Comment on “Evolutionary transitions between beneficial and phytopathogenic Rhodococcus challenge disease management”
Abstract
We would like to address a number of concerns regarding this paper (Savory et al., 2017)
https://doi.org/10.7554/eLife.35272.001Plant growth promotion by the Rhodococcus pistachio bushy top syndrome (PBTS) isolates
While the analysis by Savory et al. (2017) of Rhodococcus fascians isolates that are found to cause leafy gall disease on ornamental plants is noteworthy, the data presented do not unambiguously demonstrate plant growth promotion by PBTS Rhodococcus isolates. In contrast, the data presented in Savory et al. (2017) (Figure 7B and Figure 7—figure supplement 1) indicate that, upon inoculation, the Nicotiana benthamiana roots were stunted in comparison to the roots of control plants. PBTS Rhodococcus 2 caused significant root stunting at an inoculum level of only 2.5 × 106 CFU (Figure 7B), 103 fold lower than the standard dose used throughout the paper. In orchard systems, inadequate root development affects tree anchorage in soils and influences aboveground tree and canopy development. For these reasons, the data presented by Savory et al. do not support the assertion that PBTS Rhodococcus isolates are plant growth promoting.
Potential misdiagnosis of an epidemic
Savory et al. claim to have ‘uncovered a misdiagnosed epidemic’ that has ‘perpetuated the unnecessary removal of trees and has exacerbated economic losses in the pistachio industry’. The trees that exhibited Pistachio Bushy Top Syndrome (PBTS) were removed due to their irregular growth and development, and their high percentage of grafting failure (>70%; Stamler et al., 2015a). Those rootstocks that did successfully accept a scion bud developed bark cracking along the bud/graft union that weakened the tree. Pistachio ranch owners and managers of affected orchards concluded that tree removal was the only economically feasible option well before Stamler et al., 2015a was published. An ongoing study has demonstrated that PBTS trees entering maturity exhibit a 73% reduction in yield and lower nut quality in comparison to asymptomatic trees of the same age in the same orchard (Fichtner et al., 2018). Ranch managers opted for the more expensive hand-harvest, as opposed to mechanical harvest of symptomatic trees, due to the risk of breakage at the cracked graft union. Three of the authors on Stamler et al., 2015a are pistachio horticulturists with close connections to the pistachio industry. We are personal witnesses to the fact that the statements regarding tree removal in California, Arizona and New Mexico are inaccurate (Savory et al., 2017). The majority of PBTS symptomatic trees were removed from orchards a year before Stamler et al., 2015a was published (Northcutt, 2015; Fichtner et al., 2015). Hence, the claim that the trees were removed due to a misdiagnosis is erroneous, unjustly discredits the research team involved in the diagnosis, and insults the pistachio growers who decided to remove trees.
Presence of virulence genes
Savory et al. state that 'contrary to previous findings, there is no evidence to support the diagnosis that Rhodococcus without a virulence plasmid are responsible for an unusual growth problem that has plagued the pistachio industry.’ It was never claimed that PBTS was caused by Rhodococcus isolates lacking virulence genes (Stamler et al., 2015a). On the contrary, the isolates utilized for establishing Koch’s postulates were shown to have virulence genes (fasD and fasA) using PCR amplification and direct sequencing (Stamler et al., 2015a). Since previous work on R. fascians isolates from ornamental plants has shown that the presence of virulence genes is required for pathogenicity (Desomer et al., 1988; Crespi et al., 1992; Eason et al., 1995; Eason et al., 1996; Stange et al., 1996; Nikolaeva et al., 2009), we agree that PBTS isolates without these described virulence genes are likely non-pathogenic.
Subpopulations and loss of detectable virulence genes in PBTS Rhodococcus isolates when culturing on synthetic media
Savory et al. use a ‘Genome Announcement’ (Stamler et al., 2016) as evidence for the lack of virulence genes in PBTS1 and PBTS2, although the title of the cited paper clearly stated that the information provided concerned draft sequences of PBTS1 and PBTS2, thus recognizing that not all of the genetic information was obtained (i.e., fasD, fasA, and attA). Our unpublished work has shown that these specific Rhodococcus isolates obtained from UCB-1 pistachio trees and grown on synthetic media contain a subpopulation of bacterial cells with virulence genes (fasD and fasA). After subculturing the bacteria only twice on synthetic media, these virulence genes become undetectable by PCR. Thus, when sequencing these subcultured isolates using the PacBio platform, the virulence genes (originally detected using PCR amplification) were no longer detected (Stamler et al., 2016). Plasmid instability and loss of virulence are recognized in several bacterial species and were also described for leafy gall-inducing R. fascians isolates. Sabart et al., 1986 demonstrated that R. fascians (previously named Corynebacterium fascians) lost virulence within several generations due to growing conditions, and Nikolaeva et al., 2009 showed instability of virulence due to plasmid loss in certain R. fascians isolates.
