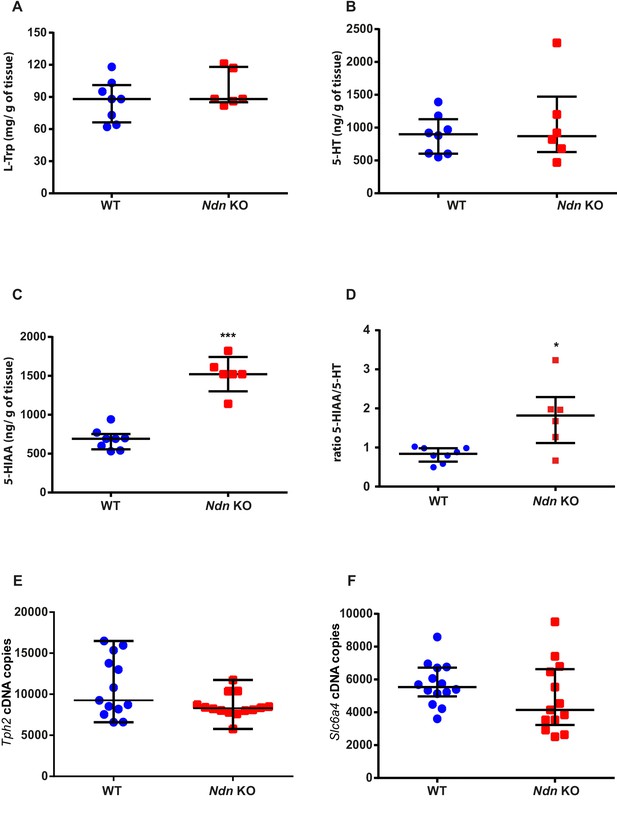
5-HT metabolism, Tph2 and Slc6a4 transcripts quantification in Ndn-KO mice.
(A–D) 5-HT metabolic analyses from medulla extracted from WT (n = 8) and Ndn-KO (n = 6) mice (at E18.5): (A) 5-HT substrate (L-Trp) (mg per gram of tissue): WT: 85.8 (64.5, 98.5); Ndn-KO: 83.6 (80.7, 11.2);MW, p=0.83, N.S. (B) 5-HT (ng per gram of tissue): WT: 877.5 (587.4, 1099); Ndn-KO: 826.5 (596.1, 1399.0); MW, p>0.99, N.S.). (C) The first metabolite of 5-HT (5-hydroxyindoacetic acid, 5-HIAA) (ng per gram of tissue): WT: 672.8 (541.1, 733.4); Ndn-KO: 1444 (1354, 1579); MW, p=0.0007. The significant increase of 5-HIAA in Ndn-KO conducts to a high 5HIAA/5-HT ratio: WT: 0.8 (0.6, 1.0); Ndn-KO: 1.8 (1.1, 2.3); MW, p=0.02. (E–F) RT-qPCR analyses of Tph2 and Slc6a4 transcripts in Ndn-KO (n = 14) and WT (n = 13) brainstems of neonate mice (P1). (E) Tph2 cDNA copies: WT: 9259 (7864, 14567); Ndn-KO: 8295 (7955, 9141), MW, p=0.12, N.S. (F) Slc6a4 cDNA copies: WT: 5541 (4974, 6720); Ndn-KO: 4149 (3228, 6629), MW, p=0.15, N.S. Neither Tph2 nor Slc6a4 presented differences between WT and Ndn-KO mice. Scatter dots represent Q2 (Q1, Q3). N.S.: non-significant; *p<0.05; ***p<0.001.
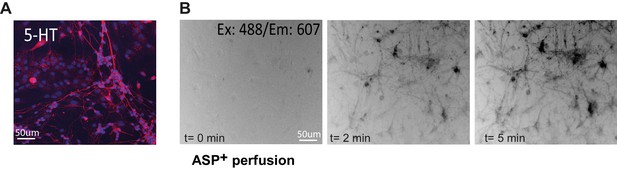
ASP+ uptake in neurons of raphe primary cultures.
(A) 5-HT immunocytochemistry on primary raphe cultures showing positive 5-HT neurons (red). (B) Time lapse illustration of ASP+ fluorescence (black) accumulation into cells bodies and fibers over 5 min of recording (t = 0; 2; 5 min).
