Individuals physically interacting in a group rapidly coordinate their movement by estimating the collective goal
Figures
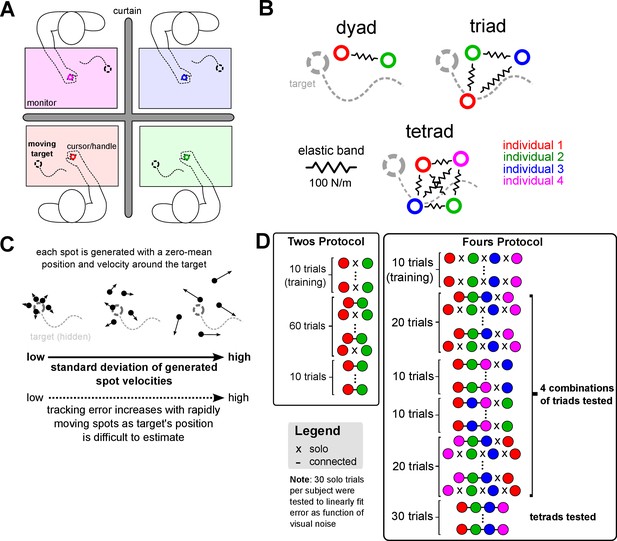
Dyads, triads and tetrads, whose right hands were connected, tracked a common target together to investigate collective physical interaction.
(A) Subjects were recruited in twos or fours (schematic shows a tetrad). Each participant held onto a robotic handle with their dominant right hand to control a cursor on a monitor and track the same randomly moving target. Only one’s own cursor and the target were displayed on the monitor. (B) Physical coordination for dyads, triads and tetrads was enabled by forces exerted through the robotic handle that elastically connected all individuals’ right hands together. The interaction was removed in some trials to measure each individual’s solo tracking error as a function of the deviation in the target spots’ velocities. (C) The target was composed of five spots were spread thinly or widely to control each individual’s tracking performance. The deviation in the spots’ velocities, which was fixed during a trial, was randomized at every trial so participants could not know their skill relative to their partners beforehand. (D) Experimental protocol for twos and fours, where each circle represents an individual denoted by color. A dash indicates the individual was connected to partners. Both twos and fours experienced 10 solo training trials to become acquainted to the task. Twos then experienced 30 pairs of trials with and without the elastic connection, that is connected-solo-connected-solo etc. for 30 repetitions, and then 10 connected trials. For fours, three individuals of a tetrad were selected, forming all four different combinations of triads, and interacted in triads for one block per combination. In the first and last of these triad blocks, triads experienced 10 pairs of connected-solo trials, while the second and third triad blocks was composed of 10 connected trials. This was done to intersperse the solo trials to have a robust measure of the relationship between visual noise level and tracking error. In the final block, all individuals interacted as a tetrad for 30 trials.
The video shows a 15 second trial that each subject observed on their screen during training trials when the noise was lowest.
The target is composed of five spots, which are regenerated every 400ms.
This video shows a 15-s trial where the visual noise was highest.
Each of the five spots generated around the actual target position rapidly spread out as their velocity is picked from a wider Gaussian distribution.
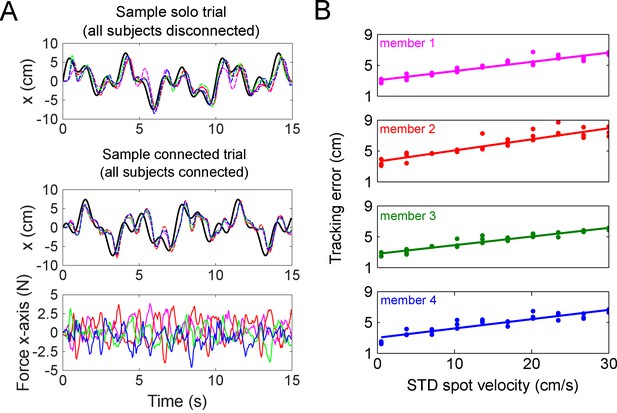
Sample trajectories from a single trial, and how the tracking error is tightly related with the visual noise imposed by the experimenter on each individual’s target.
(A) Raw data showing the trajectories in the x-axis from a sample tetrad, where the black trace is the target and each dashed colored trace (red, green, blue and magenta) is one subject. The top panel shows the x-axis position of all subjects in a tetrad in a solo trial (all disconnected), whereas the bottom two panels are the x-axis position and force from a sample connected trial where all four subjects were coupled to each other via elastic bands. Subjects’ positions in both the sample solo and connected trials were all delayed with respect to the target due to visual feedback delays in anticipating the target’s motion. The force felt by each partner is approximately zero mean, and depended on each individual’s relative position to their partners in the group. (B) Linear fit of the standard deviation of spots’ velocities versus the tracking error from a sample tetrad. Each level of noise was tested for three trials without the elastic band to assess individual tracking error. This data was linearly regressed to estimate the expected tracking error of each individual as a function of the visual noise on the target imposed by the experimenter. This enabled us to test collectives composed of individuals with different tracking skill.
