Strong biomechanical relationships bias the tempo and mode of morphological evolution
Figures
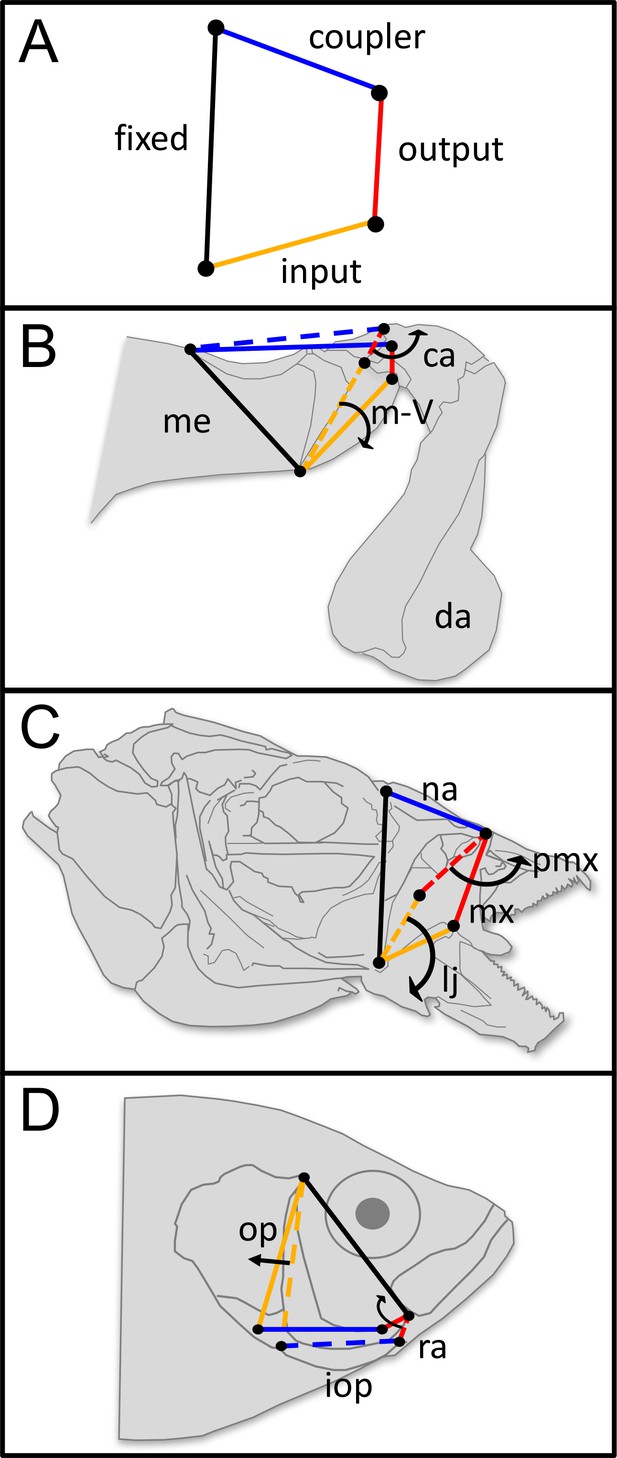
Four-bar linkage systems have evolved independently multiple times across animals and are comprised of four rotatable links that transmit motion and force.
(A) Four-bar linkages consist of a fixed link (black) and three mobile links: input (orange), output (red), and coupler (blue). (B) In the raptorial appendages of mantis shrimp (Stomatopoda), rotation of the input link (meral-V, m-V, which is part of the merus segment, me) causes the output link (carpus, ca) to rotate outward, which then rapidly rotates the dactyl (da). (C) In the oral four-bar system that evolved independently in labrid and cichlid fishes, the input link (lower jaw, lj) rotates ventrally, causing rotation in the nasal (na) and in the output link (maxilla, mx), resulting in premaxillary (pmx) protrusion. (D) In the opercular four-bar linkage system of centrarchid fish, the input link (opercle and subopercle, op) swings posteriorly, as does the interopercle (iop). This motion is transmitted to the output link (retroarticular process in the mandible, ra), which causes the mandible to rotate ventrally and open the lower jaw. (B–D) Dashed lines denote the closed configuration of input (orange), output (red), and coupler (blue) links, whereas solid lines denote their open configuration following motion. Arrows denote the direction of motion. Distal/anterior is to the right and dorsal is toward the top of the page.