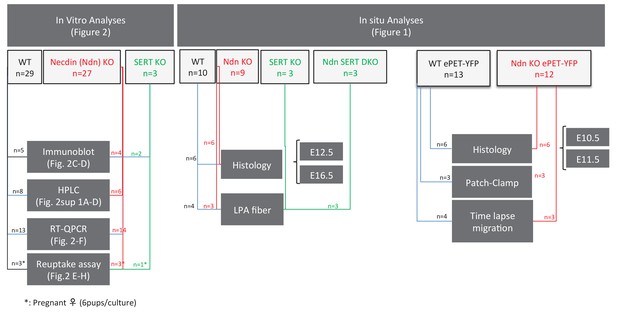
Flow diagram of mice used for in vitro and in situ analyses in Figures 1 and 2 and their corresponding supplement figures.
https://doi.org/10.7554/eLife.32640.010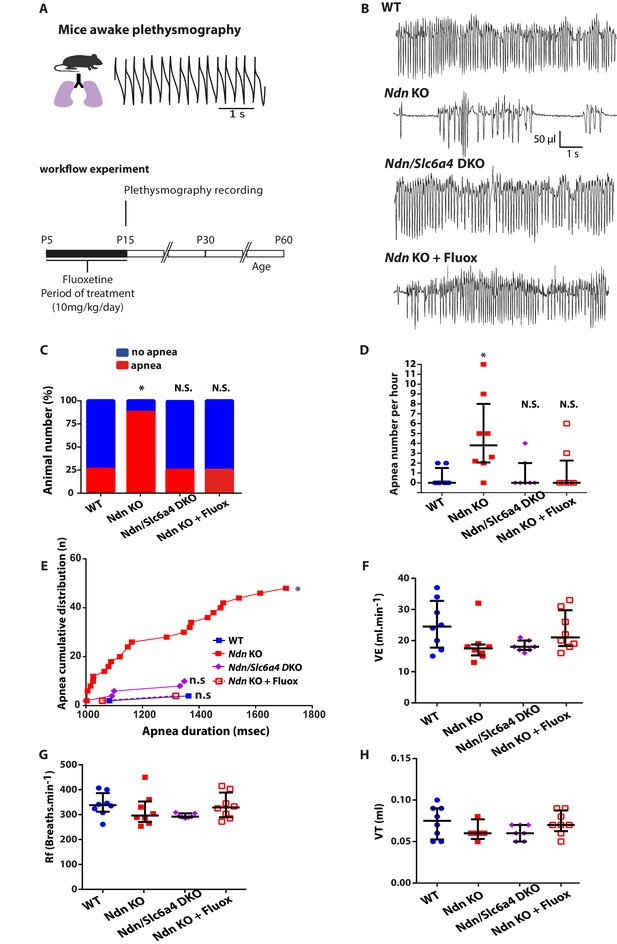
Genetic ablation or pharmacologic inhibition of SERT suppresses apnea and rescues central chemoreflex in Ndn-KO mice.
(A) Workflow experiment of constant airflow whole body plethysmography performed in unanaesthetized, unrestrained WT, Ndn-KO, Ndn/Slc6a4-DKO and Ndn-KO+Fluox mice at the age of P15. Ndn-KO and WT animals (indicated here and in the figure as WT or Ndn-KO) have been pre-treated with 0.9% NaCl from age P5 to P15. Ndn-KO mice (indicated here and in the figure as Ndn-KO+Fluox) have been pre-treated with with Fluoxetine (10 mg/Kg/day) from age P5 to P15. (B) Plethysmographic recordings of WT, Ndn-KO, Ndn/Slc6a4-DKO and Ndn-KO+Fluox mice at the age of P15. (C–E) Quantification of apnea in P15 mice. (C) Proportion of apneic mice: WT: 2 of 8; Ndn-KO: 7 of 8; corresponding respectively to 25% and 87%; Chi2 test, p=0.01. Genetic ablation of Slc6a4or early Fluoxetine treatment normalized the number of Ndn-KO apneic mice: Ndn/Slc6a4 DKO: 2 of 8, 25%; Chi2 test, p>0.99, N.S.; Ndn-KO+Fluox: 2 of 8, 25%; Chi2 test, p>0.99, N.S. (D) Number of apnea in Ndn-KO compared to WT mice: WT: 0.0 (0.0, 1.5), n = 8; Ndn-KO: 3.8 (2.0, 8.0), n = 8; p=0.01. Genetic ablation of Slc6a4 or Fluoxetine treatment normalized the number of apnea of Ndn-KO mice to WT values: Ndn/Slc6a4-DKO: 0.0 (0.0, 2.0), n = 8; p>0.99, N.S.; Ndn-KO+Fluox: 