TrpV1 receptor activation rescues neuronal function and network gamma oscillations from Aβ-induced impairment in mouse hippocampus in vitro
Figures
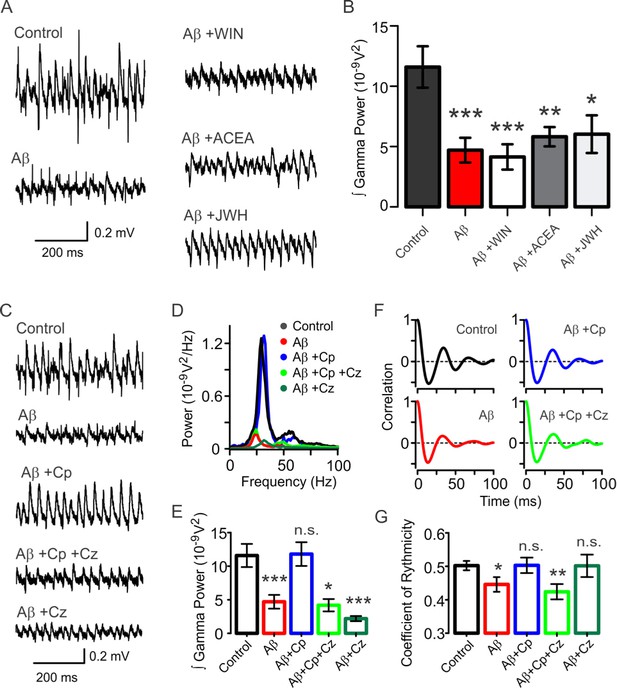
Activation of TrpV1, but not CB1/CB2, protects the hippocampal network from Aβ-induced degradation of gamma oscillations.
(A) Representative sample traces of KA-induced gamma oscillations in hippocampal slices. Control: gamma oscillations 20 min after 100 nM KA bath application; Aβ: degradation of KA-induced gamma oscillations after 15 min pre-incubation with 50 nM Aβ; Aβ+WIN: pre-incubation with CB1/CB2 receptor agonist WIN does not protect gamma oscillations from Aβ-induced degradation; Aβ+ACEA: CB1 agonist ACEA; Aβ+JWH: CB2 agonist JWH; both also show no protective properties against the Aβ-induced degradation. (B) Summary histogram of integrated gamma power from the experimental conditions described in A. (C) Representative sample traces of control gamma oscillation (control) and the Aβ-induced reduction of gamma oscillations (Aβ). TrpV1 activation by its agonist Cp (10 µM) protected gamma oscillations from Aβ-induced impairment (Aβ+Cp) while TrpV1 antagonist Cz (10 µM) blocked Cp protection (Aβ+Cp+Cz). The effect of Aβ together with Cz is also shown (Aβ+Cz). (D) Power spectra for the experimental conditions described in C: Control (black), Aβ (red), Aβ+Cp (blue), Aβ+Cp+Cz; (green), Aβ+Cz; (dark green). (E) Integrated power of gamma oscillations from the experiments described in C and D, showing the protective properties of TrpV1 activation by Cp. (F) Average auto-correlograms showing the Aβ-induced reduction of the quality of gamma oscillation and the protective effect following TrpV1 activation. (G) Summary bar-graph of the coefficient of rhythmicity (Cr) calculated from the experimental conditions described in F. The effect of Aβ together with Cz is also shown (Aβ+Cz). Quantification of integrated power and Cr were performed from the same 60s-segment 20 min after gamma oscillations induction by bath application of 100 nM KA (for experimental data see Figure 1—source data 1). Mann-Whitney test (one-tailed) was used for statistical significance on absolute values. Data is presented as mean ± SEM. * indicates p < 0.05, **p < 0.01, ***p < 0.001 and n.s. indicates no statistically significant differences.
-
Figure 1—source data 1
Experimental data for Figure 1.