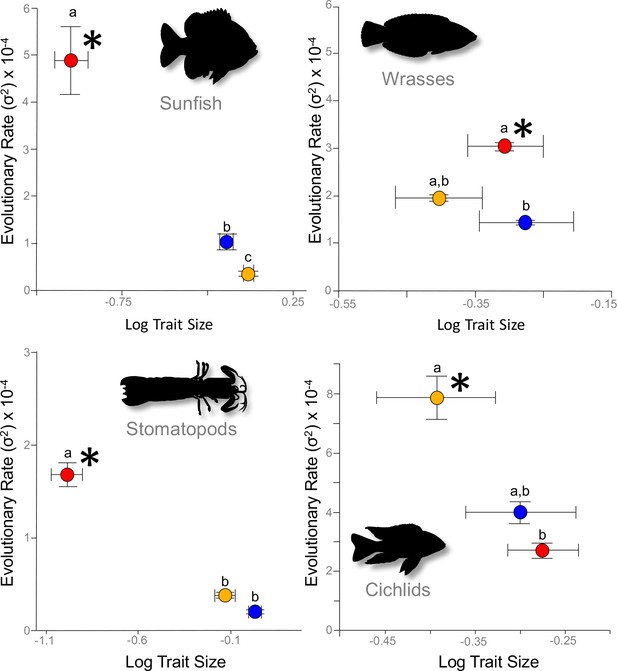
Across the four focal systems, evolutionary rate is consistently faster in the links to which the mechanical output is most mechanically sensitive (asterisks).
The evolutionary rate parameter, σ2 (± 95% confidence interval), is depicted for each link in each system. Orange circles denote the input link, red circles denote the output link, and blue circles denote the coupler link. Shared letters denote rates that are not statistically different from each other (statistical results are in Table 1).
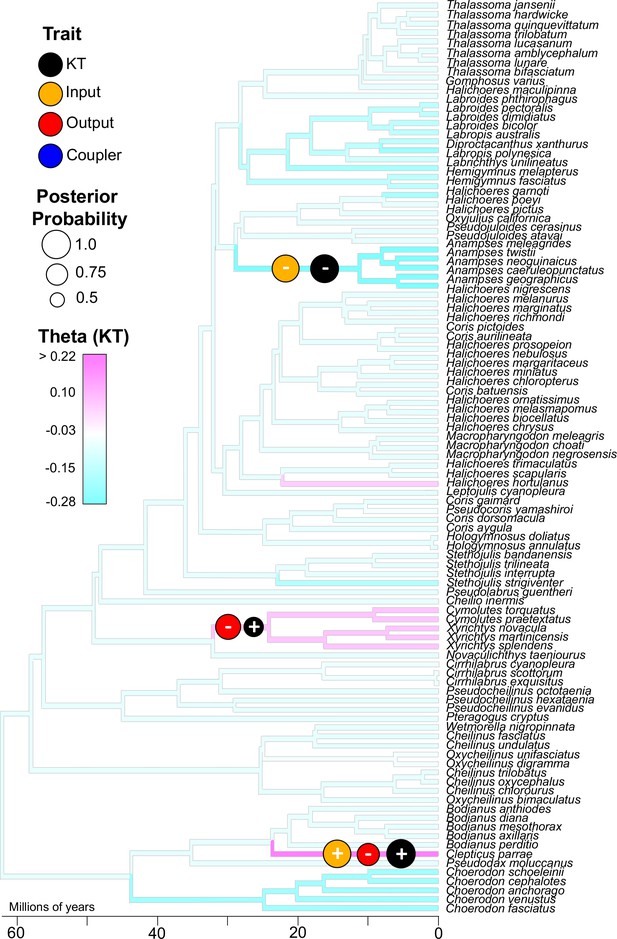
Three well-supported transitions in KT (black circles) occurred across the phylogeny of wrasses.
