A type III-A CRISPR-Cas system employs degradosome nucleases to ensure robust immunity
Figures
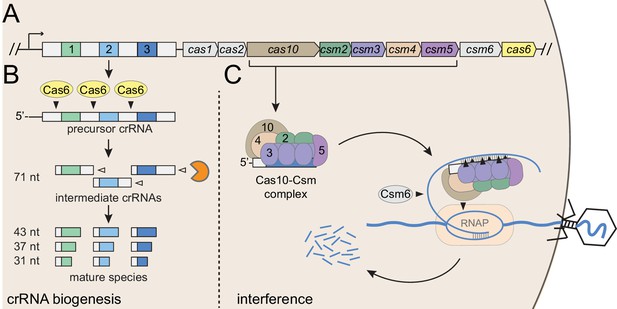
Type III-A CRISPR-Cas defense in S. epidermidis RP62a.
(A) The type III-A CRISPR-Cas system in S. epidermidis (known as CRISPR-Cas10) encodes three spacers (colored squares), four repeats (white squares) and nine CRISPR-associated (cas and csm) genes. (B) During crRNA biogenesis, the repeat-spacer array is transcribed into a precursor crRNA, which is subsequently processed in two steps. First, the Cas6 endoribonuclease cleaves within repeat sequences to yield intermediate crRNAs that are ~71 nucleotides in length. These intermediates are then subjected to a second maturation step in which their 3’-ends are trimmed to generate mature crRNAs that range from 31 to 43 nucleotides in length. Mature crRNAs combine with Cas10, Csm2, Csm3, Csm4, and Csm5 to form the Cas10-Csm complex. (C) Interference is triggered when the Cas10-Csm complex binds foreign transcripts that bear complementarity to the crRNA. During interference, invading DNA and RNA are destroyed--while RNA is shredded by each Csm3 subunit in the complex, the DNA coding strand is cleaved by Cas10. An accessory nuclease, Csm6, also participates in the immune response by degrading invader-derived transcripts. Filled triangles indicate cleavage events catalyzed by CRISPR-associated proteins, and open triangles indicate cleavage events catalyzed by non-Cas nucleases. RNAP, RNA polymerase.
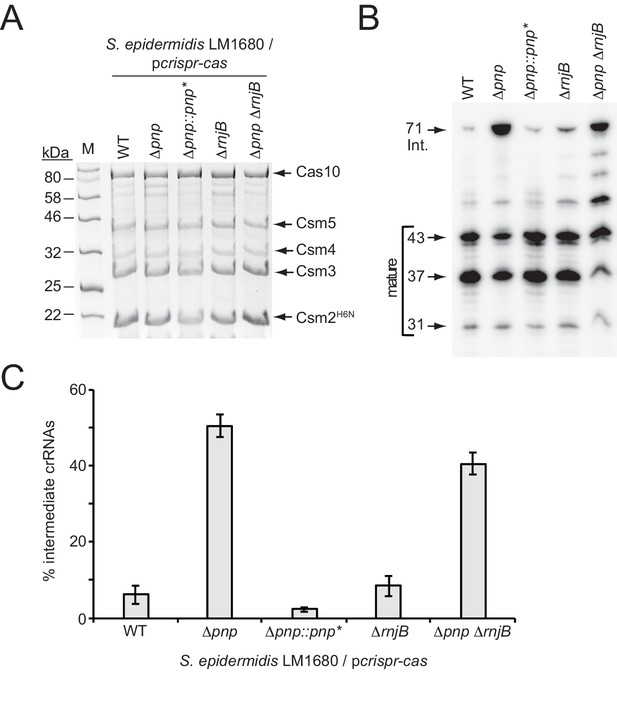
PNPase promotes crRNA maturation.
