TMEM266 is a functional voltage sensor regulated by extracellular Zn2+
Figures
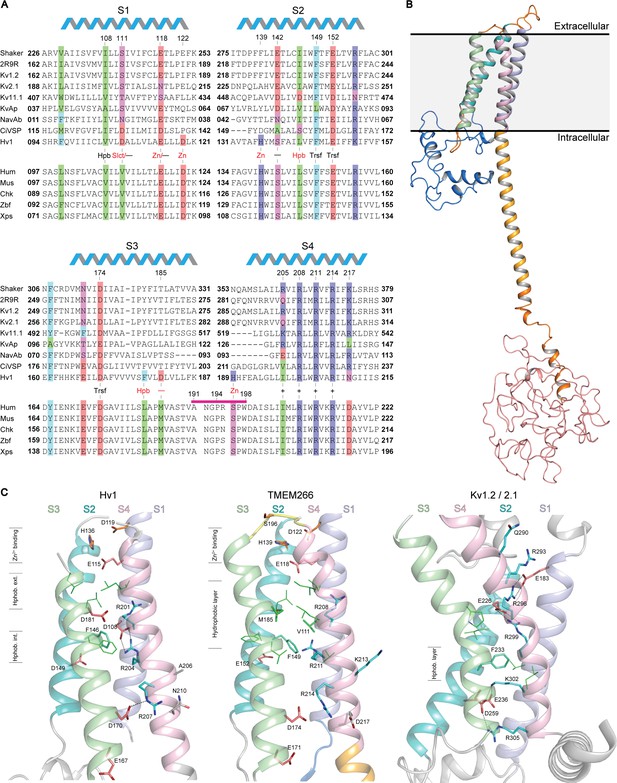
Relationship between the S1-S4 domain in hTMEM266 and those in established voltage sensors.
(A) Multiple sequence alignment of S1-S4 voltage-sensing domains from a selection of Kv and Nav channels, Ci-VSP, Hv1 and diverse orthologs of hTMEM266. A selection of highly conserved acidic (red), basic (blue), polar uncharged (magenta), aromatic (cyan) and aliphatic (green) residues are highlighted, including the functionally important S4 Arg (+), charge-transfer center (Trsf), and acidic and/or polarizable ‘counter-charges’ (-) at positions facing in towards the domain core. In addition, selected residues for Hv1 including the proton ‘selectivity filter’ (Slct), zinc binding residues (Zn), and conserved acidic (-) and hydrophobic residues (Hpb) at the core of the domain are labelled in red. hTMEM266 residue numbers are marked above for reference and the helical schematic above the alignment marks the extent of the helices in the homology model of hTMEM266, while residues that were mutated to Cys for fluorimetry experiments are indicated by a magenta bar. Sequences included in the alignment (identified here by their Refseq or PDB accession #) are: Drosophila Shaker (NP_001162788.1), Kv1.2/2.1 paddle chimera (PDB ID: 2R9R), Rat Kv1.2 (NP_037102.1), Rat Kv2.1 (NP_037318.1), Human Kv11.1 (NP_000229.1), Aeropyrum pernix Kv (Genbank: BAA79939.1), Arcobacter butzleri Nav (WP_012147720.1), Ciona intestinalis VSP (NP_001028998.1.), Mouse Hv1 (NP_001035954.1), along with TMEM266 orthologs from human (NP_689548.2), mouse (NP_766511.1), chicken (XP_001233623.3), zebrafish (NP_001074141.1) and Xenopus frog (XP_018108344.1). (B) Homology model of the full-length human hTMEM266 based on Hv1 structure (PDB ID: 3WKV). Predicted domains are color coded as follows: N-terminus (blue), S1 (light blue), S2 (cyan), S3 (light green), S4 (light pink), coiled coil (orange), disordered C-terminus (red). Membrane boundaries are estimated from superposition with the Kv1.2/2.1 paddle chimera structure where detergents and lipids are resolved. (C) Structural comparison of the S1-S4 domains of the human hTMEM266 homology model with those of Hv1 (PDB ID: 3WKV) and Kv1.2/2.1 paddle chimera (PDB ID: 2R9R). Backbone coloring scheme from (B) has been preserved and the functionally important S4 Arg residues (blue), S2 phenylalanine from the charge transfer center (cyan), and stabilizing acidic counter-charges (red) are rendered as sticks with dashed lines between the side chains denoting predicted H-bonds. In the Hv1 structure, aliphatic residues (green) directed into the 4TM core form distinct hydrophobic layers above (extracellular) and below (intracellular) the charge-transfer center. In contrast, in the hTMEM266 homology model there is a single, thicker hydrophobic layer extending upwards from the level of the charge transfer center, while the hydrophobic layer is lower and thinner in the Kv1.2/2.1 structure. Finally, residues believed to contribute to the extracellular Zn2+ binding sites are marked in orange on the Hv1 and hTEM266 structures.
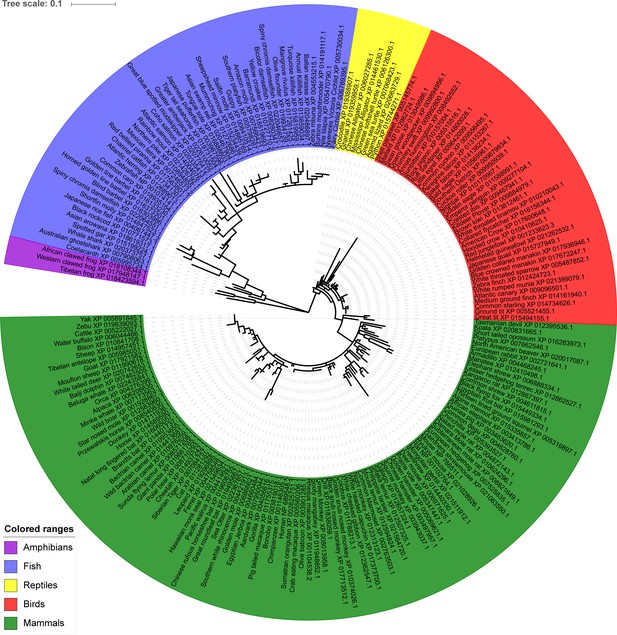
Phylogenetic analysis of TMEM266.
The evolutionary history was inferred using the Maximum Likelihood method based on the JTT matrix-based model. The tree with the highest log likelihood (−4450.66) is shown. Initial tree(s) for the heuristic search were obtained automatically by applying Neighbor-Join and BioNJ algorithms to a matrix of pairwise distances estimated using a JTT model, and then selecting the topology with superior log likelihood value. A discrete Gamma distribution was used to model evolutionary rate differences among sites (five categories (+G, parameter = 0.5924)). The tree is drawn to scale, with branch lengths measured in the number of substitutions per site. The analysis involved 198 amino acid sequences. All positions containing gaps and missing data were eliminated. There was a total of 193 positions in the final dataset. Evolutionary analyses were conducted in MEGA7.
