Network oscillation rules imposed by species-specific electrical coupling
Figures
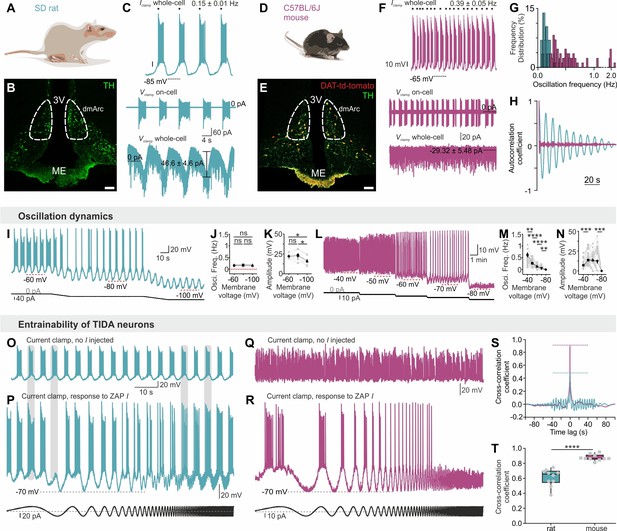
Slow, regular, fixed vs fast, irregular, entrainable activity in TIDA neurons between two rodent species.
(A–B) Distribution of TH-immunostained TIDA neurons (green) in the rat dorsomedial arcuate nucleus (dmArc; coronal section). ME, median eminence; 3V, third ventricle. (C) Patch clamp recordings from a rat TIDA neuron show a slow and robust oscillation evident in whole-cell current clamp (top), on-cell (middle) as well as voltage clamp (bottom) configurations. (D–E) Mouse TIDA neurons in the dmArc visualized by dopamine transporter (DAT)-driven tdTomato fluorescence (red) and TH immunostaining (green). (F) Whole-cell current clamp recording (top) from a mouse TIDA neuron shows fast and irregular phasic firing, also evident in on-cell configuration (middle), whereas no oscillatory current is seen voltage-clamp mode (bottom). (G) Frequency distribution of the oscillation frequency in rat (blue) vs mouse (purple) TIDA neurons, with a narrow range of slow frequencies in rat and a wide range of faster oscillation frequencies in mouse (n = 29–115 per group). (H), Representative autocorrelograms of rat and mouse TIDA oscillation. (I) Whole-cell current clamp recording: Progressive hyperpolarization fails to alter oscillation frequency in rat TIDA cells. (J, K,) Quantification of rat TIDA oscillation frequency (J) and amplitude (K) at different voltages (−60 mV, −80 mV and −100 mV; n = 7, one-way ANOVA with Bonferroni as post-hoc test). (L) Progressive hyperpolarization (as in I) gradually slows oscillation frequency in mouse TIDA cells, with ultimate collapse of the oscillation below −80 mV. (M, N) Quantification of mouse TIDA oscillation frequency (M) and amplitude (N) at different voltages (n = 34, one-way ANOVA with Bonferroni as post-hoc test). (O–T) Test for entrainability of TIDA neurons in rat vs mouse arcuate slices during whole-cell current clamp recording. (O) Rat TIDA neuron immediately prior to ZAP current (I; sinusoidal current of gradually increasing frequency) injection presented in (P). (P) Bottom trace; injection of ZAP I does not change the rat TIDA neuron’s oscillation frequency. (Q) Mouse TIDA neuron immediately prior to ZAP I injection presented in (R). (R) Injection of ZAP I entrains mouse TIDA neurons. (S) Cross correlation coefficient example and T) quantification between the injected ZAP I and the membrane voltage response in the two species (n = 10 per group, p<0.0001, unpaired t-test; rat shown in blue, mouse shown in purple). Data expressed as mean ±s.e.m.
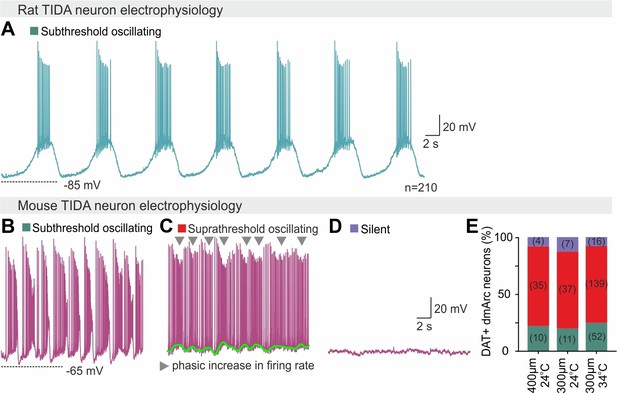
Homogeneous vs heterogeneous electrophysiological activity in rat vs mouse TIDA neurons.