(A) Cas10-Csm complexes extracted from various strains of S. epidermidis LM1680/pcrispr-cas are shown. The plasmid pcrispr-cas encodes the entire CRISPR-Cas10 system with a 6-Histidine tag on the N-terminus of Csm2 (Csm2H6N). Whole cell lysates from indicated strains bearing this plasmid were subjected to Ni2+ affinity chromatography, and purified complexes were resolved and visualized using SDS-PAGE followed by Coomassie G-250 staining. Δpnp::pnp* is a complementation strain in which the Δpnp strain received a variant of the pnp gene containing five silent mutations (pnp*, see Figure 2—figure supplement 2 for details). (B) Total crRNAs associated with Cas10-Csm complexes purified from indicated S. epidermidis LM1680 strains are shown. crRNAs were extracted from the complexes using trizol reagent, radiolabeled on their 5’-ends, and resolved on a denaturing urea-PAGE gel. The gel was exposed to a storage phosphor screen and screens were imaged using a Typhoon phosphor imager. (C) Fractions of complex-associated intermediate crRNAs relative to total crRNA content are shown for each strain. Complex-associated crRNAs were analyzed with ImageQuant software to determine band densities. The percent of intermediate crRNAs was calculated as the ratio of the 71 nt band density to the sum of band densities of the major crRNA species (71, 43, 37, and 31 nt). The data represent an average of 3–5 independent trials (±S.D). Specific numbers of replicates are as follows: WT and Δpnp, five replicates; ΔrnjB, four replicates; Δpnp::pnp* and ΔpnpΔrnjB, three replicates each (see Figure 2—source data 1).
-
Figure 2—source data 1
Accompanies Figure 2C.
Percentages of intermediate crRNAs extracted from indicated S. epidermidis LM1680 strains bearing pcrispr-cas.
- https://doi.org/10.7554/eLife.45393.007
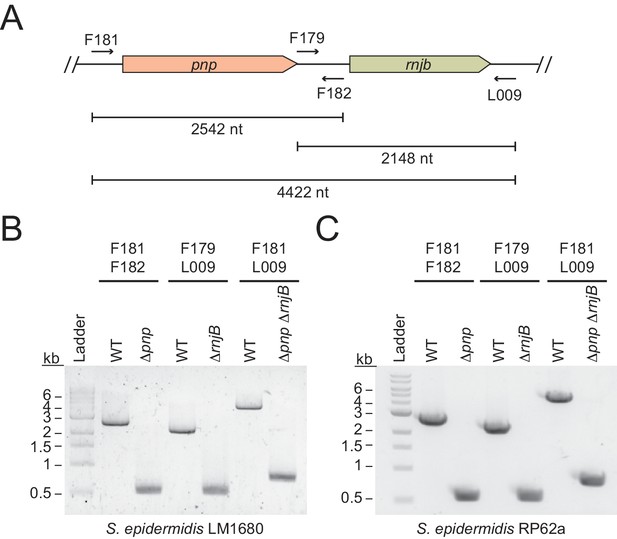
Confirmation of pnp and rnjB knockouts.
(A) Illustration of the pnp and rnjB genomic loci and corresponding primers used to amplify these regions (see Supplementary file 1 for primer sequences). The genes were deleted from S. epidermidis using the pKOR1 allelic replacement system (Bae et al). Following mutagenesis, deletions were confirmed by amplifying genomic DNA using indicated primers. (B) and (C) PCR products from indicated S. epidermidis LM1680 (B) and RP62a (C) strains are shown.
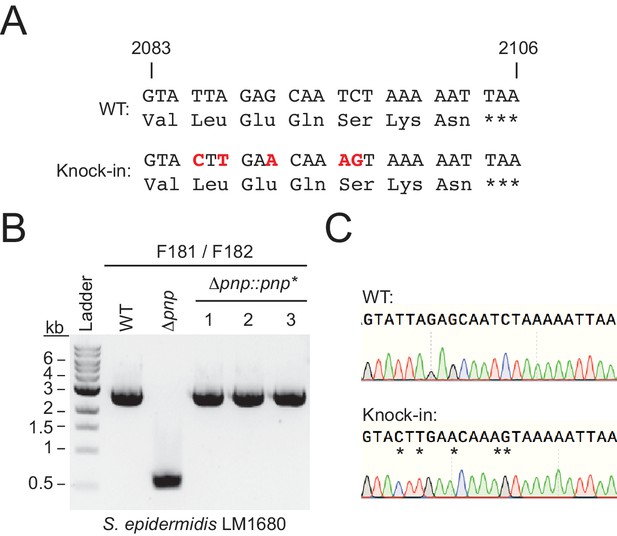
Confirmation of pnp knock-in strain.