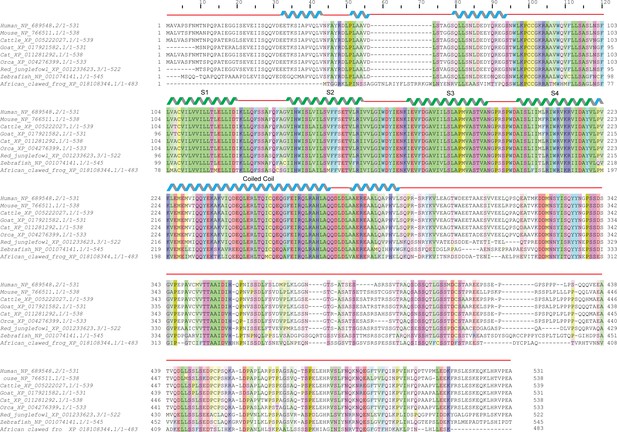
Sequence alignment and conservation of TMEM266.
Multiple sequence alignment of diverse orthologs of TMEM266. Highly conserved acidic (red), basic (blue), polar uncharged (magenta), aromatic (cyan) and aliphatic (green) residues are highlighted. Schematic above the alignment marks the extent of the secondary structural elements predicted in our homology model of hTMEM266.
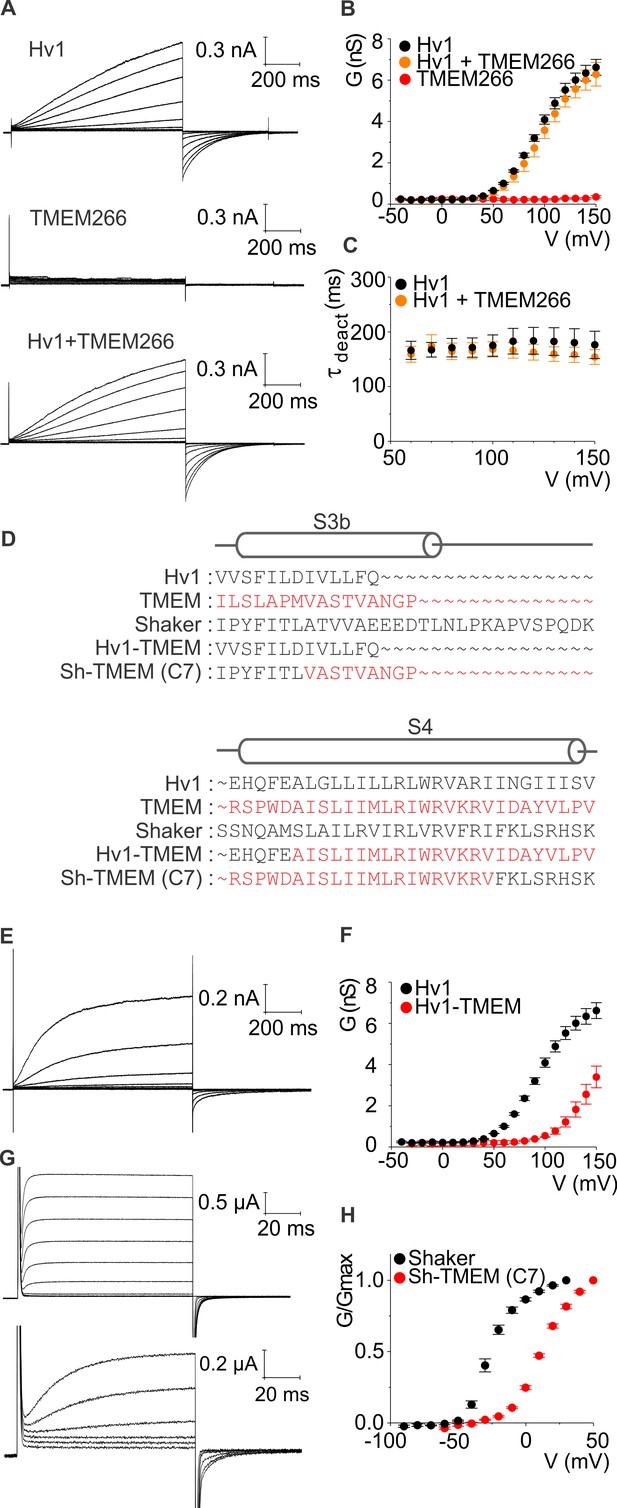
hTMEM266 does not function as a proton channel but its S4 helix can support voltage sensing in Hv1 and Kv channels.
(A) Whole-cell macroscopic currents recorded from HEK cells expressing hHv1, hTMEM266, and hHv1 plus hTMEM266 (top to bottom) elicited using 1 s test depolarization from −40 to +150 mV in increments of 10 mV. Holding voltage was −80 mV and tail voltage was −60 mV. Currents displayed are from −30 to 150 mV in increments of 20 mV for clarity. No leak correction has been applied. (B) Average conductance (G)-voltage (V) relationships for hHv1 (n = 4), hTMEM266 (n = 4), and hHv1 and hTMEM266 co-transfected (n = 3). (C) Average deactivation time constants (τ) derived from single-exponential fits to tail currents elicited by repolarization to −60 mV from cells in B. (D) Sequence alignments of hHv1, hTMEM266, Shaker and two chimeras. (E) Proton currents recorded from a HEK cell expressing the hHv1-hTMEM266 chimera elicited using 1 s test depolarization from −40 to +150 mV in increments of 10 mV. Holding voltage was −80 mV and tail voltage was −40 mV. Currents shown are from −30 to 150 mV in increments of 20 mV for clarity. No leak correction applied. (F) Average G-V relationships for Hv1 (n = 4) and the hHv1-hTMEM266 chimera (n = 3). (G) Macroscopic K+ currents recorded from oocytes expressing Shaker (top) and the Shaker-hTMEM266 chimera (bottom) elicited using 100 ms test depolarization from −90 to +80 mV in increments of 10 mV. Holding voltage was −90 mV and tail voltage was −60 mV for Shaker and −50 mV for the chimera. Current traces from −60 to 30 mV for Shaker and the Shaker-hTMEM266 chimera from −50 to 50 mV, in increments of 20 mV, are shown. (H) Average normalized G-V relationships for Shaker (n = 4) and Sh-hTMEM266 (n = 6). In all panels error bars represent SEM.
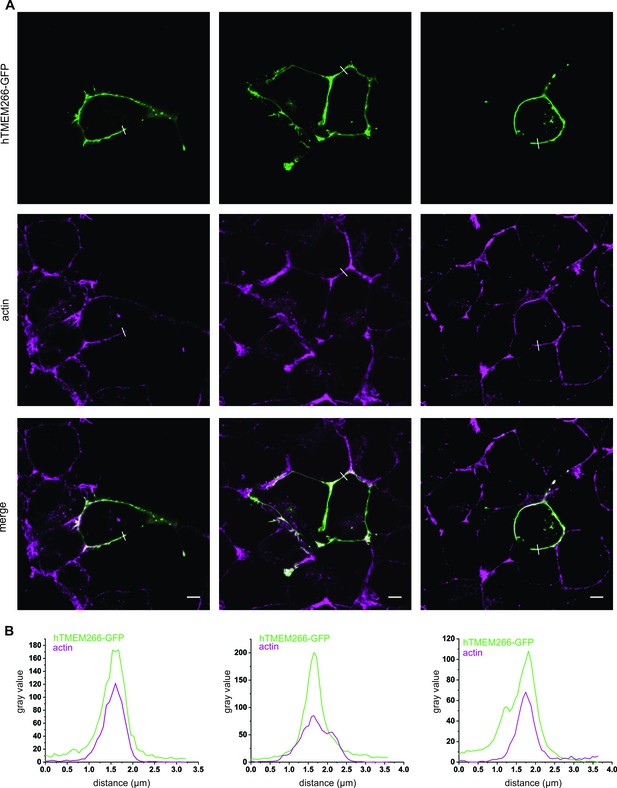
hTMEM266 efficiently traffics to the plasma membrane of HEK 293 cells.