- https://doi.org/10.7554/eLife.37703.005
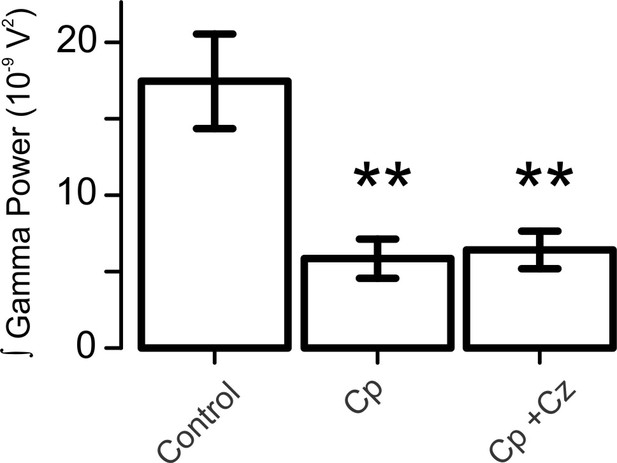
Capsaicin induces a reduction in hippocampal gamma oscillation power via a TrpV1-independent mechanism.
Summary bar-graph showing the integrated power of gamma oscillations (normalized) from slices in control conditions, slices treated with 10 µM Cp and slices treated with 10 µM Cp plus 10 µM Cz. Note that Cz did not block the reduction in gamma power, indicating a mechanism independent of TrpV1 receptor activation (for experimental data see Figure 1—figure supplement 1—source data 1). Mann-Whitney test (one-tailed) was used for statistical significance on absolute values. Data is presented as mean ± SEM. ** indicates p < 0.01.
-
Figure 1—figure supplement 1—source data 1
Experimental data for Figure 1—figure supplement 1.
- https://doi.org/10.7554/eLife.37703.004
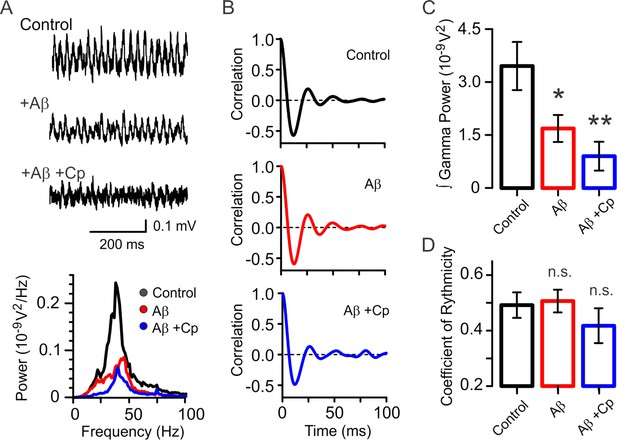
Capsaicin’s preventative effect is absent in hippocampal slices from TrpV1 KO mice.
(A) Representative sample traces and power spectra of KA-induced gamma oscillations in hippocampal slices from TrpV1 KO mice showing that Cp does not display the same protective properties against Aβ-induced reduction in gamma oscillations as in WT slices (Figure 1C–G). (B) Likewise, the coefficient of rhythmicity (Cr) remains unaltered after Aβ and Aβ+Cp treatments compared to control. (C) Integrated power of gamma oscillations from the experimental conditions described in A: Control (black), Aβ (red) and Aβ+Cp (blue). (D) Summary bar-graph of Cr from the experimental conditions described in B. Quantifications of integrated power and Cr were performed from the same 60s-segment 20 min after gamma oscillations induction by bath application of 100 nM KA (for experimental data see Figure 2—source data 1). Mann-Whitney test (one-tailed) was used for statistical significance on absolute values. Data is presented as mean ± SEM. * indicates p < 0.05, ** p < 0.01 and n.s. indicates no statistically significant differences.
-
Figure 2—source data 1
Experimental data for Figure 2.
- https://doi.org/10.7554/eLife.37703.007
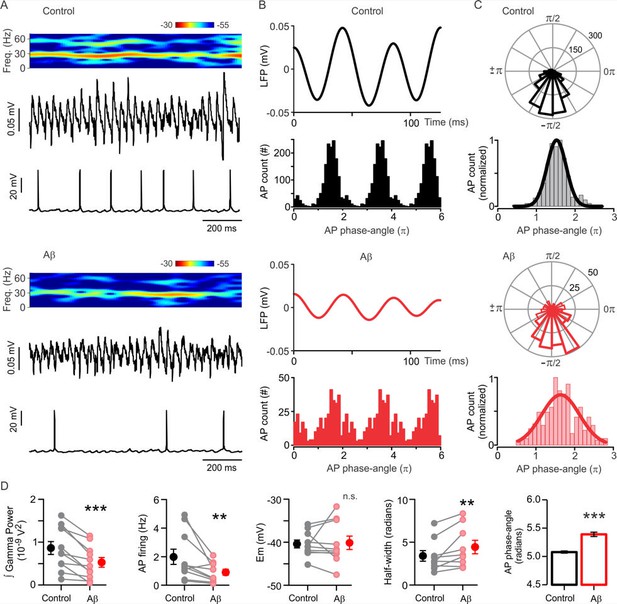
Aβ-induced desynchronization of AP firing in CA3 pyramidal cells.