(A) A segment of the coding region of PNPase into which silent mutations were introduced (red nucleotides) in order to differentiate the knock-in strain from the original wild-type. The pnp gene bearing these mutations was replaced into the Δpnp strain in its original genetic locus using the pKOR1 allelic replacement system (Bae and Schneewind, 2006). Following mutagenesis, the insertion was confirmed by amplifying genomic DNA using primers F181 and F182 (see Supplementary file 1 for primer sequences). (B) PCR products from the indicated S. epidermidis LM1680 strains which confirm an insertion was introduced. (C) Representative sequence trace of a PCR product confirming the presence of the silent mutations.
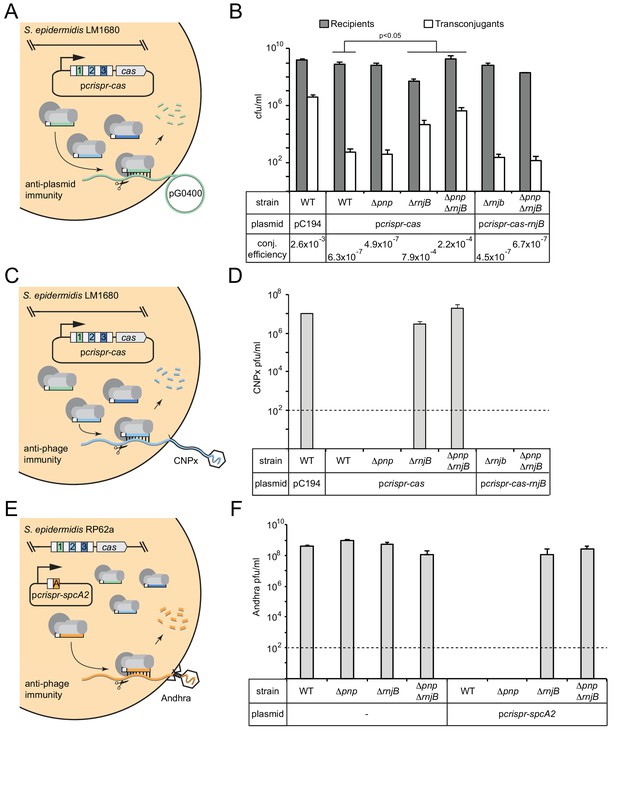
RNase J2 is essential for CRISPR-Cas10 immunity against plasmid and phage.
(A) Illustration of the conjugation assay used to test CRISPR-mediated anti-plasmid immunity. In this assay, the conjugative plasmid pG0400 is transferred from a S. aureus RN4220 donor (not shown) into S. epidermidis LM1680 recipient strains containing pcrispr-cas, in which the first spacer (green square) bears complementarity to pG0400. (B) The indicated recipient strains harboring either pC194 (empty vector), pcrispr-cas, or pcrispr-cas-rnjB (which encodes rnjB downstream of the CRISPR-Cas10 system) were mated with S. aureus RN4220/pG0400. Cells were subsequently plated on selective media to enumerate S. epidermidis recipients and transconjugants. Conjugation (conj.) efficiencies were determined as the average numbers of transconjugants/recipients. Shown is a representative of 2–9 independent trials (see Figure 3—source data 1 for exact replicate numbers). The significant differences between conjugation efficiencies observed for the wild-type strain in comparison to the ΔrnjB and ΔpnpΔrnjB mutants are indicated (t-test, one-tailed, p=0.04 and 0.02, respectively). (C) Illustration of the phage challenge assay used to test CRISPR-mediated anti-phage immunity in S. epidermidis LM1680. In this assay, dilutions of phage CNPx are plated atop lawns of cells containing pcrispr-cas, in which the second spacer (light blue square) bears complementarity to the phage genome. (D) The various strains containing indicated plasmids were challenged with CNPx and the resulting plaque forming units per milliliter (pfu/ml) were enumerated. (E) Illustration of the assay to test CRISPR-mediated anti-phage immunity in S. epidermidis RP62a. In this assay, the genome-encoded CRISPR-Cas10 system works together with a spacer encoded in the plasmid pcrispr-spcA2 (orange square) to protect against phage Andhra. (F) The indicated strains were challenged with Andhra and the resulting pfu/ml were enumerated. For both phage challenge assays, the data shown is an average (±S.D.) of three technical replicates as a representative of several independent trials (see source data files). Dotted lines indicate the limits of detection for these assays.