(A) Confocal microscopy images of HEK 293 cells expressing hTMEM266-GFP (green) counterstained with phalloidin to label F-actin (magenta). Scale bar represents 5 µm. (B) Intensity profiles of hTMEM266-GFP (green) and F-actin (magenta) at the edge of three different HEK 293 cells measured along the indicated white lines shown in A.
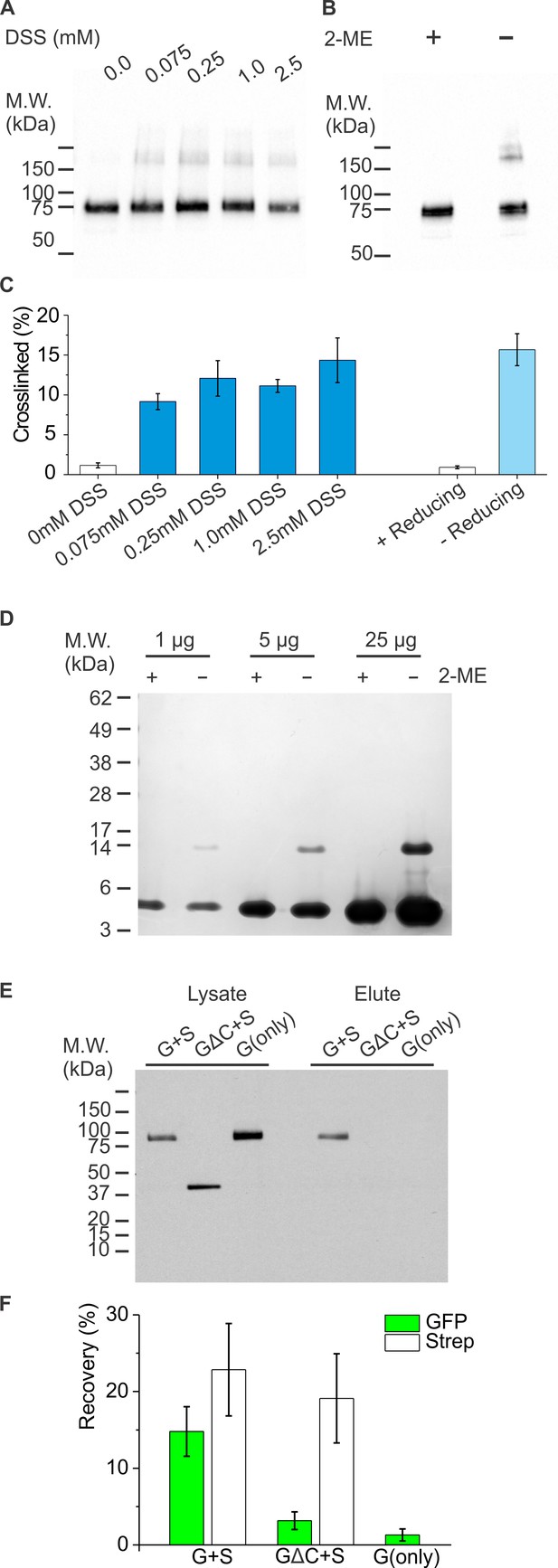
Oligomerization of hTMEM266.
(A) Western blots of membrane fractions of HEK 293 T cells expressing C-terminal twin-Strep-tagged hTMEM266 treated with varying concentration of DSS prior to cell lysis, resolution on SDS-PAGE and probing with anti-Strep antibody. (B) Western blots of membrane fractions of HEK 293 T cells expressing C-term Strep-tagged hTMEM266 subjected to air oxidation and resolved on SDS-PAGE with or without 2-ME treatment prior to probing with anti-Strep antibody. (C) Average extent of crosslinking quantified using Image Lab (Biorad). For DSS crosslinking n = 3–9 from four separate experiments. For air oxidation n = 6 from three separate experiments in the presence of 2-ME and n = 16 from eight separate experiments in the absence of 2-ME. Error bars represent SEM. (D) SDS-PAGE profile of the purified C-terminal coiled-coil of hTMEM266 (residues 224–289; M.W. – 7.8 kDa) with or without 2-ME treatment. Although the protein band in the presence of 2-ME (corresponding to the monomer) runs below the expected M.W. standard, its molecular mass was verified by mass spectrometry to be correct (data not shown). The appearance of an additional higher M.W. band at ~15 kDa in the absence of 2-ME indicates the presence of a disulfide mediated dimer. (E) Western blots of SDS-PAGE gels measuring heterotypic pulldown of hTMEM266. HEK 293 T cells were transfected with variants of N-terminal GFP-tagged (G) and C-terminal twin-Strep-tagged (S) hTMEM266 constructs. G∆C denotes G with a deletion of the C-terminus generated by introducing a stop codon after Leu-225. Lysed cells were applied to Streptactin-coated beads, washed, and eluted. Western blot probed with anti-GFP shows cell lysates and corresponding pulldowns (elute) for cells expressing both G and S, GΔS and S or G alone. The GFP signal is comparable in all lysate lanes, but only full length GFP-hTMEM266 (G) is enriched in the elute lanes when expressed along with S. Expected MWs are 61 kDa for S, 85 kDa for G and 53 kDa for G∆C. (F) Quantification of average percent recovery calculated from intensities of the elute band compared to that of the lysate band (n = 10 to 13 replicates from four independent experiments). Error bars represent SEM.