(A) Representative sample traces of concomitant LFP and patch-clamp recordings showing the Aβ-induced impairment of gamma oscillation power and AP firing rate. The spectrogram of the LFP activity shows the reduction in gamma oscillations power. (B) Filtered LFP recording showing three cycles of gamma oscillations (top) and frequency distribution plot of the AP gamma-phase-angles (bottom) in control (black) and after Aβ application (red). The frequency distribution plot shows the AP gamma-phase-angles repeated three times to represent the AP fire windows during gamma oscillations. (C) Representative polar-plots (top) showing the distribution of the AP phase-angles before (black) and after 1 µM Aβ application (red). Phase-angles and gamma oscillation-phases are presented in radians; the peak of the oscillation cycle corresponds to 0π and the trough corresponds to ±π. Normalized frequency-distribution (bottom) showing the AP phase-angles to which a Gaussian function was fitted to calculate the half-width as a measure of the synchronization level before and after Aβ application (see Materias and methods). Note that Aβ application induced a reduction in the number of AP (see AP count in (B) and polar plots scale in (C) and increased the AP fire window half-width. (D) Quantification of the integrated power, AP firing, AP firing window half-width and AP phase-angles before (control, black) and after 1 µM Aβ application (red). Note that Aβ induced a shift in the preferred phase-angle and a desynchronization of AP firing represented by an increase in the half-width of the AP firing windows (Gaussian curve fitted). Quantifications, except for half-width, were performed by averaging 5 min of control and the last 5 min of Aβ treatment with values taken over 1 min period (for experimental data see Figure 3—source data 1). Wilcoxon matched-pairs test (one-tailed) was used for statistical significance on absolute values in all quantifications except AP phase-angles for which statistically significant differences were tested by using the Mann-Whitney test (one-tailed). In all quantifications except for AP phase-angles each experiment is presented as before-after data and the mean ± SEM is also shown. ** indicates p < 0.01, ***p < 0.001 and n.s. indicates no statistically significant differences.
-
Figure 3—source data 1
Experimental data for Figure 3.
- https://doi.org/10.7554/eLife.37703.009
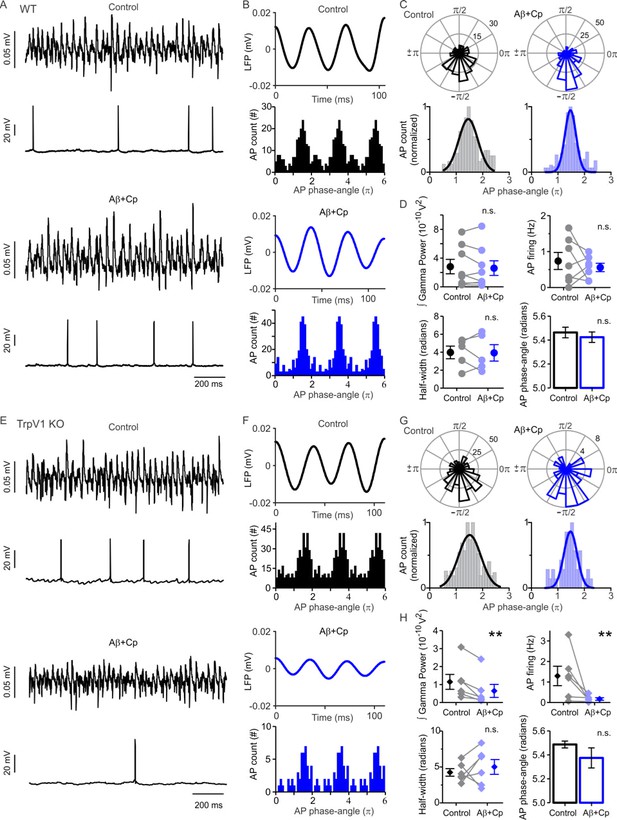
TrpV1 activation prevents Aβ-induced desynchronization of AP firing.