-
Figure 3—source data 1
Accompanies Figure 3B.
Recipients, transconjugants, and conjugation efficiencies measured for various S. epidermidis LM1680 strains harbouring indicated plasmids.
- https://doi.org/10.7554/eLife.45393.009
-
Figure 3—source data 2
Accompanies Figure 3D.
CNPx plaque counts in pfu/mL when plated on various S. epidermidis LM1680 strains harbouring indicated plasmids.
- https://doi.org/10.7554/eLife.45393.010
-
Figure 3—source data 3
Accompanies Figure 3F.
Andhra plaque counts in pfu/mL when plated on various S. epidermidis RP62a strains harbouring indicated plasmids.
- https://doi.org/10.7554/eLife.45393.011
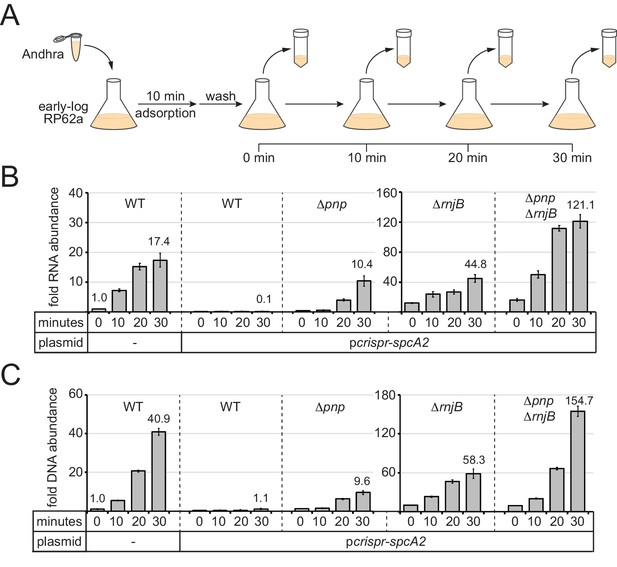
PNPase and RNase J2 are required for efficient CRISPR-mediated clearance of invading nucleic acids.
(A) Diagram of the phage infection assay used to track the accumulation of phage-derived nucleic acids over time. In this assay, early-log S. epidermidis RP62a cells were challenged with phage Andhra in a 2:1 (bacteria:phage) ratio. Following a 10 min adsorption period, cells were washed to remove unadsorbed phages. A fraction of infected cells was harvested immediately after the wash (time = 0) and every 10 min thereafter for 30 min. Harvested cells were heat-killed and subjected to RNA or DNA extraction. (B) The abundance of phage RNA was quantified for indicated strains at each time point using quantitative reverse-transcriptase PCR (qRT-PCR). Total RNA extracts were reverse-transcribed using primers for the phage-encoded DNA polymerase gene (gp09) as well as the host-encoded gene for glyceraldehyde-3-phosphate dehydrogenase (gap). A fraction of the resulting cDNA mix was used as a template in the qPCR reaction. Phage cDNA copy number was normalized against host cDNA values, and the normalized value for the 0 min time point in wild-type cells lacking pcrispr-spcA2 was set to one to determine the relative abundance for the rest of the time points. (C) The abundance of phage DNA was quantified for indicated strains at each time point using quantitative PCR (qPCR). Total DNA extracts were subjected to qPCR using primers complementary to the phage gp09 gene and host gap gene. Phage DNA copy number was normalized against host values, and the normalized value for the 0 min time point in wild-type cells lacking pcrispr-spcA2 was set to one to obtain the relative DNA abundance for the rest of the time points. For both qRT-PCR and qPCR, the mean ±S.D. of triplicate measurements are shown as a representative of at least two independent trials (see source data files).
-
Figure 4—source data 1
Accompanies Figure 4B.
Relative phage RNA abundance measured in indicated S. epidermidis RP62a strains following qRT-PCR analysis.
- https://doi.org/10.7554/eLife.45393.015
-
Figure 4—source data 2
Accompanies Figure 4C.
Relative phage DNA abundance measured in indicated S. epidermidis RP62a strains following qPCR analysis.