With each of these transitions in KT, either the input (orange circle) or output link (red circle) also experienced a strongly-supported shift in magnitude (sign indicates directionality of trait shift). The coupler link (blue circle) did not exhibit a strongly-supported shift. These analyses were performed using reversible-jump MCMC which detected significant shifts (optimal trait value, θ) based on the distribution of traits across the phylogeny (KT trait distribution is overlaid as a color map on the tree branches; see Figure 3—figure supplement 1 for color maps of the other trait distributions). The sizes of the circles represent posterior probability (threshold posterior probability for a strongly supported transition was set at > 0.5.
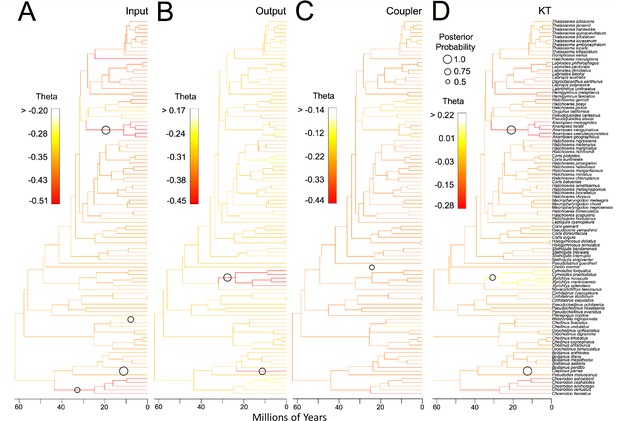
Results of reversible-jump MCMC analysis of evolutionary trait shifts for the input link (A), output link (B), coupler link (C), and KT (D).
Color in each phylogeny denotes trait values with the range of values (theta) for each trait given in each legend. Circles denote branches where shifts in trait values were supported (posterior probability >0.5). Circle size denotes posterior probability of trait shifts.
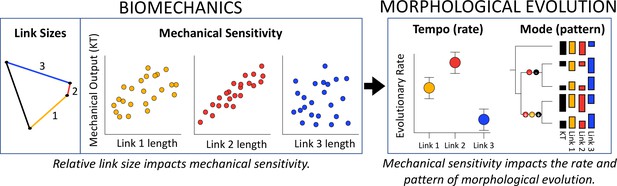
The three conceptual frameworks of many-to-one mapping, mechanical sensitivity and constraints converge at the intersection of biomechanics and morphological evolution, highlighting the importance of analyzing these systems at multiple levels.
Many-to-one mapping (multiple configurations yield similar mechanical outputs) can occur in any linkage system, yet mechanical sensitivity defines the tight correlations between link size and kinematic transmission (KT). From a biomechanical perspective, mechanical sensitivity occurs because length changes in short links have disproportionately large effects on KT. From an evolutionary perspective, rate of evolutionary change (tempo) is accelerated in the links to which KT is most mechanically sensitive. Many-to-one mapping again emerges from analyses of evolutionary mode (pattern), in which statistically significant shifts in link length and KT across the topology of the phylogeny can occur through multiple configurations, yet only in the links to which the system is most mechanically sensitive.
Tables
Phylogenetic Generalized Least Squares (PGLS) regressions reveal that the relationship between KT and link size (mechanical sensitivity) varies among four-bar linkage systems.
In each analysis, the mobile links are predictor variables and kinematic transmission is the response variable.