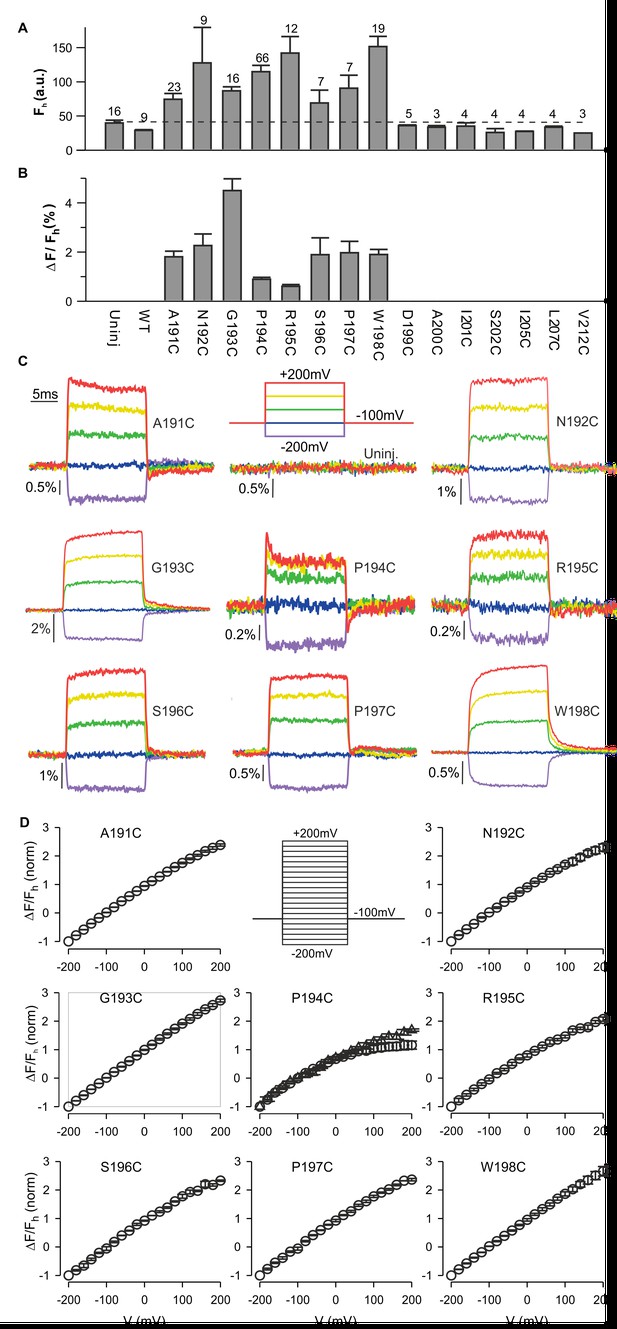
Fluorescence responses from hTMEM266 labeled at the external ends of the S3 and S4 helices.
(A) Average fluorescence intensities (Fh, arbitrary units) of TAMRA-MTS labeled oocytes expressing hTMEM266 without (WT) or with Cys substitutions at different positions between the external ends of S3 and S4. Holding voltage was −100 mV. Dashed line represents the average fluorescence intensity recorded from uninjected oocytes (Uninj). Number of oocytes is indicated above each bar. (B) Average change in fluorescence intensity (ΔF/Fh) for all positions studied. ΔF is the change in fluorescence intensity for a voltage step from −100 mV to +200 mV and Fh is the fluorescence intensity at −100 mV. The number of oocytes is indicated above the corresponding bar in A. (C) Representative fluorescence signals recorded from uninjected oocytes and oocytes expressing Cys mutants of hTMEM266 after labeling with TAMRA-MTS. Voltage protocol with color code is shown in the top middle panel. (D) Normalized average fluorescence-voltage (ΔF/Fh-V) relations for labeled constructs (n = 3 oocytes). ΔF/Fh was normalized to the value measured at −200 mV. Circles represent ΔFe/F where ΔFe is the difference between the average fluorescence at the end (last 5 ms) of the depolarization step and the baseline fluorescence at −100 mV. For the P194C panel triangles represent ΔFi/F where ΔFi is the difference between the baseline fluorescence at −100 mV and the initial fluorescence at the beginning of the depolarization step. In all panels error bars represent SEM.
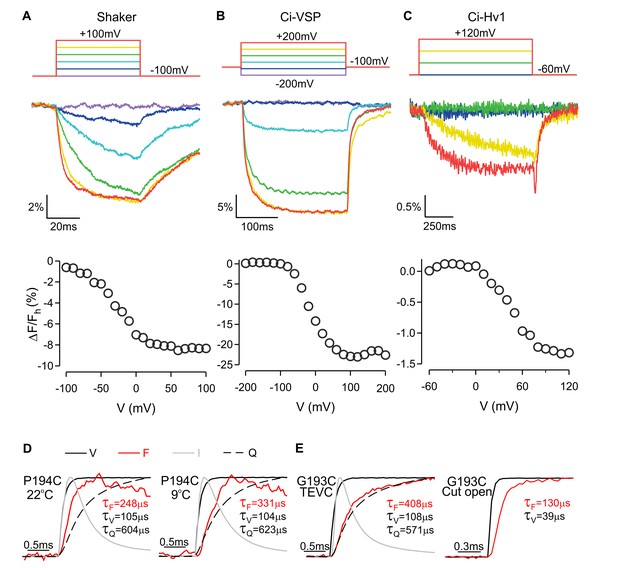
Fluorescence responses for Shaker, Ci-VSP, Ci-Hv1 and hTMEM266.
(A) Fluorescence response to voltage steps and ΔF/Fh-V relation recorded from an oocyte expressing Shaker A359C/W434F labeled with TMRM-maleimide. (B) Fluorescence response to voltage steps and ΔF/Fh-V relation recorded from an oocyte expressing Ci-VSP ArcLight-A242C (Jin et al., 2012). (C) Fluorescence response to voltage steps and ΔF/Fh-V relation recorded from an oocyte expressing and Ci-Hv1 S242C labeled with Alexa Fluor488-maleimide. (D) Normalized responses recorded from oocytes expressing hTMEM266 P194C at 22⁰C (left panel) and 9⁰C (right panel). Solid black lines are the recorded membrane voltage, solid red lines are the fluorescence signal, and solid gray lines are capacitive transients recorded without capacitive compensation. Dashed line represents the charge (Q) calculated by integrating the capacitive transients. τF, τV, and τQ are time constants obtained from fits of a single exponential function to the rising phase of fluorescence (F), membrane voltage (V) and charge (Q). (E) Normalized responses recorded from oocytes expressing hTMEM266 G193C using two-electrode voltage clamp (left panel) or the cut-open oocyte technique (right panel). Color coding and the definition of the time constants are the same as in (D).
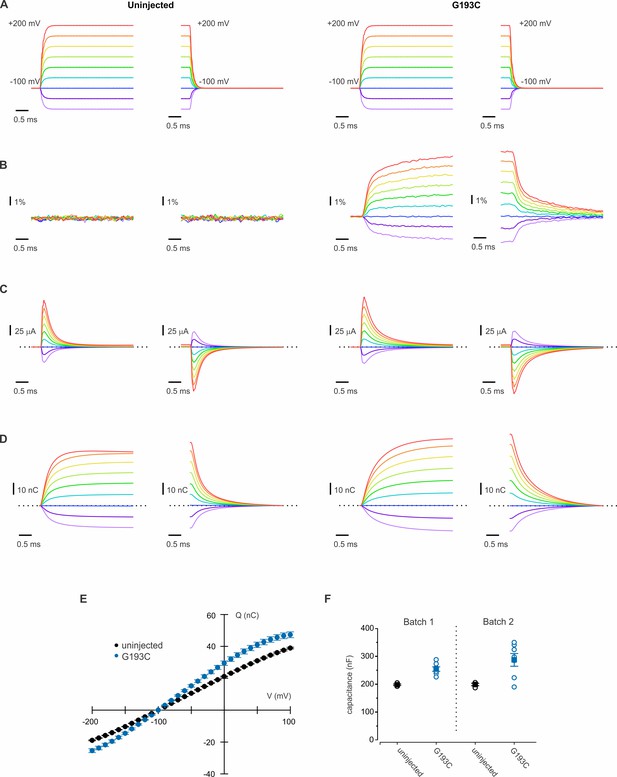
Transient currents from oocytes expressing hTMEM266.