- https://doi.org/10.7554/eLife.45393.016
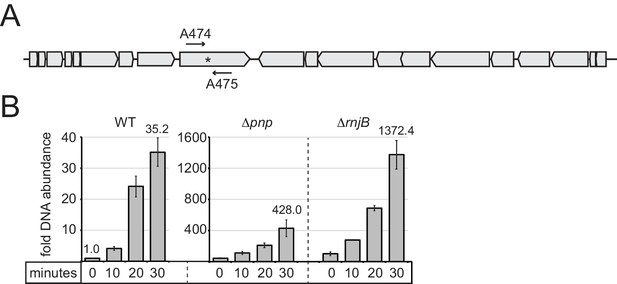
Accumulation of phage DNA in RP62a strains in the absence of CRISPR-Cas10 immunity.
(A) A schematic of phage Andhra’s genome. The region targeted by spcA2 within the gp9 gene is shown (asterisk), as well as the phage-specific primers that were used for qPCR and qRT-PCR, A474 and A475 (Supplementary file 1). (B) Indicated S. epidermidis RP62a strains were challenged with Andhra and cells were harvested at various time points (0, 10, 20, and 30 min) post-infection (refer to the diagram in Figure 4A for details). Total DNA was extracted, and phage DNA accumulation was quantified with qPCR using two sets of primers: A474/A475, as well as genome-specific primers S001/S002 (Supplementary file 1) for normalization. Phage DNA copy number was normalized against host values, and the normalized value for the 0 min time point in wild-type cells was set to one to obtain the relative DNA abundance for the rest of the time points. The mean ±S.D. of duplicate measurements are shown as a representative of two independent trials (see Figure 4—figure supplement 1—source data 1).
-
Figure 4—figure supplement 1—source data 1
Accompanies Figure 4—figure supplement 1.
Relative phage DNA abundance measured in indicated S. epidermidis RP62a strains following qPCR analysis.
- https://doi.org/10.7554/eLife.45393.014
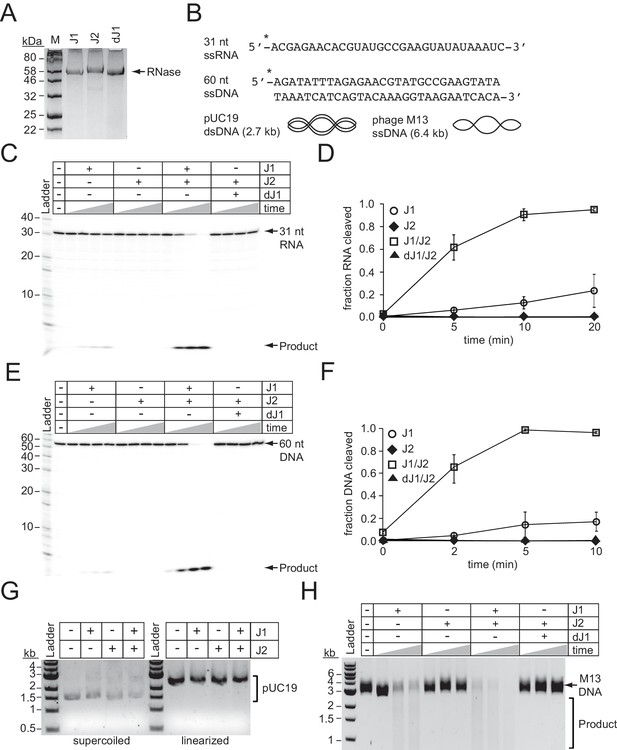
RNase J2 promotes RNase and DNase activities of RNase J1.