(A) Representative whole-cell current clamp recording of a TIDA neuron from a hypothalamic rat brain slice. (B–D) Representative whole-cell current clamp recordings of three mouse TIDA neurons, illustrate the wide spectrum of electrical activity (subthreshold oscillating, suprathreshold oscillating and silent) found in the murine TIDA population. (E) Quantification of the percentage of neurons found at the three different states shown in (b–d) under different recording conditions. Note that the proportions remain the same regardless of network volume (slice thickness, 300 or 400 μm) or temperature (24°C or 34°C).
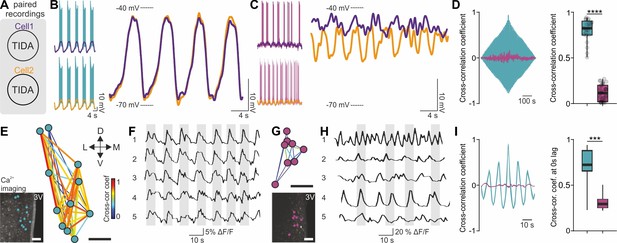
Synchronous vs asynchronous activity in TIDA neurons between two rodent species.
(A) Paired whole-cell recordings were performed to test synchronous activity between cells. (B) Representative paired rat TIDA neuron recordings (left) shown superimposed and filtered for action potentials at right to reveal near identical membrane voltage fluctuations. (C) Representative paired mouse TIDA neuron recordings (left) reveal asynchronicity when superimposed (right). (D) Left; cross-correlation coefficient in example paired recordings from rat (blue) and mouse (purple) TIDA neurons. Right; quantification of cross-correlation coefficient at 0 s lag (n = 21–31 respectively, p<0.0001, unpaired t-test). (E) Ca2+ imaging of rat TIDA neurons. Lower inset: oscillating cells in the dmArc slice shown highly connected by relative cross-correlation. (F) Representative Ca2+ imaging traces of five rat TIDA neurons in same slice revealing synchronous oscillations. (G) Ca2+ imaging of mouse TIDA neurons. Lower inset: Cells in the dmArc in the same slice exhibit minimal cross-correlation. (H) Representative Ca2+ imaging traces of five mouse TIDA neurons in same slice revealing asynchronous oscillation. (I) Left; cross-correlation coefficient in rat (blue) Ca2+ imaging traces vs cross-correlation coefficient in mouse (purple) TIDA neurons. Right; quantification of cross correlation coefficient at 0 s lag (n = 14–21 respectively). Data expressed as mean ±s.e.m. Image scale bars (E G) 50 μm. 3V, third ventricle.
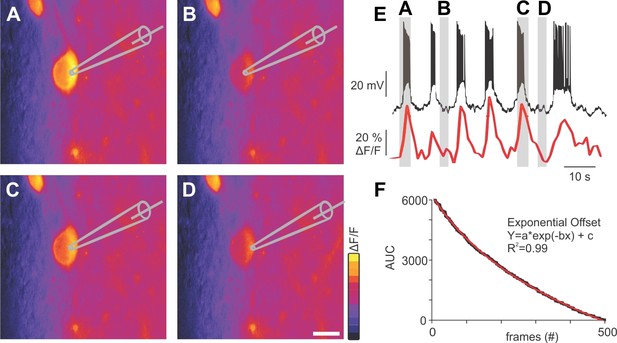
Ca2+oscillations correlate highly with membrane voltage oscillations in TIDA neurons.
(A-D) Calcium images from a single TIDA neuron in different time points. Dark colours represent low-calcium activity, brighter colors represent high-calcium activity in the cell, scale bar is 10 µm. (E) Simultaneous calcium imaging and whole-cell in DAT-GCaMP3 mouse TIDA neuron. Shadow areas refer to the previous images at a peak of florescence (A and C, high-calcium activity) and low fluorescence (B and D, low-calcium activity). (F) Bleach correction by exponential frame subtraction performed with Image J EMBL tools.