0.0 (0.0, 2.2), n = 8; p>0.99, N.S. p-values determined by KW test followed by Dunn post-hoc test with comparison to WT. (E) Cumulative distribution of apnea (number of cumulated values) over apnea duration (msec) in WT, Ndn-KO, Ndn/Slc6a4-DKO and Ndn-KO treated by Fluoxetine. Compared to WT, Ndn-KO mice demonstrated a significant increase of cumulative apnea both in term of number and duration (Kolmogorov-Smirnov test, p=0.01). However, such increase was normalized to WT after genetic deletion of Slc6a4 or Fluoxetine treatment. (F–H) Basic breathing parameters: (F) Minute ventilation, VE (the total volume breathed over one min): WT: 24.5 (17.7, 32.7), n = 8; Ndn-KO: 17.5 (15.2, 18.7), n = 8; p=0.14, N.S.; Ndn/Slc6a4-DKO: 18.0 (17.0, 20.0), n = 8; p=0.25, N.S. and Ndn-KO+Fluox: 21.0 (18.2, 29.7), n = 8; p>0.99, N.S.. (G) Frequency of breathing, Rf (breaths/min): WT: 338 (312, 3867), n = 8; Ndn-KO: 296 (270, 352), n = 8; p=0.56, N.S.; Ndn/Slc6a4-DKO: 292 (2890, 305), n = 8; p=0.16, N.S. and Ndn-KO+Fluox: 329 (289, 388), n = 8; p>0.99, N.S. (H) Tidal Volume, VT (the volume flow per breath): WT: 0.07 (0.05, 0.09), n = 8; Ndn-KO: 0.06 (0.06, 0.06), n = 8; p=0.38, N.S.; Ndn/Slc6a4-DKO: 0.06 (0.05, 0.07), n = 8; p=0.51, N.S. and Ndn-KO+Fluox: 0.07 (0.06, 0.08), n = 8; p>0.99, N.S. p-values determined by K-W test followed by Dunn post-hoc test with comparison to WT.Scatter dots represent Q2 (Q1, Q3). N.S.: non-significant; *p<0.05.
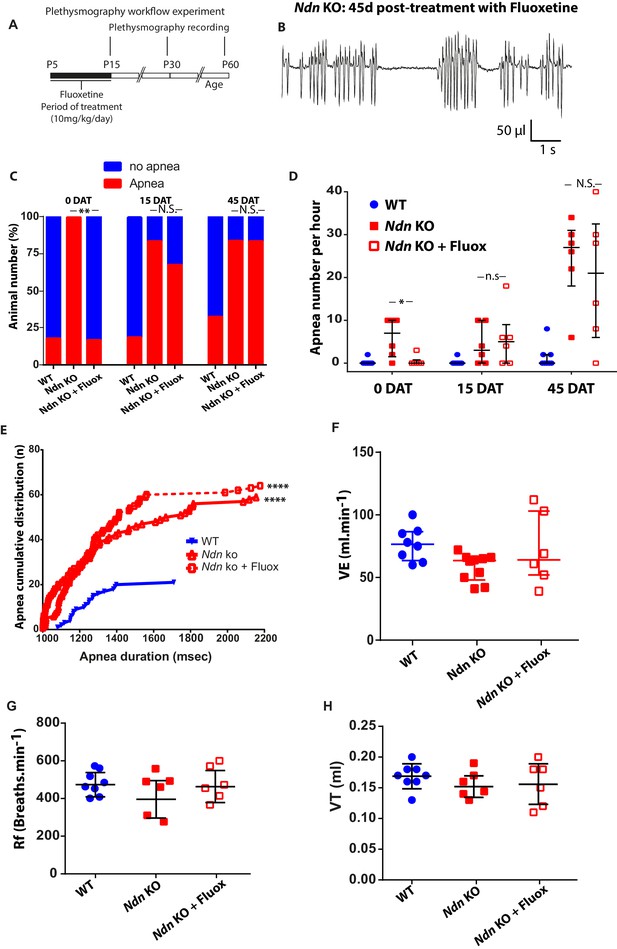
Early life Fluoxetine treatment has only short-term positive effects on Ndn-KO apneas.