(A) Purified recombinant ribonucleases J1, J2, and a catalytically-dead variant of J1 bearing H74A and H76A mutations (dJ1). Proteins were resolved by SDS-PAGE and visualized using Coomassie G-250 staining. (B) RNA and DNA substrates used in nuclease assays. (C) Ribonuclease activities of RNases J1 and J2. The 5’-end labelled 31-nucleotide RNA substrate was combined with 0.5 pmols of indicated enzyme(s). The reaction mixture was incubated at 37°C for increasing time points (0, 5, 10, or 20 min.) RNAs were resolved using denaturing urea-PAGE. (D) Quantification of ribonuclease activity. Shown is the average fraction RNA cleaved (±S.D.) of three independent trials (see Figure 5—source data 1). (E) Deoxyribonuclease activities of RNases J1 and J2 on linear single-stranded DNA. The 5’-end labelled 60-nucleotide DNA substrate was combined with 0.5 pmols of indicated enzyme(s). The reaction mixture was incubated at 37°C for increasing time points (0, 2, 5, or 10 min.) DNAs were resolved using denaturing urea-PAGE. (F) Quantification of deoxyribonuclease activity on single-stranded DNA. The average fraction DNA cleaved (±S.D.) from three independent trials is shown (see Figure 5—source data 2). (G) Deoxyribonuclease activities of RNases J1 and J2 on double-stranded DNA substrates. The indicated enzymes (two pmols) were combined with 0.1 μg pUC19 DNA that was supercoiled or linearized by PstI digestion. The reaction mixture was incubated at 37°C for 1 hr and DNA was resolved on an agarose gel. (H) Deoxyribonuclease activities of RNases J1 and J2 on single-stranded circular DNA. The indicated enzyme(s) (four pmols) were combined with 0.5 μg of phage M13MP18 DNA and the reaction mixture was incubated at 37°C for increasing time points (15, 30, 60 min.) The DNA was resolved on a 0.7% agarose gel. Images in panels G and H are representatives of four independent trials.
-
Figure 5—source data 1
Accompanies Figure 5D.
Fractions of RNA cleaved by indicated enzymes at various time points.
- https://doi.org/10.7554/eLife.45393.019
-
Figure 5—source data 2
Accompanies Figure 5F.
Fractions of DNA cleaved by indicated enzymes at various time points.
- https://doi.org/10.7554/eLife.45393.020
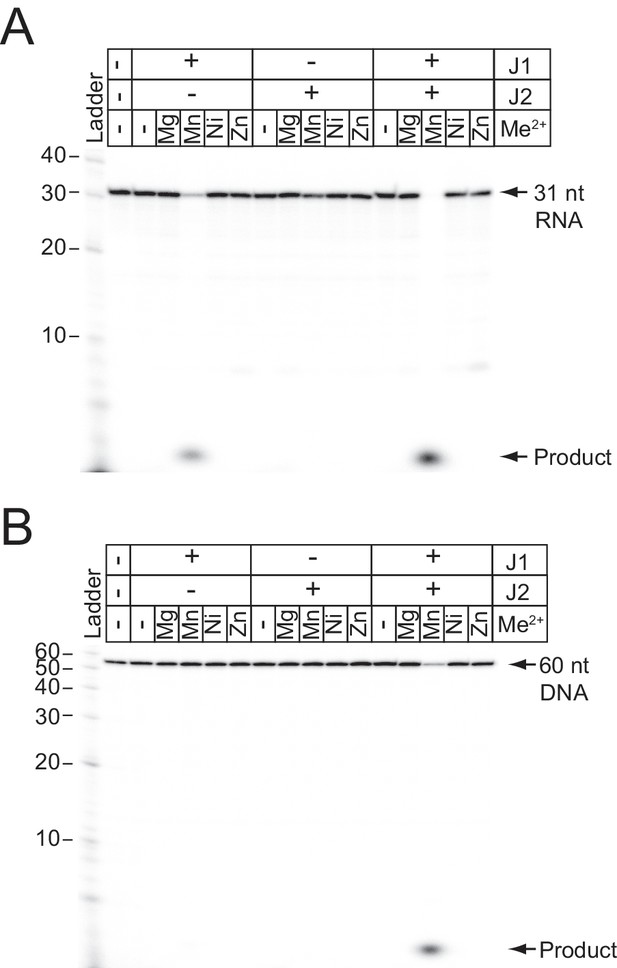
Metal-dependent cleavage of RNA and DNA by RNases J1 and J2.
(A) RNA cleavage by Ribonucleases J1 and/or J2. A 5’-end labelled 31-nucleotide RNA substrate (shown in Figure 5B) was combined with 0.5 pmols of each enzyme and 10 mM of EDTA or indicated divalent metal ions for 20 min at 37°C. (B) DNA cleavage by Ribonucleases J1 and/or J2. A 5’-end labelled 60-nucleotide DNA substrate (shown in Figure 5B) was combined with 0.5 pmols of each enzyme in 10 mM of EDTA or indicated divalent metal ions for 10 min at 37°C. Nucleic acids were resolved using denaturing urea-PAGE.