(A) Representative sample traces of concomitant LFP and patch-clamp recordings in WT slices before (control, black) and after 1 µM Aβ plus 5 µM Cp application (blue). (B) Filtered WT LFP recording showing three cycles of gamma oscillations (top) and frequency distribution plot of the AP gamma-phase-angles (bottom) in control (black) and after Aβ+Cp application (blue). The frequency distribution plot shows the WT AP gamma-phase-angles repeated three times to represent the AP fire windows during gamma oscillations. (C) Representative polar-plots (top) showing the distribution of the WT AP phase-angles before (control, black) and after Aβ+Cp application (blue). Phase-angles and gamma oscillation-phases are presented in radians; the peak of the oscillation cycle corresponds to 0π and the trough corresponds to ±π. Normalized frequency-distribution (bottom) showing the AP phase-angles to which a Gaussian function was fitted to calculate the half-width to show the synchronization level before and after Aβ application (see Materials and methods). (D) Quantification of the integrated gamma power, AP firing, AP firing window half-width and AP phase-angles before (control, black) and after Aβ+Cp application (blue) in WT slices. (E) Representative sample traces of concomitant LFP and patch-clamp recordings in TrpV1-KO slices before (control, black) and after 1 µM Aβ plus 5 µM Cp application (blue). (F) Filtered TrpV1-KO LFP recording showing three cycles of gamma oscillations (top) and frequency distribution plot of the AP gamma-phase-angles (bottom) in control (black) and after Aβ+Cp application (blue). (G) Representative polar-plots (top) showing the distribution of the TrpV1-KO AP phase-angles before (control, black) and after Aβ+Cp application (blue). Normalized frequency-distribution (bottom) showing the AP phase-angles with the Gaussian function fitted. (H) Quantification of the integrated gamma power, AP firing, AP firing window half-width and AP phase-angles before (control, black) and after Aβ+Cp application (blue) in TrpV1-KO slices. Note that application of 5 µM Cp together with 1 µM Aβ prevented the Aβ-related reduction in KA-induced gamma oscillation power and AP firing rate in WT but not in TrpV1-KO slices demonstrating that the preventive role of Cp is mediated by the activation of TrpV1. Quantifications, except for half-width, were performed by averaging 5 min of control and the last 5 min of Aβ+Cp treatment with values taken over 1 min period. Wilcoxon matched-pairs test (one-tailed) was used for statistical significance on absolute values in all quantifications except AP phase-angles for which statistically significant differences were tested by using the Mann-Whitney test (one-tailed). In all quantifications except for AP phase-angles each experiment is presented as before-after data and the mean ± SEM is also shown. ** indicates p < 0.01 and n.s. indicates no statistically significant differences (for experimental data see Figure 4—source data 1).
-
Figure 4—source data 1
Experimental data for Figure 4.
- https://doi.org/10.7554/eLife.37703.013
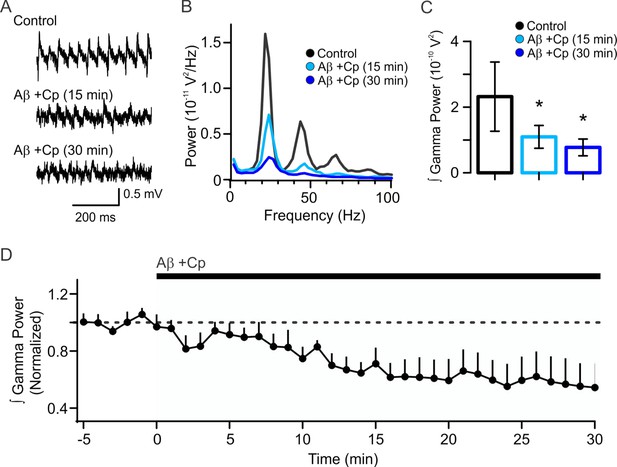
10 µM capsaicin does not prevent Aβ-induced reduction in gamma oscillation power.