(A) Workflow experiment of constant airflow whole body plethysmography performed in unanaesthetized, unrestrained WT and Ndn-KO mice at 0, 15 and 45 days after treatment (DAT). Ndn-KO and WT animals (indicated here and in the figure as WT or Ndn-KO) have been pre-treated with 0.9% NaCl from age P5 to P15. Ndn-KO mice (indicated here and in the figure as Ndn-KO+Fluox) have been pre-treated with Fluoxetine (10 mg/Kg/day) from age P5 to P15. (B) Plethysmographic recordings of early Fluoxetine treated Ndn-KO mice at 45 DAT showing apnea. (C) In the WT and Ndn-KO groups, the prevalence of mice with apnea did not change over time, although a significantly proportion of apneic mice were found in Ndn-KO group compared to WT group. Comparison of the proportion of apneic mice over post-treatment time points between Ndn-KO treated groups (vehicle or Fluoxetine) confirmed that at 0 DAT, Fluoxetine significantly decreased the prevalence of Ndn-KO apneic mice (Ndn-KO+vehicle: 0 of 6, 100%; Ndn-KO+Fluox: 1 of 6; 16%, n = 6; Chi2, p=0.01), to similar level of WT (WT: 1 of 6; 16%). However, this difference was not anymore observed at 15 DAT (Ndn-KO+vehicle: 1 of 6; 16%; Ndn-KO+Fluox: 2 of 6; 33%, n = 6; Chi2, p=0.66, N.S.) and 45 DAT (Ndn-KO+vehicle: 1 of 6; 16%; Ndn-KO+Fluox: 1 of 6; 16%, n = 6; Chi2, p=1.0, N.S.). N.S.: non-significant; **p<0.01. (D) Number of apnea over time (0, 15, 45 DAT) in the different mice groups. Except for WT group which values were stable over time, vehicle or Fluoxetine-treated Ndn-KO mice present an increase in the number of apnea over time which appears significant at 45 DAT compared to 0 DAT: Ndn-KO (0 DAT: 7(1.5, 10); 45 DAT: 27(18, 31); n = 6, p=0.001); Ndn-KO+Fluox (0 DAT: 0(0, 0.75); 45 DAT: 21(6, 32); n = 6, p=0.001). Comparison between the Ndn-KO treated groups (vehicle or Fluoxetine) confirmed significant difference in the number of apnea at 0 DAT (p=0.041) but revealed non-significant difference at 15 DAT (Ndn-KO: 3(0, 10); Ndn-KO+Fluox: 5(0, 9), p>0.99; N.S.) and 45 DAT (Ndn-KO: 27(18, 31); Ndn-KO+Fluox: 21(6, 32), p=0.75; N.S.). P-values determined by two-way repeated-measure (RM) ANOVA followed by Bonferroni post-hoc test. Scatter dots represent Q2 (Q1, Q3). N.S.: non-significant; *p<0.05. (E) Cumulative distribution of apnea (number of cumulated values) over apnea duration measured in WT, Ndn-KO and Ndn-KO treated with Fluoxetine (45 DAT). At this stage, the distribution of apnea duration appeared similar between treated and untreated Ndn-KO mice suggesting that early life Fluoxetine treatment in Ndn-KO had no long term effect on apnea in those animals. Both Ndn-KO groups (vehicle or Fluoxetine-treated) appeared significantly different to WT (p=0.0001, Kolmogorov-Smirnov test). ****p<0.0001. (F–H) Breathing parameters measured at 45 DAT: (F) Minute ventilation, VE: WT: 76.5(61.5, 88.7), n = 8; Ndn-KO: 58.5(41.7, 64.2), n = 6; p=0.16, N.S.; and Ndn-KO+Fluox: 65.0(48.7, 105.3), n = 6; p=0.96, N.S.; (G) Frequency of breathing, Rf: WT: 469(4067, 531), n = 8; Ndn-KO: 368 (302, 460), n = 6; p=0.13, N.S.; and Ndn-KO+Fluox: 450 (402, 579), n = 6; p>0.99, N.S; (H) Tidal Volume, VT: WT: 0.17(0.15, 0.18), n = 8; Ndn-KO: 0.14(0.13, 0.16), n = 6; p=0.25, N.S.; and Ndn-KO+Fluox: 0.16(0.11, 0.18), n = 6; p=0.89, N.S p-values determined by K-W test followed by Dunn post-hoc test with comparison to WT. Scatter dots represent Q2 (Q1, Q3).