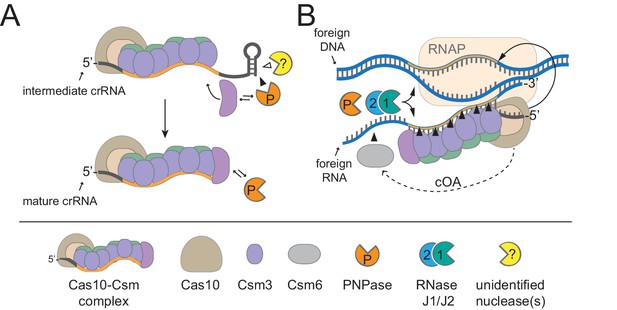
A model for CRISPR-Cas10 immunity in which multiple steps in the pathway rely upon degradosome nucleases.
(A) During complex assembly, Csm5 recruits and stimulates PNPase, which trims the 3’-ends of intermediate crRNAs. The combined activities of PNPase and other unidentified nuclease(s) are required for crRNA maturation. (B) During interference, PNPase and the RNase J1/J2 complex help to degrade invading nucleic acids alongside the Cas nucleases. RNAP, RNA Polymerase; cOA, cyclic oligoadenylates.
Tables
Reagent type (species) or resource | Designation | Source or reference | Identifiers | Additional information |
---|---|---|---|---|
Gene (Stahylococcus epidermidis) | pnp | N/A | GenBank: CP000029.1_SERP0841 | encodes PNPase |
Gene (S. epidermidis) | rnjB | N/A | GenBank: CP000029.1_SERP0842 | encodes RNaseJ2 |
Gene (S. epidermidis) | rnjA | N/A | GenBank: CP000029.1_SERP0676 | encodes RNase J1 |
Strain, strain background (S. epidermidis) | RP62a | PMID: 3679536 | GenBank: CP000029.1 | LA Marraffini (Rockefeller University) |
Strain, strain background (S. aureus) | RN4220 | PMID: 21378186 | GenBank: NZ_AFGU00000000 | LA Marraffini (Rockefeller University) |
Strain, strain background (phage CNPx) | CNPx | PMID: 26755632 | GenBank: NC_031241 | LA Marraffini (Rockefeller University) |
Strain, strain background (phage Andhra) | Andhra | PMID: 28357414 | GenBank: KY442063 | isolated in-house |
Genetic reagent (S. epidermidis) | LM1680 | PMID: 24086164 | LA Marraffini (Rockefeller University), derivative of RP62a with large deletion | |
Genetic reagent (S. epidermidis) | RP62a Δpnp | This paper | the central 2016 nucleotides of the pnp coding region are deleted, Figure 2—figure supplement 1 | |
Genetic reagent (S. epidermidis) | LM1680 Δpnp | This paper | the central 2016 nucleotides of the pnp coding region are deleted,Figure 2—figure supplement 1 | |
Genetic reagent (S. epidermidis) | LM1680 Δpnp::pnp* | This paper | a copy of pnp with five silent mutations re-introduced into the pnp locus, see Figure 2—figure supplement 2 | |
Genetic reagent (S. epidermidis) | RP62a ΔrnjB | This paper | the central 1647 nucleotides of the rnjB coding region are deleted, Figure 2—figure supplement 1 | |
Genetic reagent (S. epidermidis) | LM1680 ΔrnjB | This paper | the central 1647 nucleotides of thernjB coding region are deleted, Figure 2—figure supplement 1 | |
Genetic reagent (S. epidermidis) | RP62a ΔpnpΔrnjB | This paper | contains in-frame deletions of pnp and rnjB as described in the cells above | |
Genetic reagent (S. epidermidis) | LM1680 ΔpnpΔrnjB | This paper | contains in-frame deletions ofpnp and rnjB as described in the cells above | |
Recombinant DNA reagent | pKOR1 | PMID: 16051359 | LA Marraffini (Rockefeller University) | |
Recombinant DNA reagent | pKOR1_Δpnp | This paper | to create in-frame deletion of pnp via allelic replacement, see Supplementary file 1 | |
Recombinant DNA reagent | pKOR1_pnp* | This paper | to re-insert a copy of pnp containing five silent mutations into the pnp locus via allelic replacement, see Supplementary file 1 | |
Recombinant DNA reagent | pKOR1_ΔrnjB | This paper | to create in-frame deletion of rnjB via allelic replacement, Supplementary file 1 | |