(A–D) Examples of the voltage (A), fluorescence (B), current (C) and integrated charge (D) measured during two-electrode voltage-clamp recordings from a control un-injected oocyte (left) and oocyte expressing the hTMEM266/G193C labeled with TAMRA (right). Oocytes were held at −100 mV and every 5 s the voltage was stepped for 15 ms to values between −200 mV and +200 mV. (E) Average charge transferred, Q, as a function of voltage, V, for control un-injected oocytes (n = 8) and oocytes expressing G193C (n = 14). Q was determined by integrating the capacitive current for the first 2 ms of each voltage step. Error bars are SEM. (F) Apparent capacitance for control un-injected oocytes and oocytes expressing hTMEM266/G193C. Results are presented for two independent batches of oocytes and the mean (filled circles) and SEM (error bars) for each group are plotted in addition to the individual capacitance values (open circles).
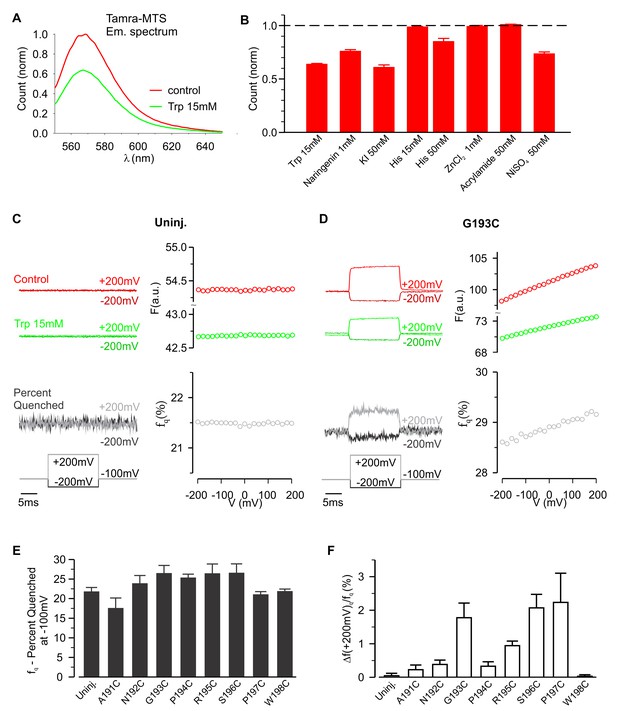
Voltage-dependent tryptophan quenching of TAMRA-labeled hTMEM266 constructs.
(A) Quenching of TAMRA-MTS fluorescence by tryptophan (15 mM) in aqueous solution. (B) Normalized average solution TAMRA-MTS fluorescence in the presence of potential quenchers or modulatory ions. (C) Effect of 15 mM tryptophan on fluorescence from an uninjected oocyte labeled with TAMRA-MTS. Examples of voltages steps from −100 mV to −200 mV or +200 mV are shown on the left, with the fluorescence intensity in the absence (red) and presence (green) of 15 mM tryptophan plotted above the resulting percent of fluorescence quenched (fq, grey). The voltage dependence of the fluorescence and fluorescence quenching are plotted on the right. (D) Effect of 15 mM tryptophan on fluorescence from an hTMEM266 G193C expressing oocyte labeled with TAMRA-MTS. (E) Average percent of fluorescence quenched by 15 mM tryptophan, fq, at the holding voltage (−100 mV) for TAMRA-MTS labeled hTMEM266 constructs with Cys substituted at the external ends of S3 and S4, as well as for uninjected oocytes (n = 4 to 7 oocytes). (F) Average voltage-dependent change in quenching at +200 mV, Δfq(+200 mV)/fq(−100 mV), for TAMRA-MTS labeled hTMEM266 constructs with Cys substituted at the external ends of S3 and S4, as well as for uninjected oocytes (n = 4 to 7 oocytes). See Figure 4—figure supplement 1 for primary data for positions other than G193C. In all panels error bars represent SEM.
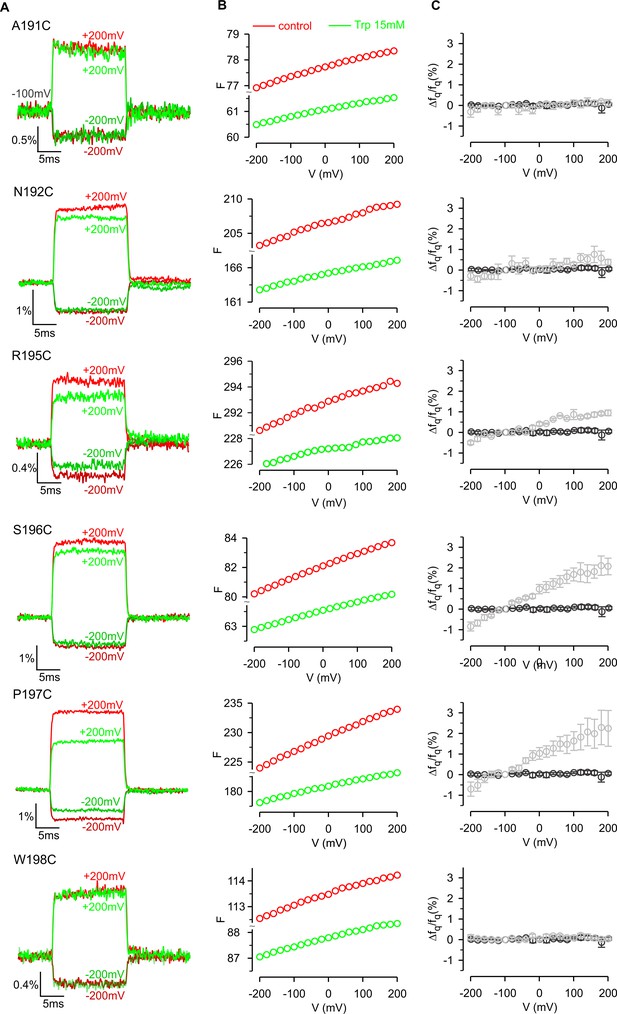
Voltage-dependent tryptophan quenching of TAMRA-labeled Cys- substituted hTMEM266 for different positions at the external sides of the S3 and S4 helices.
(A) Fluorescence responses to voltage steps before (red) and after application of tryptophan (15 mM; green) recorded from Cys-substituted hTMEM266 expressing oocytes labeled with TAMRA-MTS. Fluorescence traces are overlaid to match baseline fluorescence at −100 mV. (B) F-V relations recorded before (red) and after application of tryptophan (15 mM; green) for Cys-substituted hTMEM266 expressing oocytes labeled with TAMRA-MTS. (C) Voltage dependence of the average fluorescence quenched by tryptophan, Δfq/fq, for Cys-substituted hTMEM266 expressing oocytes (grey; n = 4 to 7 oocytes). Results for uninjected oocytes labeled with TAMRA-MTS are shown for comparison (black circles; n = 5). Error bars represent SEM.