Cichlids (df = 28) | |||||
---|---|---|---|---|---|
Predictor | Coeff.±SE | T | P | r2 | AIC |
Input | 0.63 ± 0.09 | 7.08 | 1.1e-7 | 0.623 | −119.22 |
Output | −0.23 ± 0.24 | −0.96 | 0.343 | 0.002 | −89.77 |
Coupler | −0.46 ± 0.14 | −3.42 | 0.002 | 0.270 | −98.95 |
Mantis shrimp (df = 34) | |||||
---|---|---|---|---|---|
Predictor | Coeff.±SE | T | P | r2 | AIC |
Input | 0.20 ± 0.32 | 0.64 | 0.527 | 0.017 | −90.86 |
Output | −0.77 ± 0.08 | −9.75 | 2.3e-11 | 0.729 | −138.34 |
Coupler | 0.03 ± 0.39 | 0.07 | 0.948 | 0.029 | −90.48 |
Sunfish (df = 17) | |||||
---|---|---|---|---|---|
Predictor | Coeff.±SE | T | P | r2 | AIC |
Input | 3.02 ± 0.68 | 4.48 | 3.3e-4 | 0.514 | −44.72 |
Output | −1.18 ± 0.04 | −26.56 | 2.7e-15 | 0.975 | −97.92 |
Coupler | −1.00 ± 0.63 | −1.58 | 0.133 | 0.133 | −32.48 |
Wrasses (df = 99) | |||||
---|---|---|---|---|---|
Predictor | Coeff.±SE | T | P | r2 | AIC |
Input | 1.00 ± 0.13 | 7.45 | 3.5e-11 | 0.353 | −240.36 |
Output | −1.47 ± 0.10 | −14.15 | 2.2e-16 | 0.666 | −306.55 |
Coupler | 0.39 ± 0.18 | 2.25 | 0.027 | 0.039 | −201.14 |
Additional files
-
Supplementary file 1
Pair-wise comparisons of evolutionary rate.
For each trait (KT, input link, output link, and coupler link), the Brownian Motion evolutionary rate parameter (σ2) is given. For each comparison, the AICC score for a model in which rates are allowed to vary (obs.) and constrained to be equal (const.) are given, as are the Likelihood Ratio Test (LRT) score and corresponding p value for df=1.
- https://doi.org/10.7554/eLife.37621.009
-
Supplementary file 2
Variance was substantially higher for the output link in mantis shrimp and sunfish.
Therefore, for these systems, we repeated pair-wise comparisons of evolutionary rate while incorporating intraspecific measurement error (With Error). We also present results for rate comparisons without measurement error incorporated (No Error). For each comparison, the AIC score for a model in which rates are allowed to vary (AICobserved) and constrained to be equal (AICconstrained) are given, as are the Likelihood Ratio Test (LRT) score and corresponding p value for df=1. Evolutionary rate differences between linkages were robust to measurement error.
- https://doi.org/10.7554/eLife.37621.010
-
Supplementary file 3
A rotatable 3D phylomorphospace plot reveals variation in mechanical output (KT, denoted with color) with respect to oral four-bar linkage morphology (x, y, and z axes) across 30 species of cichlids (Family: Cichlidae).
KT is inversely correlated with the input link and, to a lesser extent, positively correlated with the coupler link. To view these relationships, scroll the plot to place the input and coupler links as x and y-axes, and note the linear correlation between the two variables, coupled with a distinct color transition in the KT color map overlaid on the data. To zoom in and out of the plot scroll upwards and downwards, respectively.
- https://doi.org/10.7554/eLife.37621.011
-
Supplementary file 4
Mechanical output (KT, denoted with color) varies with respect to oral four-bar linkage morphology (x, y, and z axes) across 101 species of wrasses (Family: Labridae).
KT is strongly correlated with the input and output links, and these relationships can be viewed dynamically in 3D phylomorphospace by scrolling the plot to place the input and output links as x and y axes, and noting the linear correlation between the two variables, coupled with a distinct color transition in the KT colormap overlaid on the data. Patterns of mechanical sensitivity in the wrasse oral four-bar were similar in the opercular four-bar of sunfish, but quite different from the oral four-bar of cichlids, despite a common evolutionary origin. To zoom in and out of the plot scroll upwards and downwards, respectively.
- https://doi.org/10.7554/eLife.37621.012
-
Supplementary file 5
A rotatable 3D phylomorphospace plot reveals differential mechanical sensitivity between KT (denoted with color map) and linkage morphology (x, y, and z axes of plot) across 19 species of sunfish (Family: Centrarchidae).