Recombinant DNA reagent | pKOR1_ΔpnpΔrnjB | This paper | to create in-frame deletions of pnp and rnjB via allelic replacement, see Supplementary file 1 | |
Recombinant DNA reagent | pcrispr-cas | PMID: 23935102 | LA Marraffini (Rockefeller University) | |
Recombinant DNA reagent | pcrispr-cas-rnjB | This paper | contains the coding region of rnjB inserted downstream of cas6, see Supplementary file 1 | |
Recombinant DNA reagent | pcrispr-spcA2 | PMID: 28885820 | created in-house | |
Recombinant DNA reagent | pET28b-His10Smt3 | PMID: 15263846 | LA Marraffini (Rockefeller University) | |
Recombinant DNA reagent | pET28b-His10 Smt3-rnjA | This paper | for RNase J1 overexpression and purification, see Supplementary file 1 | |
Recombinant DNA reagent | pET28b-His10Smt3-rnjB | This paper | for RNase J2 overexpression and purification, see Supplementary file 1 | |
Recombinant DNA reagent | pET28b-His10Smt3-drnjA | This paper | for RNase J1/H74A H76A overexpression and purification, see Supplementary file 1 | |
Recombinant DNA reagent | pUC19 | New England Biolabs | Cat. # N3041S | |
Recombinant DNA reagent | M13MP18 | New England Biolabs | Cat. # N4040S | |
Sequence-based reagent | DNA oligonucleotides (multiple) | Eurofins MWG Operon | To build and sequence recombinant DNA constructs, and to perform quantitative PCR, see Supplementary file 1 | |
Peptide, recombinant protein | T4 Polynucleotide kinase | New England Biolabs | Cat. # M0201L | |
Peptide, recombinant protein | Lysostaphin | AmbiProducts via Fisher | Cat. # NC0318863 | |
Peptide, recombinant protein | DNase I | New England Biolabs | Cat. # M0303S | |
Peptide, recombinant protein | M-MuLV reverse transcriptase | New England Biolabs | Cat. # M0253L | |
Peptide, recombinant protein | DpnI | New England Biolabs | Cat. # R0176S | |
Peptide, recombinant protein | SUMO Protease | MCLAB, http://www.mclab.com/SUMO-Protease.html | Cat. # SP-100 | |
Commercial assay or kit | EZNA Cycle Pure Kit | Omega Bio-tek via VWR | Cat. # 101318–892 | |
Commercial assay or kit | EZNA Plasmid DNA Mini Kit | Omega Bio-tek via VWR | Cat. # 101318–898 | |
Commercial assay or kit | Wizard Genomic DNA Purification Kit | Promega Corporation via VWR | Cat. # A1120 | |
Commercial assay or kit | PerfeCTa SYBR Green SuperMix | Quanta Biosciences via VWR | Cat. # 101414–150 | |
Chemical compound, drug | TRIzol Reagent | Thermo Fisher | Cat. #15596026 | |
Chemical compound, drug | TRI REAGENT RT | Fisher Scientific | Cat. # NC0850610 | |
Chemical compound, drug | BAN (4-bromoanisole) | Fisher Scientific | Cat. # NC9734505 | |
Chemical compound, drug | g-32P-ATP | Perkin Elmer | Cat. # BLU502H250UC | |
Chemical compound, drug | HisPur Ni-NTA Resin | Thermo Fisher | Cat. # 88222 | |
Software, algorithm | ImageQuant TL | GE Healthcare/Life Sciences | RRID: SCR_014246 | Version 8.1, used for densitometry |
Software, algorithm | CFX Manager | BioRad | Cat. # 1845000 | Version 3.1, used for qPCR analysis |
Additional files
-
Supplementary file 1
DNA oligonucleotides used in this study.
Already corrected in first full submission. Added after initial submission
- https://doi.org/10.7554/eLife.45393.022
-
Transparent reporting form
- https://doi.org/10.7554/eLife.45393.023