(A–B) Representative sample traces and power spectra of KA-induced gamma oscillations in hippocampal slices before (control, black) and after 1 µM Aβ and 10 µM Cp application (15 min, light blue; 30 min, dark blue). (C) Quantification of the integrated power of the experimental conditions shown in A. (D) Time-course of normalized gamma power before and after Aβ+Cp treatment. Note that 10 µM Cp did not prevent Aβ-induced reduction in gamma power (for experimental data see Figure 4—figure supplement 1—source data 1). Wilcoxon signed rank test (one-tailed) was used for statistical significance on absolute values. Data is presented as mean ± SEM. * indicates p < 0.05.
-
Figure 4—figure supplement 1—source data 1
Experimental data for Figure 4—figure supplement 1.
- https://doi.org/10.7554/eLife.37703.012
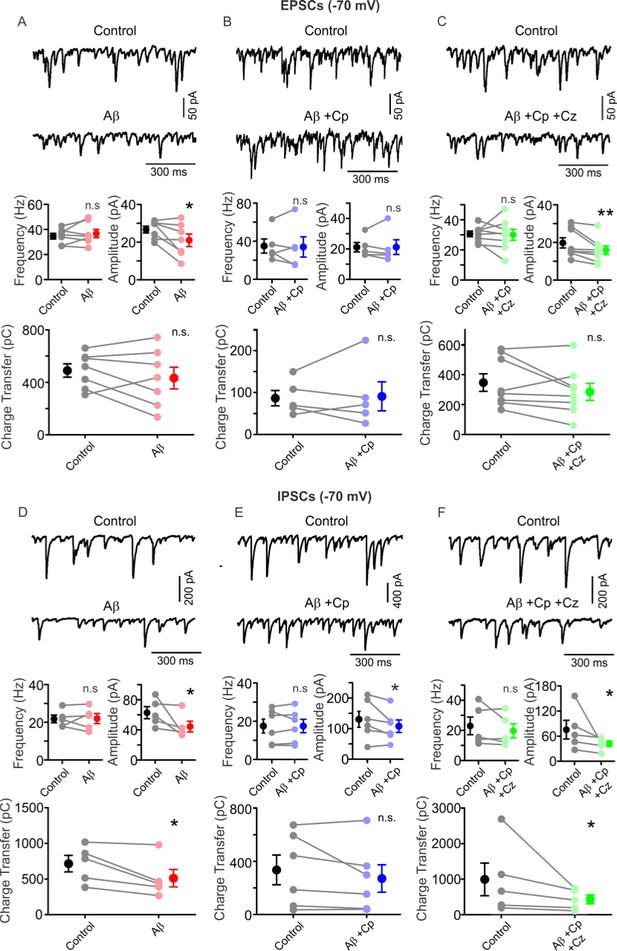
TrpV1 activation prevents Aβ-induced impairment of EPSC/IPSC current balance.
(A) Representative traces (top) of EPSCs (PC, Vh=-70mV) before (control, black) and after 1 µM Aβ application (red) in hippocampal slices activated with 100 nM KA. Summary-graph (bottom) of the EPSCs frequency, amplitude and charge transfer before (control, black) and after Aβ application (red). (B) Representative traces (top) of EPSCs (PC, Vh=-70mV) before (control, black) and after 1 µM Aβ plus 5 µM Cp application (blue) in hippocampal slices activated with 100 nM KA. Summary-graph (bottom) of the EPSC frequency, amplitude and charge transfer before (control, black) and after Aβ+Cp application (blue). (C) Representative traces (top) of EPSCs (PC, Vh=-70mV) before (control, black) and after 10 µM Cz together with 1 µM Aβ plus 5 µM Cp application (green) in hippocampal slices activated with 100 nM KA. Summary-graph (bottom) of the EPSC frequency, amplitude and charge transfer before (control, black) and after Aβ+Cp+ Cz application (green). (D) Representative traces (top) of IPSCs (PC, Vh=-70mV) before (control, black) and after 1 µM Aβ application (red) in hippocampal slices activated with 100 nM KA. Summary-graph (bottom) of the IPSC frequency, amplitude and charge transfer before (control, black) and after Aβ application (red). (E) Representative traces (top) of IPSCs (PC, Vh=-70mV) before (control, black) and after 1 µM Aβ plus 5 µM Cp application (blue) in hippocampal slices activated with 100 nM KA. Summary-graph (bottom) of the IPSC frequency, amplitude and charge transfer before (control, black) and after Aβ+Cp application (blue). (F) Representative traces (top) of IPSCs (PC, Vh=-70mV) before (control, black) and after 10 µM Cz together with 1 µM Aβ plus 5 µM Cp application (green) in hippocampal slices activated with 100 nM KA. Summary-graph (bottom) of the IPSC frequency, amplitude and charge transfer before (control, black) and after Aβ+Cp+ Cz application (green). Note that Cp treatment reversed Aβ-related impairment of excitatory/inhibitory current balance by restoring EPSC amplitude and IPSC charge transfer. Quantifications were performed by averaging 5 min of control and the last 5 min of treatment with values taken over 1 min period (for experimental data see Figure 5—source data 1). Wilcoxon matched-pairs test (one-tailed) was used for statistical significance on absolute values. Each experiment is presented as before-after data and the mean ±SEM is shown. * indicates p < 0.05, **p < 0.01 and n.s. indicates no statistically significant differences.