High plasmid instability in R. fascians
Savory et al. state that Nikolaeva et al., 2009 used the wrong molecular tools to evaluate pathogenicity, but these authors showed that for some isolates, individual fas positive colonies gave rise to fas positive and fas negative bacteria, very effectively ruling out the possibility that these findings were caused by genetically different lineages.
Poor growth of the control pistachio UCB-1 trees
The work by Savory et al. indicates that R. fascians and PBTS Rhodococcus 1 and 2 were inoculated on UCB-1 seedlings and no effects were observed (Figure 7—figure supplement 1; panel E). Savory et al. provided no details in regards to the germination, size of seedlings, or growth conditions. In our work, micropropagated, clonal UCB-1 plants were used to mimic the propagation type of affected PBTS trees in the field while minimizing the potential influence of host variability on symptom expression (Stamler et al., 2015a). Based on the data provided in Savory et al. (Figure 7—figure supplement 1; panel E), all of the UCB-1 pistachio seedlings surprisingly exhibited little to no growth after 60 days. In contrast, the mock-inoculated clonal trees used by Stamler et al., 2015a continued to grow rapidly for more than 100 days, and it was nearly 80 days post-inoculation before statistically significant differences in height were observed between inoculated and mock-inoculated trees (Figure 1). The poor growth of mock-inoculated UCB-1 seedlings (Savory et al., 2017) suggests that experimental conditions were not conducive to healthy pistachio growth, thus rendering the control trees unsuitable as a basis of comparison.
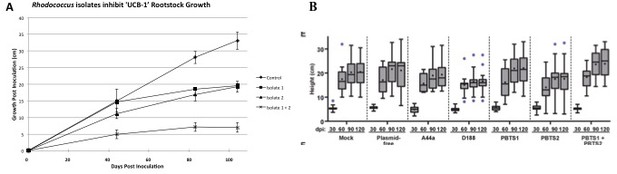
Comparison of pistachio ‘UCB-1’ clonal tree assays versus ‘UCB-1’ seedling tree assays.
(A) Clonal UCB-1 trees, transitioned out of tissue culture eight weeks prior to inoculation with Rhodococcus isolates 1 and 2. Four treatments were performed with 16 plants per treatment for each trial. The control plants were mock-inoculated with buffer. Three inoculated treatments included bacterial suspensions of Rhodococcus isolate 1, Rhodococcus isolate 2, and both Rhodococcus isolate 1 and isolate 2. Growth curves showing height of UCB-1 plants at multiple time points after inoculation. Error bars are standard error (n = 16). (B) Seedling UCB-1 trees inoculated with Rhodococcus. Reprinted from Savory et al., 2017. eLife 6:e30925.
© 2015, The American Phytopathological Society. Reprinted from: Stamler et al., 2015a, First report of Rhodococcus isolates causing Pistachio Bushy Top Syndrome on ‘UCB-1’ rootstock in California and Arizona. Plant Disease 99:1468-1476.
Misrepresentation of previous work
‘There was a targeted search for Rhodococcus’ (referring to Stamler et al., 2015a, 2015b). This is not true, as many potential causative agents were evaluated.
‘Bacteria were inexplicably cultured from asymptomatic leaves distal to symptomatic tissues’. If Savory et al. are implying that R. fascians can only be cultured from symptomatic tissues, this has been known for decades (Lacey, 1939; Cornelis et al., 2001).
‘Some of the results of the molecular detection were clearly artifacts,’ referring to Stamler et al., 2015a. Savory et al. support their statement by stating that the published fasD fragments are identical in sequence, yet they fail to mention that the fasD gene over its entire length is identical among most of the ornamental isolates (Creason et al., 2014).
By stating, ‘We recognize the insurmountable challenge in showing that there is no possibility that pathogenic Rhodococcus causes pistachio bushy top syndrome,’ Savory et al. admit to the fact that their data did not show a misdiagnosis. We remain confident in our published results.