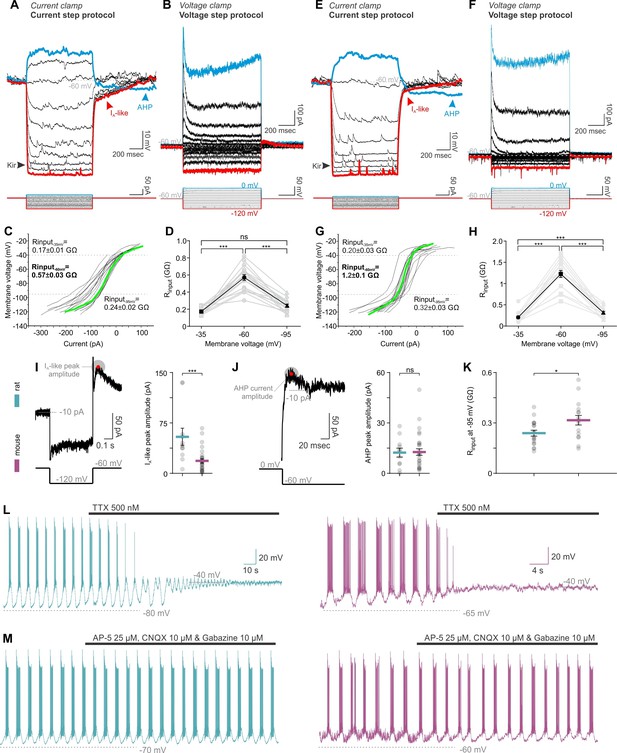
TIDA neurons have similar membrane properties, and exhibit similar sensitivity to tetrodotoxin (TTX) and synaptic blockade in both rats and mice.
(A,E) Current and (B,F) voltage step protocols identify characteristic rat (A–D) and mouse (E–H) TIDA cell conductances, namely the K+-mediated inward rectification (Kir), an A-like current (IA) and the depolarization-induced after hyperpolarization (AHP). (,G) Input resistance of TIDA neurons across membrane voltage reveals a sigmoidal plot in both species. (D,H) Higher input resistance at −60 mV in both rat (n = 18) and mouse (n = 16) TIDA neurons (one-way ANOVA with Bonferroni as post-hoc test). (I) Quantification of the A-like current and (J) the AHP current (n = 11 and 30 per group). (K) Comparison of the Rinput at −95 mV, as an indicator of the Kir current activity. (L) Application of the antagonist of voltage-gated Na+ channels, TTX, abolished the oscillation and switched it to a persistent UP state in rat (blue; n = 32) as well as in mouse (purple; n = 22) TIDA neurons. (M) Oscillation persists in both rat (n = 11) and mouse (n = 6) TIDA neurons under fast ionotropic blockade, using AMPA and NMDA glutamate receptor blockers combined with Gabazine as a GABAA receptor blocker. (A–D), rat and (E–H), mouse.
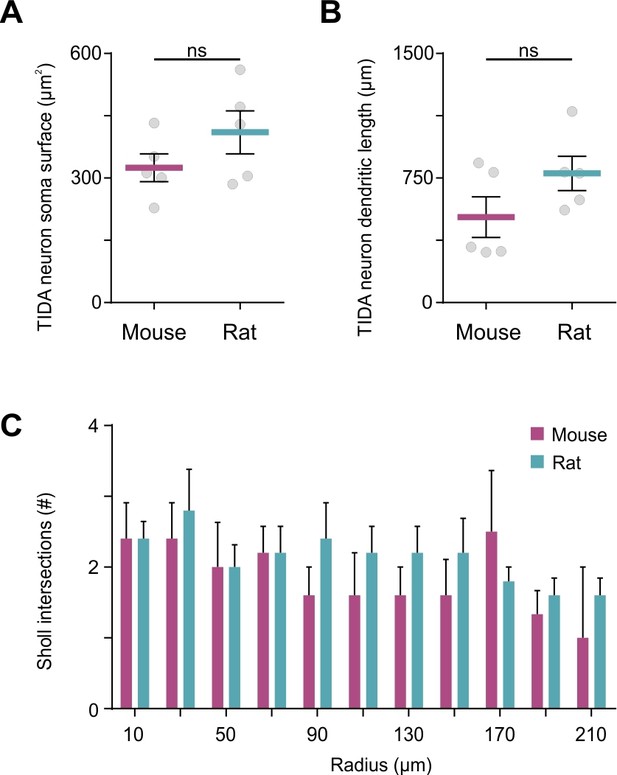
Comparison of TIDA neuron cytoarchitecture between rat and mouse.