KT is inversely correlated with the output link and positively correlated with the input link. To view this relationship, place the input and output links as x and y-axes, and note the linear correlation between the two variables, coupled with a distinct color transition in the KT color map overlaid on the data. The weak relationship association between the coupler link and KT can be viewed by placing the coupler link on the x-axis, and noting the lack of correlation with other links as well as no consistent association with the KT color map. This pattern of sensitivity is similar to the oral four-bar of wrasses.
- https://doi.org/10.7554/eLife.37621.013
-
Supplementary file 6
A rotatable 3D phylomorphospace illustrates variation in mechanical output (KT, denoted with color map) with respect to linkage morphology (x, y, and z axes of plot) across 36 species of mantis shrimp (Order: Stomatopoda).
KT is inversely correlated with the output link, and is uncorrelated with the input and coupler links. These patterns can be visualized by rotating the plot to place the output link on the x-axis, and noting the strong transition from high values of KT (red) when the output link is small to low values of KT (blue) when the output link is large. Plotting the input and coupler links on the x and y axes shows no relationship (color is dispersed across morphospace). Scrolling up and down zooms in and out of the plot, respectively.
- https://doi.org/10.7554/eLife.37621.014
-
Supplementary file 7
Analysis of evolutionary shifts for one mechanical trait (KT) and three morphological traits (input link, output link, and coupler link) in wrasses.
For each shift, the branch and the shift’s posterior probability (pp) are given. The branches that were strongly supported (pp > 0.5) are denoted in bold, and depicted in Figure 3.
- https://doi.org/10.7554/eLife.37621.015
-
Supplementary file 8
Time-calibrated phylogeny of cichlids (Family: Cichlidae) used in this study.
This phylogeny was constructed using the previously-published phylogeny by Hulsey et al., 2010.
- https://doi.org/10.7554/eLife.37621.016
-
Supplementary file 9
Time-calibrated phylogeny of mantis shrimp (Stomatopoda) used in this study was based on the previously-published phylogeny by Porter et al. (2010).
- https://doi.org/10.7554/eLife.37621.017
-
Supplementary file 10
Time-calibrated phylogeny of sunfish (Family: Centrarchidae) used in this study.
We constructed this phylogeny using the previously-published phylogeny by Near et al., 2005.
- https://doi.org/10.7554/eLife.37621.018
-
Supplementary file 11
Time-calibrated phylogeny of wrasses (Family: Labridae) used in this study.
This phylogeny is based on the previously-published wrasse phylogeny by Baliga and Law, 2016.
- https://doi.org/10.7554/eLife.37621.019
-
Supplementary file 12
The mechanical and morphological data for the cichlid (Family: Cichlidae) species used in this study.
Data were gathered from Hulsey and Garcia De Leon, 2005.
- https://doi.org/10.7554/eLife.37621.020
-
Supplementary file 13
The mechanical and morphological data for the stomatopod species used in this study.
Data were gathered from Anderson and Patek, 2015.
- https://doi.org/10.7554/eLife.37621.021
-
Supplementary file 14
The mechanical and morphological data for the sunfish (Family: Centrarchidae) species used in this study.
Data were gathered from Hu et al., 2017.
- https://doi.org/10.7554/eLife.37621.022
-
Supplementary file 15
The mechanical and morphological data for the wrasses (Family: Labridae) used in this study.
Data were gathered from Alfaro et al., 2005.
- https://doi.org/10.7554/eLife.37621.023
-
Supplementary file 16
Analyses of mechanical sensitivity in mantis shrimp and sunfish are robust to static measures of KT (see Materials and methods).
Table shows PGLS regressions examining the relationship between mobile links (predictor variable) and kinematic transmission (response variable) using static (rather than dynamic) measures of KT in mantis shrimp and sunfish.
- https://doi.org/10.7554/eLife.37621.024
-
Supplementary file 17
Using residuals of trait values produces similar mechanical sensitivity results to the commonly utilized size corrections (mobile lever/fixed lever).
Here, the predictor variables were the residuals of mobile link length regressed against fixed link length. Raw data for the wrasses were not available.
- https://doi.org/10.7554/eLife.37621.025
-
Transparent reporting form
- https://doi.org/10.7554/eLife.37621.026