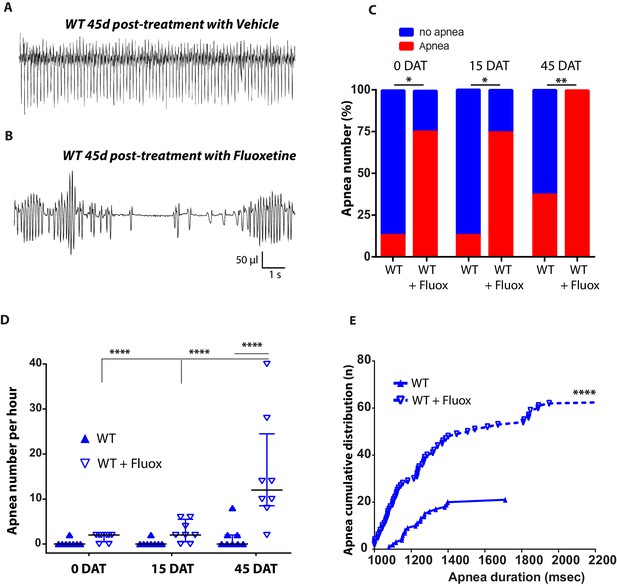
Early life treatment of Fluoxetine on respiratory apnea in wild-type mice.
(A–B) Plethysmographic recordings of WT mice at 45DAT pre-treated either with (A) 0.9% NaCl (indicated here as WT) or (B) Fluoxetine (indicated here as WT+Fluox) (10 mg/Kg/day) from age P5 to P15 (see workflow in Figure 3—figure supplement 1). Note in B the appearance of apnea for WT mice, which received the treatment. (C) Proportion of apneic mice, at 0, 15 and 45 DAT, in Fluoxetine-treated WT mice compared with WT mice: 0 DAT: WT = 1 of 8, 12.5%, WT+Fluox: 6 of 8, 75%; Chi2, p=0.011; 15 DAT: WT = 1 of 8, 12.5%, WT+Fluox: 6 of 8, 75%; Chi2, p=0.011; 45 DAT: WT = 3 of 8, 37.5% WT+Fluox: 8 of 8, 100%; Chi2, p=0.010. (D) Number of apnea per hour at different DAT: 0; 15; 45 in Fluoxetine-treated and WT groups. Except for WT group whose values were stable over time, we found for Fluoxetine-treated WT mice a significant increase of the number of apnea over time (0 DAT: 2(0.5, 2); 15 DAT: 2(0.5, 5.5); 45 DAT: 12(8.5, 24.5); p<0.0001). Comparison between both WT groups confirmed significant difference in the number of apnea at 15 DAT (WT: 0(0.2); WT+fluox: 12(8.5, 24.5)) p<0.0001). p-values determined by two-way RM ANOVA followed by Bonferroni post-hoc test. (E) Cumulative distribution of apnea (number of cumulated values) over apnea duration measured at 45 DAT in WT and WT treated with Fluoxetine. Fluoxetine treatment produces a significant increase in apnea accumulation over apnea duration (Kolmogorov-Smirnov test, p=0.0001). *p<0.05; **p<0.01; ****p<0.0001.
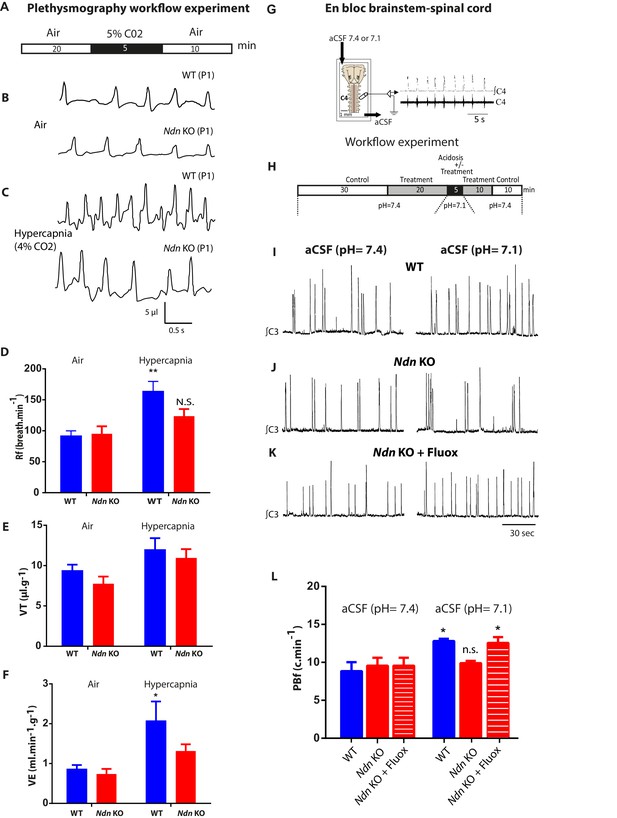
Alteration of respiratory chemoreflex in Ndn-KO neonates is rescued by Fluoxetine.