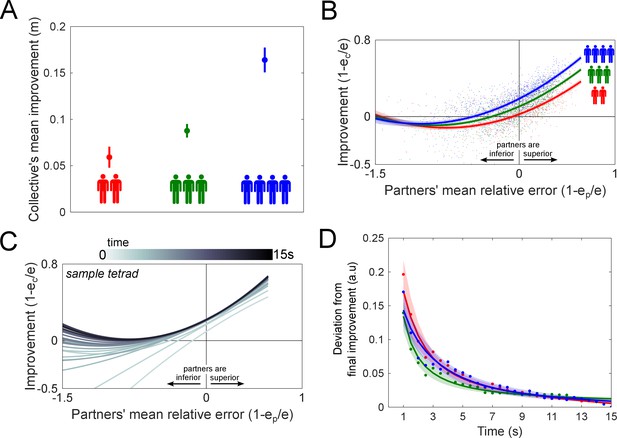
Collective physical interaction was surprisingly beneficial with coordination emerging rapidly in seconds, with the benefit in performance increasing with the number of partners.
(A) The collective improvement for dyads, triads and tetrads increased with the collective’s size, reflecting the advantage of larger collectives. (B) Performance improvement as a function of the partners’ relative error for dyads (red trace), triads (in green) and tetrads (in blue). The solid traces come from a linear mixed-effects fit of the raw data (points come from all connected trials from all groups). Interacting with a superior group was found to improve one’s performance, which was graded by the collective’s size such that a larger collective resulted in more improvement. We expected a similar effect when interacting with an inferior collective, but interacting with more inferior partners did not degrade a superior member’s performance. (C) The performance improvement of a sample tetrad is plot in increments of 0.5 s from the start to the end of the trial as a function of the partners’ relative error. The improvement rapidly converged to the improvement curve observed in Figure 3B. (D) The deviation of the improvement curve in Figure 3B from the final improvement curve is plotted as a function of the trial time for dyads, triads and tetrads. The solid line is the mean of all groups, and the area represents one standard error. The rate at which they deviated from the collective mean was independent of group size.
-
Figure 3—source data 1
Data of the partners’ mean relative error, improvement, group label and the group size used in the linear mixed-effects analysis.
This data was also used in Figure 4 and 5.
- https://doi.org/10.7554/eLife.41328.007
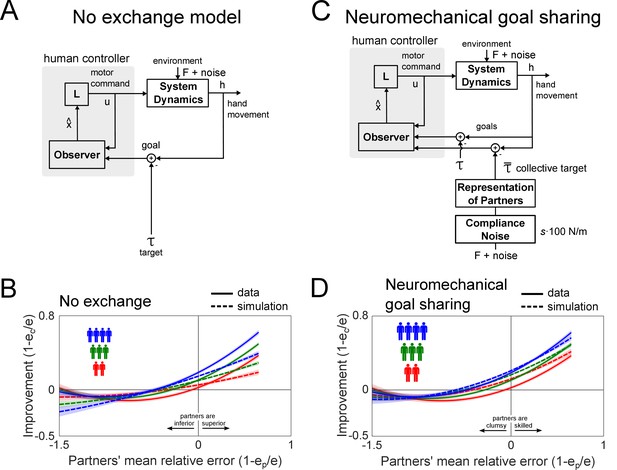
Simulations of the two proposed models of collective interaction and their predictions.
(A) The no exchange model was simulated by assuming that individuals cannot interpret the interaction forces and track the target as if they were alone under the influence of the mechanics of the elastic band. (B) This model predicted a larger inferior collective to be a greater hindrance to a superior individual of the collective, unlike the data where superior individuals maintained their performance. (C) In the neuromechanical goal sharing model, each individual built a representation of the partners’ average behavior to estimate the collective target. (D) The predictions from this model best explained the improvement of both inferior and superior individuals in the collective, and its modulation with the collective’s size.
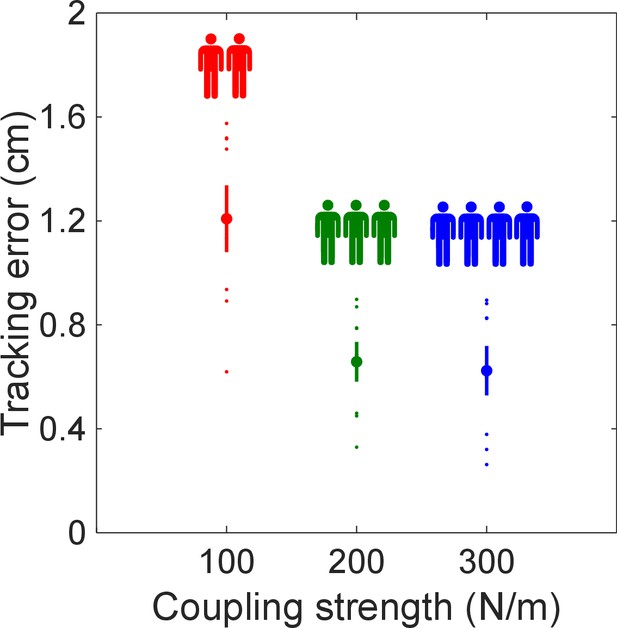
Results of the control experiment from a different set of subjects who tracked a haptic target without visual feedback.