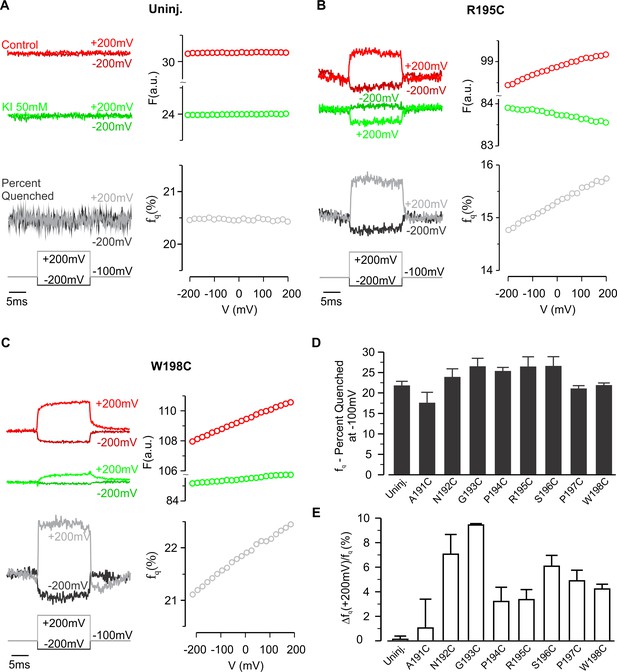
Iodide quenching of TAMRA-labeled hTMEM266.
(A) Fluorescence responses to voltage steps from −100 mV to −200 mV or +200 mV before (red) and after extracellular application of KI (50 mM; green) recorded from an uninjected oocyte labeled with TAMRA-MTS. The resulting percent of fluorescence quenched (fq, grey) is shown below, and the voltage dependence of the fluorescence and its quenching are plotted on the right. (B) Effect of 50 mM KI on fluorescence from an hTMEM266 R195C expressing oocyte labeled with TAMRA-MTS. In contrast to the control solution, fluorescence decreases with voltage when 50 mM KI is present. (C) Effect of 50 mM KI on fluorescence from an hTMEM266 W198C expressing oocyte labeled with TAMRA-MTS. (D) Average percent of fluorescence quenched by 50 mM KI, fq, at the holding voltage (−100 mV) for TAMRA-MTS labeled hTMEM266 constructs with Cys substituted at the external ends of S3 and S4, as well as for uninjected oocytes (n = 4 to 7 oocytes). (E) Average voltage-dependent change in iodide quenching, Δfq(+200 mV)/fq(−100 mV), for TAMRA-MTS labeled hTMEM266 constructs with Cys substituted at the external ends of S3 and S4, as well as for uninjected oocytes (n = 4 to 7 oocytes). See Figure 4—figure supplement 3 for primary data for most positions studied. For panels D and E, error bars represent S.
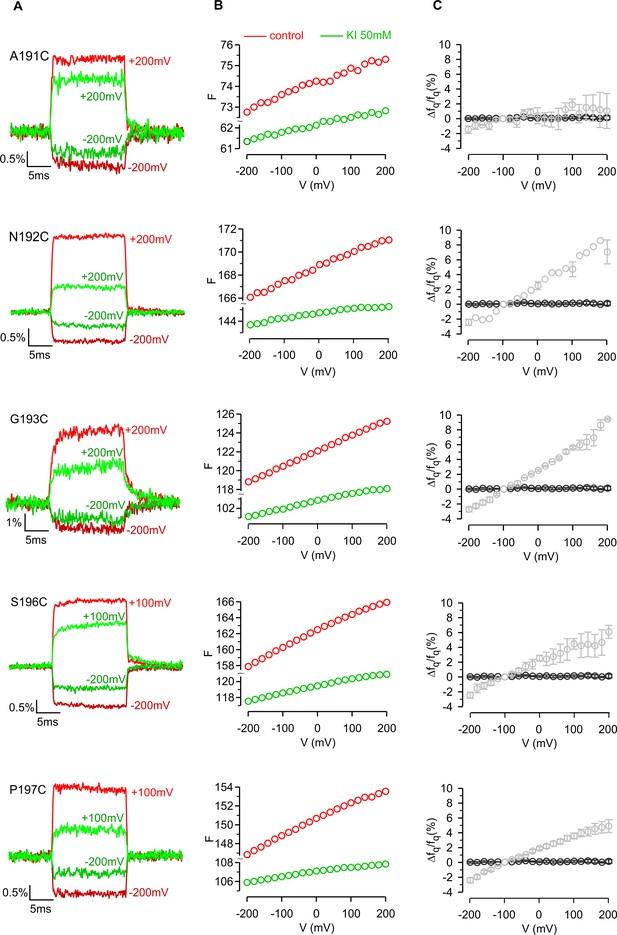
Iodide quenching of TAMRA attached to hTMEM266 at different positions on the external ends of the S3 and S4 helices.
(A) Fluorescence responses to voltage steps before (red) and after application of KI (50 mM; green) recorded from Cys-substituted hTMEM266 expressing oocytes labeled with TAMRA-MTS. Fluorescence traces are overlaid to match baseline fluorescence at −100 mV. (B) F-V relations recorded before (red) and after application of KI (50 mM; green) for Cys-substituted hTMEM266 expressing oocytes labeled with TAMRA-MTS. (C) Average voltage-dependence of quenching (Δfq/fq) by 50 mM KI (n = 3 to 4 oocytes). Results for uninjected oocytes labeled with TAMRA-MTS are shown for comparison (black circles; n = 4 oocytes). Error bars represent SEM.
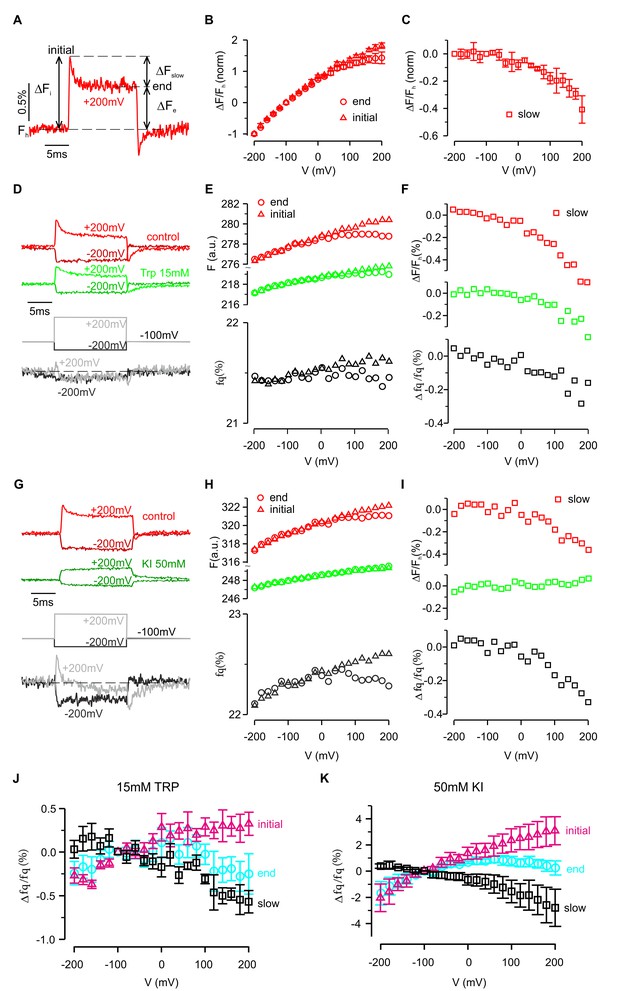
Fluorescence and quenching of hTMEM266 labeled with TAMRA-MTS at P194C.