(A–F) Effect of hypercapnia on in vivo ventilatory parameters of WT and Ndn-KO neonates. (A) Workflow experiment of constant airflow whole body plethysmography performed in unanaesthetized, unrestrained WT, Ndn-KO neonates at P0-P1 when breathing either air or hypercapnic mixture containing 4% CO2 in air for 5 min. Data for analyses were collected in the last 5 min (air) or the last min (hypercapnia). (B–C) (B) Plethysmographic recordings of WT and Ndn-KO neonates when breathing air or (C) at 5th min upon hypercapnic respiratory challenge. (D) Respiratory frequency (Rf) in WT and Ndn-KO pups when subjected to hypercapnic stress: WT Air: 91 ± 8; WT hypercapnia: 163 ± 16; n = 8, p=0.004; Ndn-KO Air: 95 ± 12; Ndn-KO hypercapnia: 123 ± 11; n = 8, p=0.31, N.S. p-values determined by two-way-ANOVA test followed by Bonferroni post-hoc test. Bar graphs represent mean ±SEM; **p<0.01; N.S.: non-significant. (E) Tidal Volume (VT) (µl.g−1) in WT neonates: WT Air: 9 ± 0.7; WT hypercapnia: 12 ± 1; n = 8, p=0.12 N.S.; in Ndn-KO: Ndn-KO Air: 7.5 ± 0.9; Ndn-KO hypercapnia: 10.9 ± 1.1; n = 8, p=0.055. p-values determined by two-way ANOVA test followed by Bonferroni post-hoc test. (F) Minute Ventilation (VE) (ml.min−1.g−1) in WT neonates: WT Air: 0.8 ± 0.1; WT hypercapnia: 2.1 ± 0.1; n = 8, p=0.01; in Ndn-KO: Ndn-KO Air: 0.7 ± 0.1; Ndn-KO hypercapnia: 1.3 ± 0.2; n = 8, p=0.3 N.S. p-values determined by two-way ANOVA test followed by Bonferroni post-hoc test. Bar graphs represent mean ±SEM. *p<0.05. (G–H) Effect of Fluoxetine treatment on the resting phrenic burst frequency (PBf) and the PBf response to acidosis in Ndn-KO medulla preparations. (G) Electrophysiological recordings of PBf produced in vitro in WT and Ndn-KO en bloc brainstem-spinal cord preparations at P0-P1 when superfused first with neutral artificial cerebrospinal fluid (aCSF) (pH 7.4) and then acidified aCSF (pH 7.1). (H) Workflow experiment of the electrophysiological recordings on medullary preparations to assess central chemosensitivity in WT and treated with Fluoxetine (20 μM) or untreated Ndn-KO mice. (I–K) Examples of continuous electrophysiological recordings of rhythmic phrenic bursts produced in en bloc brainstem-spinal cord preparations of (I) one WT, (J) one Ndn-KO and (K) one Ndn-KO treated with Fluoxetine (20 µM) pup and superfused with first neutral aCSF (pH 7.4) (left column recordings) or acidified aCSF (pH 7.1) (right column recordings). (L) Quantifications of the resting PBf (c.min−1) of en bloc preparations superfused with neutral aCSF (pH 7.4) or acidified aCSF (pH 7.1) respectively of WT (WT (pH 7.4): 8.8 c.min−1 ±1.2; WT (pH 7.1) 12.8 ± 0.3 c.min−1; n = 12, p<0.001), Ndn-KO (Ndn-KO (pH 7.4): 9.5 ± 1.0; Ndn-KO (pH 7.1): 9.8 ± 0.3 c.min−1; n = 12, p=0.41, N.S.) and Ndn-KO treated with Fluoxetine: (Ndn-KO+Fluox (pH 7.4): 9.6 ± 0.3; Ndn-KO+Fluox (pH 7.1): 12.6 ± 0.7; n = 12, p=0.04). Noticeably, under neutral aCSF (pH 7.4) no difference was observed between WT, Ndn-KO and Ndn-KO+Fluox. However, in acidified aCSF (pH 7.1), Fluoxetine significantly increased the PBf of Ndn-KO preparations. p-values determined by two-way ANOVA test followed by Tukey post-hoc test. Bar graphs represent mean ±SEM. N.S: non-significant; *p<0.05.