(A–C) Cell soma size (A), total dendrite length (B) and dendrite branching distribution (C) in TIDA neuron is statistically similar between the two species (n = 5 cells per group).
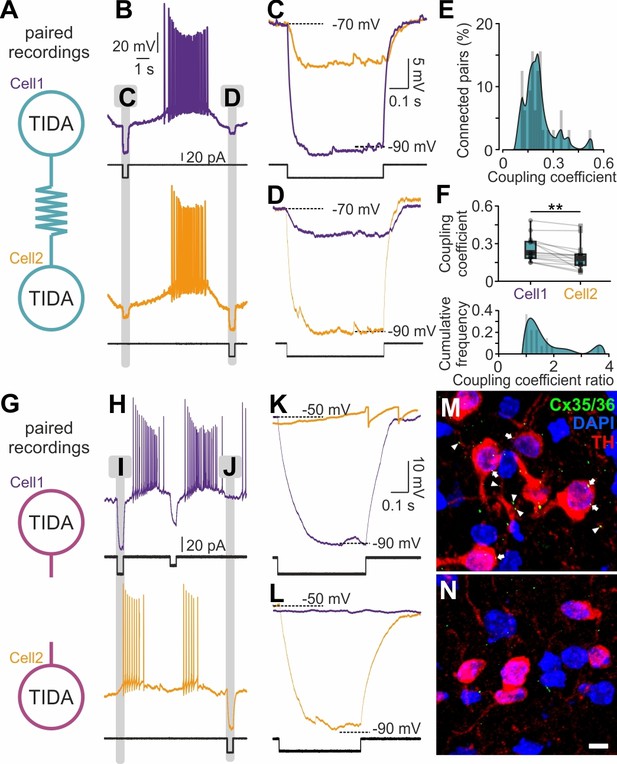
Electrical synapses in the rat but not in the mouse TIDA network.
(A) Paired TIDA neuron recordings were performed to test for reciprocal electrical connectivity in rat slices. (B) Representative recording showing bidirectional electrical coupling in rat slices. Example trace illustrating the effect of negative current injection first into cell one and then into cell 2. (C,D,) High-resolution trace during negative current injection. Note the different response amplitude in cell1 vs cell2, suggestive of asymmetrical electrical synapses. (E) Frequency distribution of the coupling coefficient, average 0.18 ± 0.02, n = 32 pairs. (F) Top; Different coupling coefficient between cell 1 and cell 2, indicative of asymmetrical electrical synapses (n = 16, p<0.01, unpaired t-test). Bottom; Cumulative frequency of the coupling coefficient ratio, indicative of the high-degree of functional coupling asymmetry. (G) Similar to (A) paired recordings were performed in mouse TIDA neurons. (H) Representative recording showing the absence of electrical coupling in mouse slices. Example trace illustrating the effect of negative current injection first into cell 1 and then into cell 2. (I) and J) indicate portions of recordings illustrated at higher resolution in (K) and (L), respectively. (K, L,) High-resolution traces illustrating lack of electrical coupling between mouse TIDA neurons (n = 40 pairs). (M, N,) Cx35/36 immunostaining (green) of the dmArc shows punctate presumed gap junctions (arrows) on the soma and dendrites of rat (M), but not mouse (N), TIDA neurons. TH (red) used to identify TIDA neurons, DAPI (blue) shows cell nuclei. Scale bars for (M N) 10 µm.
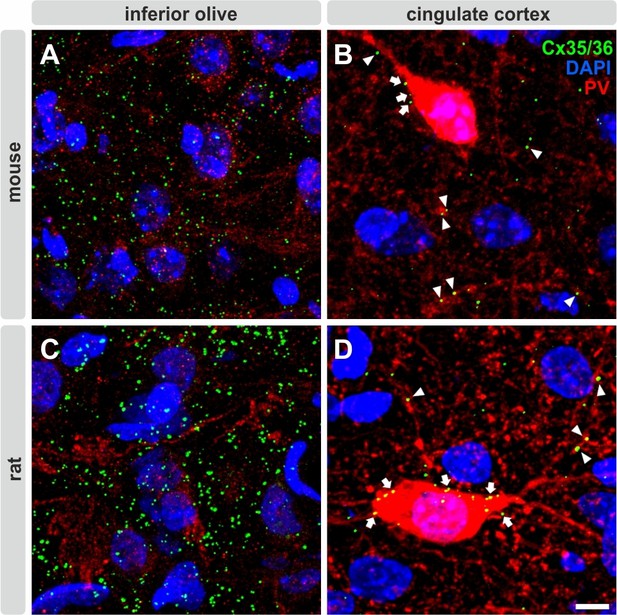
Cx35/36 immunostaining in the inferior olive and cingulate cortex in rat and mouse.