(A) Representative fluorescence response to a voltage step from −100 mV to +200 mV for a hTMEM266 P194C expressing oocyte labeled with TAMRA-MTS. Fh is the fluorescence at the holding voltage of −100 mV, ΔFi is the initial change in fluorescence at the beginning of the voltage step, ΔFe is change in fluorescence at the end of the voltage step, and ΔFslow is the change in fluorescence during the voltage pulse (ΔFslow = ΔFi – ΔFe). (B) Average ΔF/Fh as a function of voltage normalized to the value of ΔF/Fh at −200 mV. Triangles show the initial change, ΔFi/Fh, and circles represented the change and the end of the voltage step, ΔFe/Fh (n = 3 oocytes). (C) Average slow fluorescence intensity change during the voltage step, ΔFslow/Fh, as a function of membrane voltage (n = 3 oocytes). (D–F) Voltage dependent fluorescence quenching by 15 mM tryptophan. Examples of voltages steps from −100 mV to −200 mV or +200 mV are shown in panel D, with the fluorescence intensity in the absence (red) and presence (green) of 15 mM tryptophan plotted above the resulting percent of fluorescence quenched (fq, grey). Panel E shows the initial (triangles) and final (circle) fluorescence intensity during each voltage step in control (red) and 15 mM Trp (green) along with the corresponding percent of fluorescence quenched by tryptophan (grey). Finally, the magnitude of the slow component of the fluorescence (ΔFslow/Fh) in control (red) and 15 mM Trp (green), along with the resulting quenched fraction (Δfq/fq) is shown in F. (G–I) Voltage dependent quenching by 50 mM KI. (J) The average initial (triangles), end (circles) and slow change (squares) in fluorescence quenching by 15 mM tryptophan as a function of voltage (n = 7 oocytes). (K) The average initial (triangles), end (circles) and slow change (squares) in fluorescence quenching by 50 mM Iodide as a function of voltage (n = 5 oocytes). In all panels error bars represent SEM.
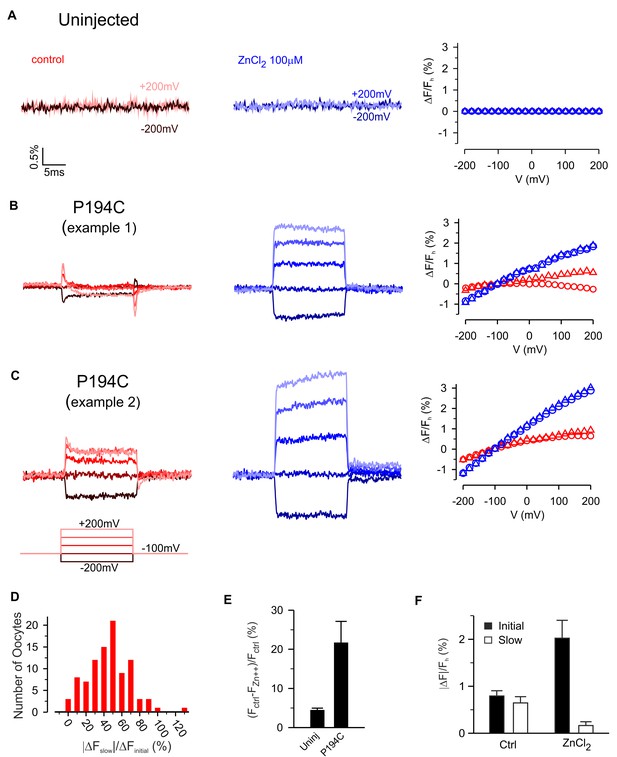
Zn2+ modulation of fluorescence from hTMEM266 labeled with TAMRA-MTS at P194C.
(A) Fluorescence from an uninjected oocyte labeled with TAMRA-MTS as voltage steps are first applied in control solution (red) and then in the presence of extracellular ZnCl2 (blue). The voltage dependence of the fluorescence, ΔF/Fh, is shown to the right. (B,C) Two examples of fluorescence from oocytes expressing hTMEM266 P194C labeled with TAMRA-MTS during voltage steps in control solution (red) and with ZnCl2 (blue). The rapid initial change in fluorescence intensity during the first 1 ms after the voltage step (triangles) and the average value during the last 5 ms (circles) of each voltage step are plotted on the right. (D) Histogram illustrating how the size of the slow fluorescence change during the voltage step (ΔFslow) relative to the rapid initial change (ΔFinitial) varies between oocytes. Both slow and initial components were evaluated for a step to +200 mV. (E) Average reduction in baseline fluorescence intensity after applying 100 µM ZnCl2, (Fctrl – FZn2+)/Fctrl, for uninjected oocytes (n = 3) and oocytes expressing P194C (n = 7). (F) Average magnitude of the initial (dark) and slow (light) fluorescence intensity changes for oocytes expressing P194C following depolarization to +200 mV in control solution and 100 µM ZnCl2 (n = 5 oocytes). In all panels error bars represent SEM.
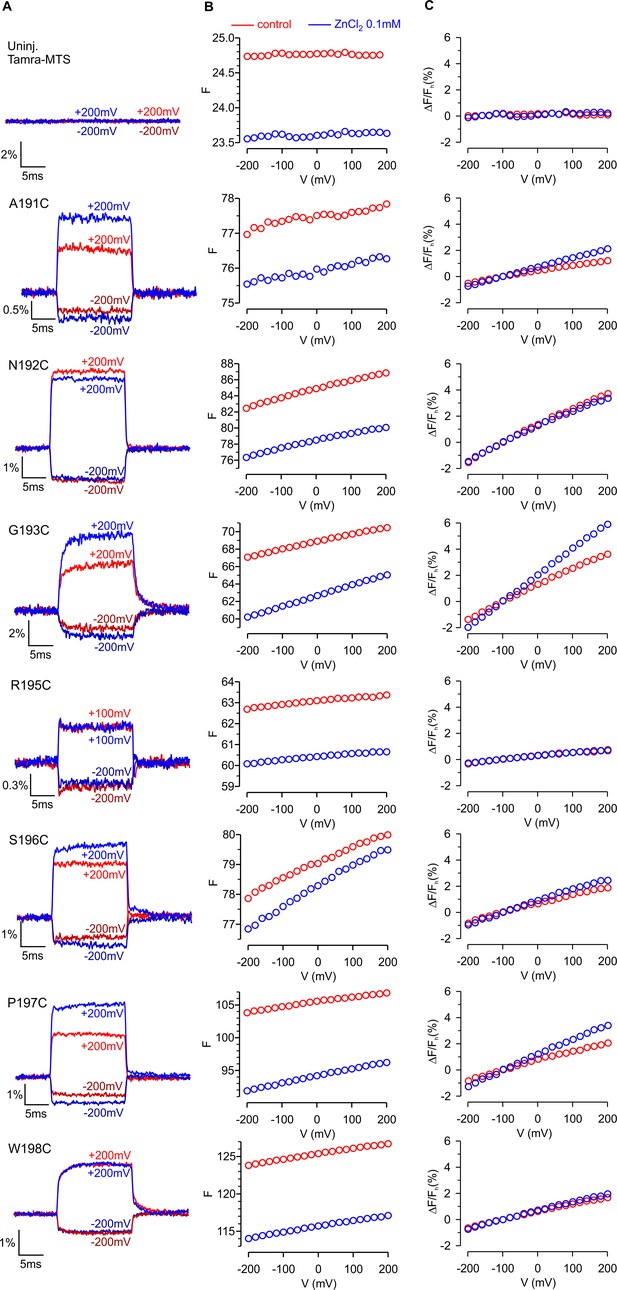
Modulation of fluorescence responses by Zn2+ for hTMEM266 labeled with TAMRA-MTS at different positions on the external ends of the S3 and S4 helices.