-
Figure 5—source data 1
Experimental data for Figure 5.
- https://doi.org/10.7554/eLife.37703.017
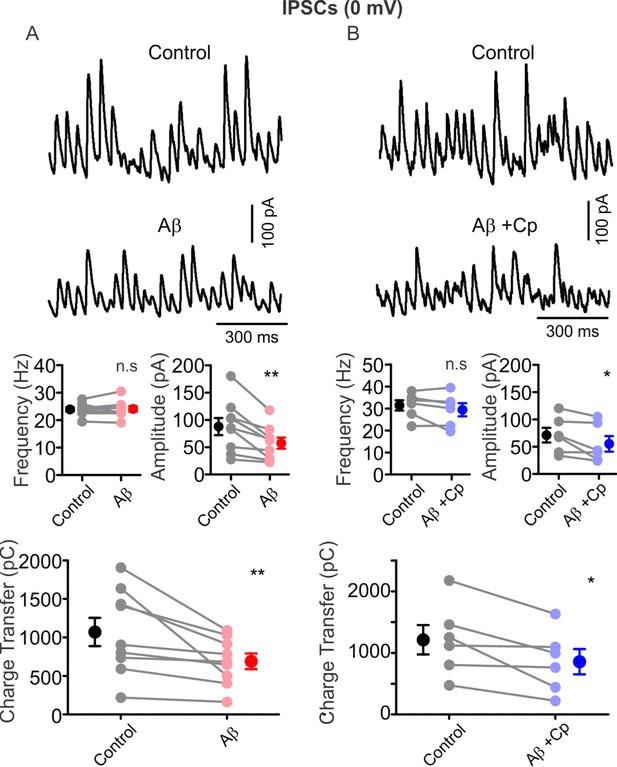
Cp does not prevent the Aβ-induced impairment of IPSC parameters recorded at Vh = 0 mV.
(A) Representative traces (top) of IPSCs (PC, Vh = 0 mV) before (control, black) and after 1 µM Aβ application (red) in hippocampal slices activated with 100 nM KA. Summary-graph (bottom) of IPSC frequency, amplitude and charge transfer before (control, black) and after Aβ application (red). (B) Representative traces (top) of IPSCs (PC, Vh = 0 mV) before (control, black) and after 1 µM Aβ plus 5 µM Cp application (blue) in hippocampal slices activated with 100 nM KA. Summary-graph (bottom) of the IPSC frequency, amplitude and charge transfer before (control, black) and after Aβ+Cp application (blue).Quantifications were performed by averaging 5 min of control and the last 5 min of treatment with values taken over 1 min period (for experimental data see Figure 5—figure supplement 1—source data 1). Wilcoxon signed rank test (one-tailed) was used for statistical significance on absolute values. Each experiment is presented as before-after data and the mean ±SEM is shown. * indicates p < 0.05 and **p < 0.01.
-
Figure 5—figure supplement 1—source data 1
Experimental data for Figure 5—figure supplement 1.