Coronal maximum intensity projections of high power confocal micrographs from C57BL/6 mice (A B) and SD rats (C D) stained for parvalbumin (PV; red), connexin-36 (Cx36; green) and DAPI (blue). Note high density of Cx36- immunoreactive (-ir) puncta in the inferior olive (A C) as well as Cx36-ir puncta in close proximity to PV-ir cell somata and dendrites in the cingulate cortex in both mice (B) and rats (D). Scale bar in (D) = 5 µm for (A–D).
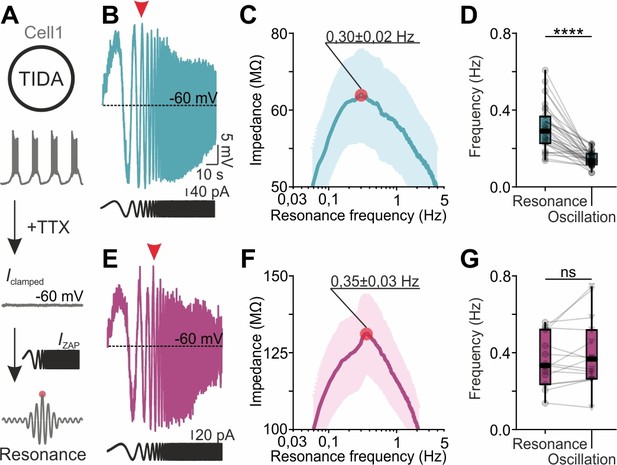
TIDA resonance frequency matches oscillation frequency in the electrically uncoupled, but not in the coupled, network.
(A) Schematic illustration of the current clamp protocol used to determine the resonance frequency of TIDA neurons. (B) Voltage response (blue) of a rat TIDA neuron to a 100 s 0.05–10 Hz ZAP current injection (black trace below) yields a maximum amplitude at the neuron’s preferred resonance frequency (red arrow). (C) Impedance profile of rat TIDA neurons in the frequency domain. The red circle indicates the resonance frequency at the maximum impedance value (0.30 ± 0.02 Hz, n = 32). (D) Quantification of resonance frequency vs oscillation frequency in rat TIDA neurons (n = 32, p<0.0001, paired t-test). (E) Recording of mouse preferred resonance frequency as in (B). (F) Impedance profile of mouse TIDA neurons in the frequency domain. The red circle indicates the resonance frequency at the maximum impedance value (0.35 ± 0.03 Hz, n = 15). (G) Resonance frequency vs oscillation frequency in mouse TIDA neurons (n = 15, p>0.05, paired t-test).
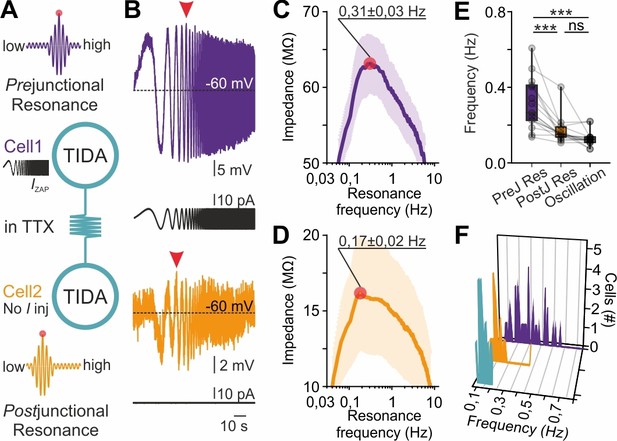
Gap junction resonance frequency dictates network frequency of coupled heterogeneous TIDA neurons.