(A) Examples of the fluorescence intensity during voltage steps from −100 mV to either −200 or +200 mV in control solution (red) and after extracellular application of ZnCl2 (blue). Traces have been normalized by the fluorescence intensity at the holding voltage, Fh. (B) Fluorescence intensity (arbitrary units) at the end of a step depolarization as a function of voltage for oocytes labeled with TAMRA-MTS in control (red) and after ZnCl2 (blue). Fe was measured as the average F during the last 5 ms of the step. (C) ΔFe/Fh as a function of voltage for oocytes labeled with TAMRA-MTS in control (red) and after ZnCl2 (blue normalized so that ΔFe(−200 mV)/Fh = −1 for the control solution).
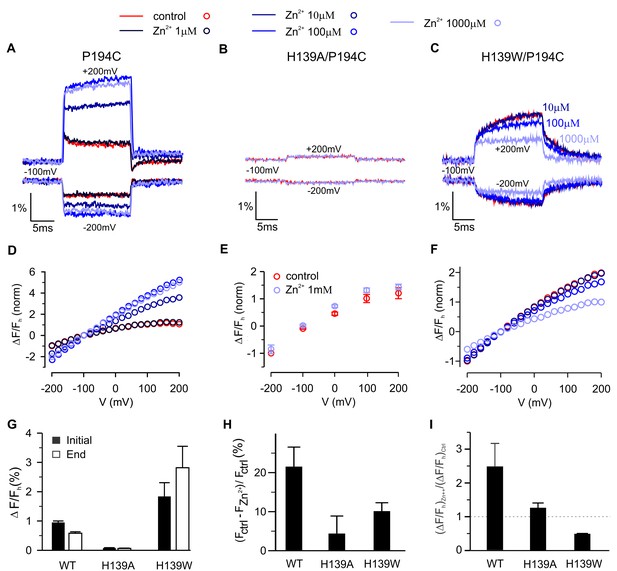
Contribution of H139 to Zn2+sensitivity of hTMEM266 labeled with TAMRA-MTS at P194C.
(A–C) Fluorescence intensity during voltage steps from −100 mV to ±200 mV for oocytes expressing hTEME266 P194C (A), the double mutant H139A/P194C (B), and the double mutant H139W/P194C (C) for control and zinc-containing solutions. (D–F) Voltage dependence of the fluorescence change at the end of the voltage pulse (ΔFe/Fh) for P194C (D), H139A/P194C (E), and H139W/P194C (F). To permit comparison between the different constructs, the data from individual oocytes were normalized so that ΔFe(−200 mV)/Fh = −1 in control solution. (G) Average change in fluorescence (ΔFe/Fh), at the beginning (black) and end (white) of a step to +200 mV in control solution for P194C (n = 4 oocytes), H139A/P194C (n = 3 oocytes), and H139W/P194C (n = 3 oocytes). (H) Average decrease in baseline fluorescence at −100 mV, (Fctrl – FZn2+)/Fctrl, upon switching from control to 1 mM Zn2+ solution. (I) Effect of 1 mM Zn2+ on the average change in fluorescence at the end of a step to +200 mV. For each construct the average change in a 1 mM Zn2+ solution (ΔFe/F)Zn2+ is normalized by the average change in control solution (ΔFe/F)ctrl (n = 4 oocytes for P194C, n = 3 oocytes for P194C/H139A, n = 3 oocytes for P194C/H139W). In all panels error bars represent SEM.
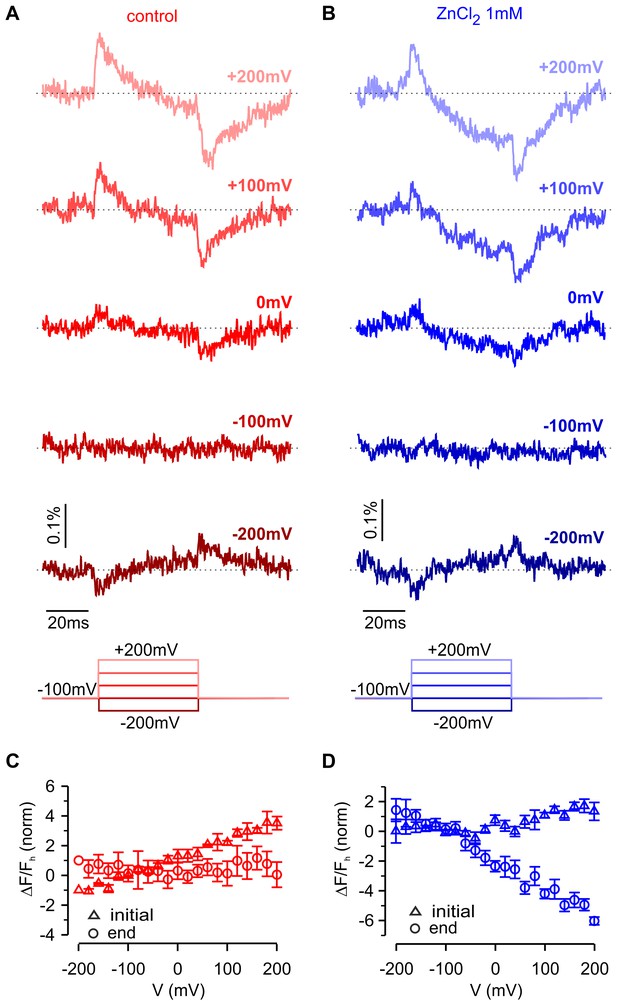
Fluorescence responses and modulation by Zn2+ for hTMEM266 tagged with super ecliptic pHluorin GFP at the C-terminus of the S4 helix.
(A,B) Fluorescence responses during voltage steps for an oocyte expressing hTMEM266-EGFP in control solution (red, (A) and after extracellular application of ZnCl2 (blue, (B). (C,D) Average change in fluorescence (ΔF/Fh) at the start (triangles) and end (circles) of voltage steps for the control solution (red, (C) and after application of ZnCl2 (blue, (D). For each oocyte ΔF/Fh was normalized to the value measured at −200 mV in control solution (n = 3 oocytes). In all panels error bars represent SEM.
Additional files
-
Transparent reporting form
- https://doi.org/10.7554/eLife.42372.020