-
Figure 4—source data 1
Plethysmography data before and after hypercapnia in WT and Ndn-KO mice.
- https://doi.org/10.7554/eLife.32640.018
-
Figure 4—source data 2
Electrophysiology data of rhythmic phrenic bursts frequency during acidosis in WT and Ndn-KO preparations - before and after Fluoxetine treatment.
- https://doi.org/10.7554/eLife.32640.019
-
Figure 4—source data 3
Relates to Figure 4—figure supplements 1 and 2.
Electrophysiology data of rhythmic phrenic bursts frequency during acidosis in WT and Ndn-KO preparations - before and after 8-OHDPAT treatment.
- https://doi.org/10.7554/eLife.32640.020
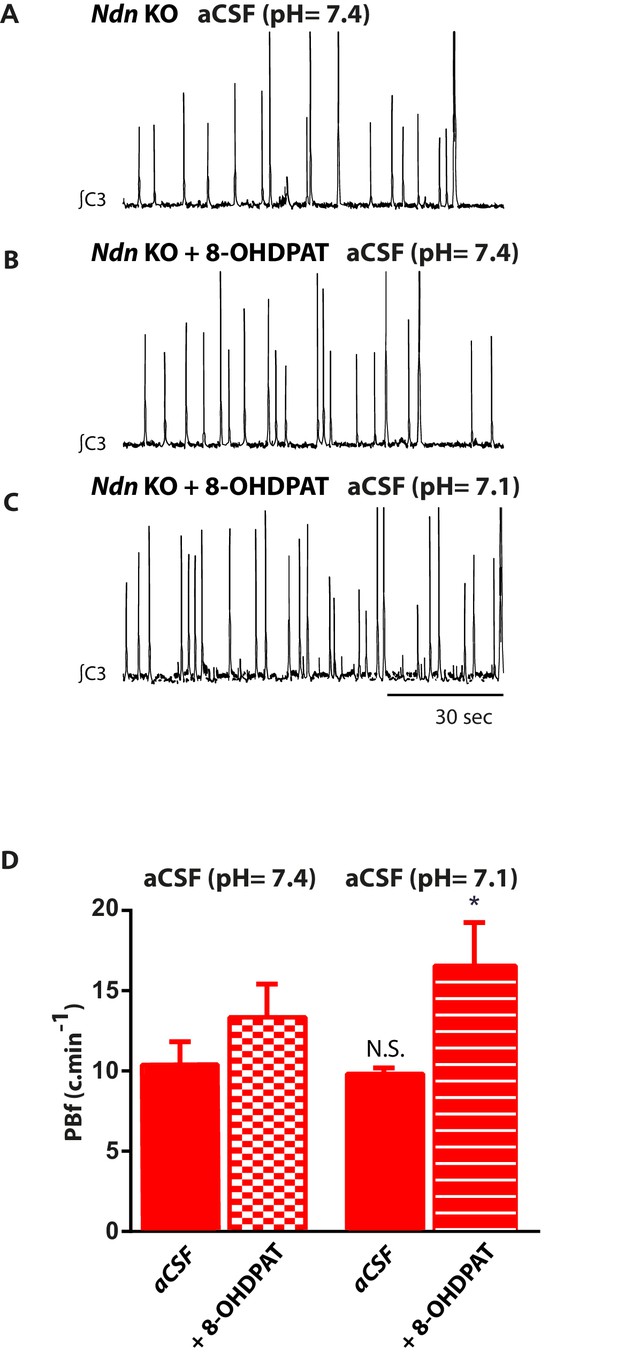
Effect of pre-treatment with the 5-HT1A-R agonist 8OHDPAT on the resting PBf and the PBf response to acidosis in Ndn-KO en bloc brainstem-spinal cord preparations of P0-P1 pups.