References
-
The plant pathogen Rhodococcus fascians colonizes the exterior and interior of the aerial parts of plantsMolecular Plant-Microbe Interactions 14:599–608.https://doi.org/10.1094/MPMI.2001.14.5.599
-
Fasciation induction by the phytopathogen Rhodococcus fascians depends upon a linear plasmid encoding a cytokinin synthase geneEMBO Journal 11:795–804.
-
Conjugative transfer of cadmium resistance plasmids in Rhodococcus fascians strainsJournal of Bacteriology 170:2401–2405.https://doi.org/10.1128/jb.170.5.2401-2405.1988
-
Virulence assessment of Rhodococcus fascians strains on pea cultivarsPlant Pathology 44:141–147.
-
Avirulent isolates of Corynebacterium fascians that are unable to utilize agmatine and proline.Applied and Environmental Microbiology 52:33–36.
Article and author information
Author details
Funding
The funders had no role in study design, data collection and interpretation, or the decision to submit the work for publication.
Copyright
© 2018, Randall et al.
This article is distributed under the terms of the Creative Commons Attribution License, which permits unrestricted use and redistribution provided that the original author and source are credited.
Metrics
-
- 892
- views
-
- 61
- downloads
-
- 7
- citations
Views, downloads and citations are aggregated across all versions of this paper published by eLife.
Download links
Downloads (link to download the article as PDF)
Open citations (links to open the citations from this article in various online reference manager services)
Cite this article (links to download the citations from this article in formats compatible with various reference manager tools)
Further reading
-
- Plant Biology
Obligate parasites often trigger significant changes in their hosts to facilitate transmission to new hosts. The molecular mechanisms behind these extended phenotypes - where genetic information of one organism is manifested as traits in another - remain largely unclear. This study explores the role of the virulence protein SAP54, produced by parasitic phytoplasmas, in attracting leafhopper vectors. SAP54 is responsible for the induction of leaf-like flowers in phytoplasma-infected plants. However, we previously demonstrated that the insects were attracted to leaves and the leaf-like flowers were not required. Here, we made the surprising discovery that leaf exposure to leafhopper males is required for the attraction phenotype, suggesting a leaf response that distinguishes leafhopper sex in the presence of SAP54. In contrast, this phytoplasma effector alongside leafhopper females discourages further female colonization. We demonstrate that SAP54 effectively suppresses biotic stress response pathways in leaves exposed to the males. Critically, the host plant MADS-box transcription factor short vegetative phase (SVP) emerges as a key element in the female leafhopper preference for plants exposed to males, with SAP54 promoting the degradation of SVP. This preference extends to female colonization of male-exposed svp null mutant plants over those not exposed to males. Our research underscores the dual role of the phytoplasma effector SAP54 in host development alteration and vector attraction - integral to the phytoplasma life cycle. Importantly, we clarify how SAP54, by targeting SVP, heightens leaf vulnerability to leafhopper males, thus facilitating female attraction and subsequent plant colonization by the insects. SAP54 essentially acts as a molecular ‘matchmaker’, helping male leafhoppers more easily locate mates by degrading SVP-containing complexes in leaves. This study not only provides insights into the long reach of single parasite genes in extended phenotypes, but also opens avenues for understanding how transcription factors that regulate plant developmental processes intersect with and influence plant-insect interactions.
-
- Microbiology and Infectious Disease
- Plant Biology
Programmed cell death occurring during plant development (dPCD) is a fundamental process integral for plant growth and reproduction. Here, we investigate the connection between developmentally controlled PCD and fungal accommodation in Arabidopsis thaliana roots, focusing on the root cap-specific transcription factor ANAC033/SOMBRERO (SMB) and the senescence-associated nuclease BFN1. Mutations of both dPCD regulators increase colonization by the beneficial fungus Serendipita indica, primarily in the differentiation zone. smb-3 mutants additionally exhibit hypercolonization around the meristematic zone and a delay of S. indica-induced root-growth promotion. This demonstrates that root cap dPCD and rapid post-mortem clearance of cellular corpses represent a physical defense mechanism restricting microbial invasion of the root. Additionally, reporter lines and transcriptional analysis revealed that BFN1 expression is downregulated during S. indica colonization in mature root epidermal cells, suggesting a transcriptional control mechanism that facilitates the accommodation of beneficial microbes in the roots.