(A) Schematic of the current clamp protocol used to record the transfer of a ZAP function command through electrical synapses by measurements of resonance frequency in coupled TIDA neurons. (B) Paired recording of electrically connected rat TIDA neurons. Voltage response of Cell 1 (purple; prejunctional) to the 100 s ZAP current injection that sweeps a 0.05–10 Hz frequency (black trace) range, yields a maximum amplitude at a preferred resonance frequency. Cell 2 membrane voltage response (orange; postjunctional) indicates maximum amplitude at a different resonance frequency. (C) The impedance profile of the prejunctional resonance in the frequency domain. The red circle indicates the resonance frequency at the maximum impedance value (0.31 ± 0.03 Hz; n = 16). (D) Postjunctional resonance impedance profile; note lower average resonance frequency (red circle; 0.17 ± 0.02 Hz; n = 16). (E) Comparison of pre- and post-junctional resonance frequency vs oscillation frequency (n = 16, one-way ANOVA with Bonferroni as post-hoc test). (F) Frequency distribution of pre- (purple) and post-junctional (orange) resonance frequency vs oscillation frequency (turquoise) (n = 16 per group).
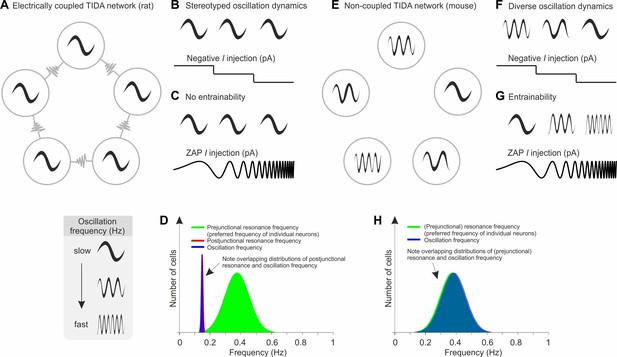
Schematic model based on the experimental data from TIDA neurons of the influence of electrical synapses on resonance and the emergence of a slow frequency oscillation in a neuronal population.
(A) In the electrically coupled network (as observed in rat TIDA neurons), neurons exhibit a robust, stereotyped, slow, synchronized oscillation. In this network, frequency is immune to perturbation and cannot be manipulated by progressive hyperpolarization (B) or by ZAP current injection (C). (D) The prejunctional resonance of individual neurons does not dictate the network oscillation frequency; rather, the gap junction-mediated postjunctional resonance commands the oscillation frequency of the syncytium. (E) In the uncoupled network configuration (as observed in mouse TIDA neurons), neurons exhibit a diverse range of membrane potential oscillations. Their phasic voltage fluctuations are strongly sensitive to progressive hyperpolarization (F) and are highly entrainable, as tested via ZAP current injections (G). (H) The fast prejunctional resonance of individual neurons matches their oscillation frequency, allowing them to march to the beat of their own intrinsic properties.
Tables
Reagent type (species) or resource | Designation | Source of reference | Identifiers | Additional information |
---|---|---|---|---|
Mouse strain, strain background | DAT-Cre mouse (C57Bl/6J) | Reference: PMID 17227870. | Gift from Dr. N-G Larsson, Karolinska Institutet. | |
Mouse strain, strain background | tdTomato floxed mouse (C57Bl/6J) | www.jax.org/strain/007905 | The Jackson Laboratory | |
Mouse strain, strain background | GCaMP3 floxed mouse (C57Bl/6J) | www.jax.org/strain/014538 | The Jackson Laboratory | |
Antibody | anti-tyrosine hydroxylase | Source: Millipore. Reference: PMID 25794171 | AB152 | |
Antibody | anti-connexin 35/36 | Source: Millipore. Reference: PMID 26760208. | MAB3045 | |
Software, algorithm | MATLAB code for network analysis | Source: PMID 25278844 |
Additional files
-
Source data 1
Oscillation dynamics in rat TIDA neurons.
Injection of negative current in current clamp results in hyperpolarization of the subthreshold oscillation. Note that the oscillation frequency remains unchanged irrespective of membrane voltage.
- https://doi.org/10.7554/eLife.33144.013
-
Source data 2
Oscillation dynamics in mouse TIDA neurons:Injection of negative current in current clamp, results in a switch from continuous firing to oscillatory discharge.
Note that upon further negative current injection, the oscillation collapses at −80 mV.
- https://doi.org/10.7554/eLife.33144.014
-
Transparent reporting form
- https://doi.org/10.7554/eLife.33144.015