(A–C) PBf produced in Ndn-KO en bloc brainstem-spinal cord preparations superfused with (A) neutral aCSF (pH 7.4) and then treated with 8OHDPAT (1 µM) either (B) in neutral aCSF (pH 7.4) or (C) in acidified aCSF (pH 7.1). (D) Quantifications of the PBf (c.min−1) of en bloc brainstem-spinal cord preparations superfused with neutral aCSF (pH 7.4) and acidified aCSF (pH 7.1) of Ndn-KO (Ndn-KO (pH 7.4): 10.2 ± 1.5; Ndn-KO (pH 7.1): 9.9 ± 0.3; p=0.99, N.S.); and Ndn-KO treated with 8OHDPAT (Ndn-KO+8 OHDPAT (pH 7.4): 13.2 ± 1.9; Ndn-KO+8 OHDPAT (pH 7.1): 16.5 ± 2.6, n = 13; p=0.04). In neutral aCSF (pH 7.4), 8OHDPAT did not affect PBf in Ndn-KO preparation. However, similarly to Fluoxetine, in acidified aCSF (pH 7.1), 8OHDPAT significantly increased the PBf of Ndn-KO preparations. p-values determined by two-way ANOVA test followed by Tukey post-hoc test. Bar graphs represent mean ±SEM. N.S.: non-significant; *p<0.05
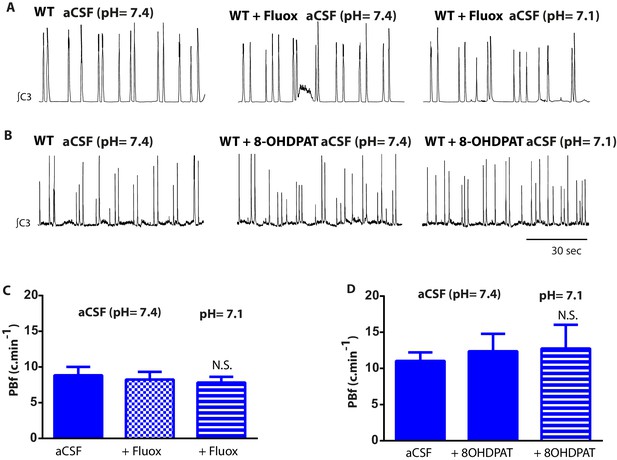
Effects of Fluoxetine and of the 5-HT1A-R agonist 8OHDPAT on the resting PBf and the PBf response to acidosis in wild-type medulla preparations.
(A–B) PBf produced in WT en bloc brainstem-spinal cord preparations superfused with (A) neutral aCSF (pH 7.4) and treated with Fluoxetine (20 μM) in aCSF (pH 7.4) or acidified aCSF (pH 7.1), (B) neutral aCSF (pH 7.4) and treated with 8OHDPAT (1 µM) in aCSF (pH 7.4) or acidified aCSF (pH 7.1). Note the absence of PBf response upon acidosis. (C–D) Quantifications of the PBf (c.min−1) of en bloc brainstem-spinal cord preparations of WT pups untreated and superfused with neutral aCSF (pH 7.4) or acidified aCSF (pH 7.1) and treated with (C) Fluoxetine (20 µM): WT (pH 7.4): 8.8 ± 1.2; WT+Fluox (pH 7.4): 8.2 ± 1.0, p>0.99, N.S.; WT+ Fluox (pH 7.1): 7.8 ± 0.7; n = 12, p>0.99, N.S.) or treated with (D) 8OHDPAT (1 µM) WT (pH 7.4): 11.0 ± 1.1; WT+8 OHDPAT (pH 7.4): 12.3 ± 2.4, p=0.76, N.S.; WT+ 8OHDPAT (pH7.1): 12.7 ± 3.3; n = 8, p=0.63, N.S. p-values determined by Friedman test followed by Dunn post-hoc test with comparison to WT. Bar graphs represent mean ±SEM. N.S.: non-significant.
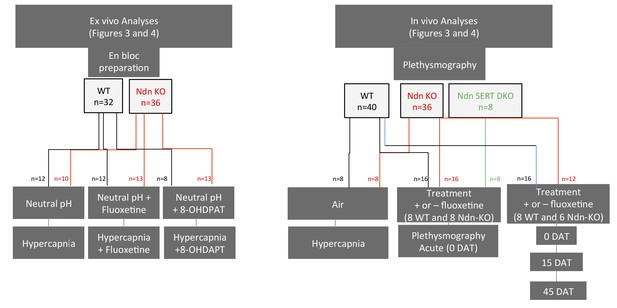
Flow diagram of mice used for ex vivo and in vivo analyses in Figures 3 and 4 and their corresponding supplement figures.
https://doi.org/10.7554/eLife.32640.017Additional files
-
Transparent reporting form
- https://doi.org/10.7554/eLife.32640.021