- https://doi.org/10.7554/eLife.37703.016
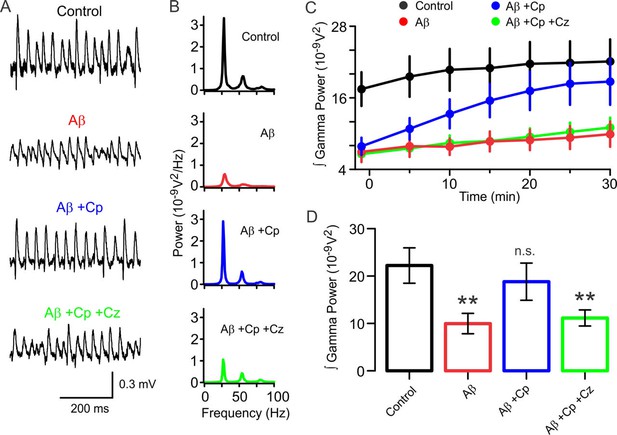
TrpV1 activation rescues the hippocampal network from Aβ-induced degradation of gamma oscillations.
(A,B) Representative sample traces and power spectra of KA-induced gamma oscillations in hippocampal slices after 30 min of control activity (black), and from slices pre-incubated with 50 nM Aβ (Aβ, red). Gamma power from Aβ-pre-incubated slices treated with 10 µM Cp (Aβ+Cp, blue), and Aβ-pre-incubated slices treated with 10 µM Cp together with 10 µM Cz (Aβ+Cp+Cz, green). Note that Cp treatment rescues gamma oscillations to levels similar to control. (C) Time-course of the integrated power of gamma oscillations from the experimental conditions described in A and B showing the time-dependent increase in gamma power in the slices treated with Cp (blue). Note that gamma power in Aβ-pre-incubated slices not treated with Cp remained low (Aβ, red). Similar results were obtained when Cz was included with the treatment (Aβ+Cp+Cz, green) indicating that the TrpV1 receptor mediates the restorative effect exerted by Cp. (D) Summary bar-graph of the integrated gamma power from the experimental conditions described in A. Integrated power was measured on 60 s segments every 5 min after treatment application. Quantification was performed after 30 min of treatment application. In all experiments, recordings were started 20 min after gamma oscillation induction by 100 nM KA bath application (for experimental data see Figure 6—source data 1). Mann-Whitney test (one-tailed) was used for statistical significance on absolute values. Data is presented as mean ± SEM. * indicates p < 0.05 and n.s. indicates no statistically significant differences.
-
Figure 6—source data 1
Experimental data for Figure 6.
- https://doi.org/10.7554/eLife.37703.019
Tables
Reagent type (species) or resource | Designation | Source or reference | Identifiers | Additional information |
---|---|---|---|---|
Genetic reagent (Mus musculus) | C57BL/6 | Charles River Laboratories | Strain Code: 027 | |
Genetic reagent (M. musculus) | TrpV1 KO | Jackson Laboratory | Stock No: 003770 RRID:IMSR_JAX:003770 | PubMed: 10764638 |
Peptide, recombinant protein | Recombinant Aβ1–42 | PMID: 25143621 | Prof. Jan Johansson (Karolinska Institutet, Sweden) | |
Chemical compound, drug | Kainate Acid | Tocris Bioscience | Cat. No. 0222 | 100 nM |
Chemical compound, drug | (E)-Capsaicin | Tocris Bioscience | Cat. No. 0462 | 5, 10 µM |
Chemical compound, drug | Capsazepine | Tocris Bioscience | Cat. No. 0464 | 10 µM |
Chemical compound, drug | Win55,212–2 mesylate | Tocris Bioscience | Cat. No. 1038 | 200 nM, 1 µM |
Chemical compound, drug | JWH 133 | Tocris Bioscience | Cat. No. 1343 | 100 nM |
Chemical compound, drug | Arachidonyl-2'-chloroethylamide | Tocris Bioscience | Cat. No. 1319 | 200 nM |
Software | Spike phase-coupling code | Custom software | See Source code file 1 provided with this study | MATLAB script. Dr. André Fisahn (Karolinska Institutet, Sweden) |
Additional files
-
Source code 1
Spike phase-coupling code.
- https://doi.org/10.7554/eLife.37703.020
-
Source data 1
Unitary AP recordings.
- https://doi.org/10.7554/eLife.37703.021
-
Transparent reporting form
- https://doi.org/10.7554/eLife.37703.022