This experiment estimated how the interaction mechanics of coupling in dyads, triads and tetrads affected the quality of the estimated target from haptics. The compliance in the connection for dyads implies that dyads have more difficulty in estimating the collective target compared with triads and tetrads who felt a greater force. These values were used to adjust the neuromechanical goal sharing and the source separation limiting case.
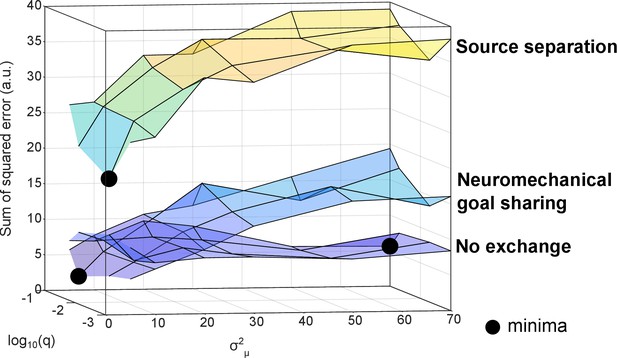
The sum of the sum of squared error between the fits from the data and the simulation as a function of and .
The SSE is relatively insensitive to changes in the strength . The model with the lowest SSE is the neuromechanical goal sharing model.
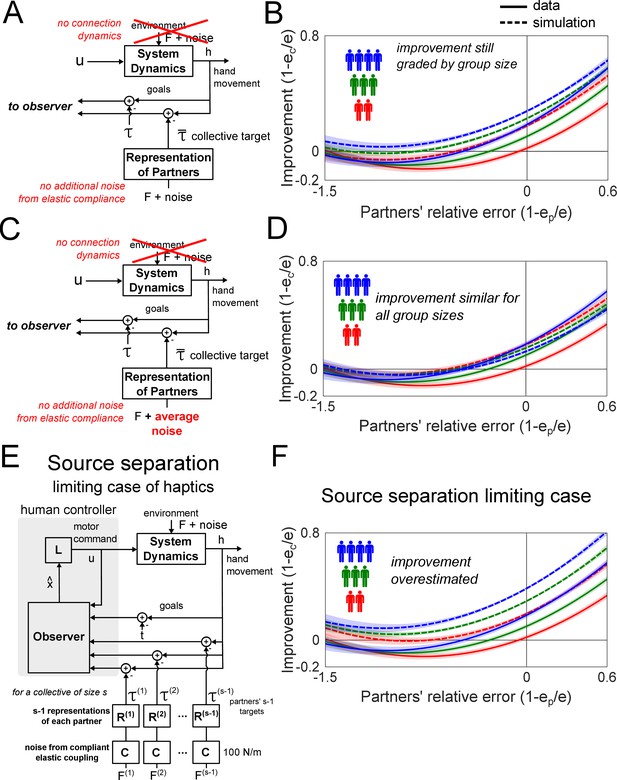
Simulation to examine the mechanism underlying the graded improvement and the limits of sharing information through haptics.
(A) A schematic of the neuromechanical goal sharing model without the dynamics of the spring and without the additional haptic noise due to the compliance of the spring. The simulation of this model are shown in (B), where the improvements remained graded by group size, implying that the performance was graded for a different reason. (C) The neuromechanical goal sharing model was simulated without the dynamics of the spring, without the additional haptic noise due to the compliance of the spring, and assuming that the noise in the interaction force was the average partners’ visual tracking noise. The simulation of this model is shown in (D). Since the noise was averaged, the group size had no effect on the improvement, which was similar for dyads, triads and tetrads. Thus, the graded improvement due to group size arose from the stochastic summation in the interaction force. (E) The source separation limiting case assumes that each individual receives additional sensory information of the target position through the interaction force from every partner. (F) The source separation case significantly overestimates the improvement for dyads, triads and tetrads, indicating that the individuals could not separate the sources of the interaction force.
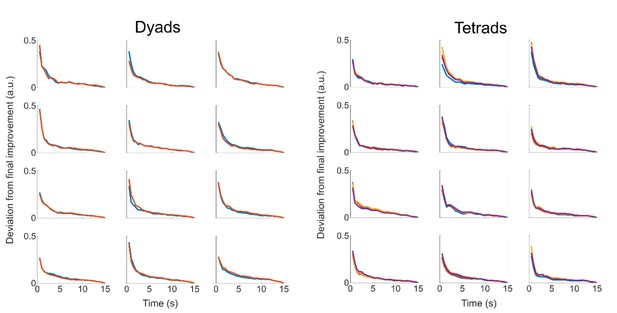
Deviation from the final improvement as a function of the time during the trial for every individual (denoted by a different colour) in all twos (left panels) and fours (right panels) recruited in our study.
The rates of convergence to the final improvement differs somewhat between different groups, but it was highly correlated between individuals of the same group. As such, it is difficult to isolate the contribution of a specific individual to the improvement of a collective, and so each group of twos and fours must be treated as a random factor in the analysis.
Additional files
-
Transparent reporting form
- https://doi.org/10.7554/eLife.41328.012